DALE SCALISE-SMITH, PT, PhD and DARCY A. UMPHRED, PT, PhD, FAPTA After reading this chapter, the student or therapist will be able to: 1. Comprehend the complexity and interlocking nature of human development over a lifetime. 2. Differentiate traditional theories of development from contemporary theories. 3. Analyze the differences among various subsystems within the human organism. 4. Identify elements of physiological changes over a lifetime. 5. Analyze normal movement strategies and identify subsystems responsibility for success of a motor task. 6. Identify normal changes in motor strategies over a lifetime and synthesize differences between normal movement patterns and pathological movement problems across the life cycle. Throughout much of the twentieth century, developmental researchers were heavily focused on skill acquisition from infancy through early childhood.1–3 In the 1970s, as research paradigms directed at motor development and motor learning evolved, it became evident that changes in motor skills were not limited to childhood but occurred throughout the life span. Consequently, the concept of life span development came to incorporate the prenatal period through older adulthood. During infancy (birth to 1 year) and childhood (1 year to 10 years) acquisition of motor skills coupled with cognitive and perceptual development are the primary foci of developmental researchers and clinicians. As the young child transitions to adolescence (11 to 19 years) and has the opportunity to experience motor behaviors across different environmental contexts, more complex behaviors emerge. Adulthood (20 to 59 years) signals a period when skills are refined and motor behaviors mature. Only through practice and repetition are skills attained and retained. Individuals who continue to use motor learning strategies into late adulthood (60 years through death) often report more successful aging than those who do not engage in such motor skills.4 Identifying mechanisms that enable individuals to be successful in acquisition and retention of functional motor behaviors is critical to examining variables that alter or impair these same behaviors in other individuals. Kandel and colleagues5 suggested that “the task of neural science is to understand the mental processes by which we perceive, act, learn, and remember” (p. 3). This view supports the interactive and collaborative nature of intrinsic and extrinsic systems to accomplish motor learning tasks. Development is often portrayed as a series of stages through which an infant progresses, with a fixed order to the sequence.6 A developmental theory may be characterized as a systematic statement of principles and generalizations that provides a coherent framework for studying development. Historically, development was thought to be linear, occurring in an invariant sequence and resulting in behavioral changes that are direct reflections of the maturation of anatomical and physiological systems.7,8 Development is generally examined in terms of quantitative and qualitative change. Although it is universally accepted that acquisition of developmental skills is not reversible, the underlying principles surrounding the emergence of these behaviors has evolved over the past 50 to 75 years. Early developmental theorists used neuromaturational models of CNS organization as the framework for conceptualizing development.1,2 These researchers provided elaborate descriptions of posture acquisition and a blueprint delineating skill development. Research focused on the emergence of cognitive and affective behaviors and ignored the processes and mechanisms involved in acquiring motor skills.9 Several investigators attributed developmental changes to intrinsic variables such as maturation of the CNS, whereas others associated changes with extrinsic variables involving the environment.1,2,10,11 During the 1930s and 1940s, Arnold Gesell and Myrtle McGraw led a cadre of avant-garde researchers exploring the field of infant motor development. Gesell1,12 described the normative time frame for when behaviors emerge, and McGraw2 examined the underlying mechanisms responsible for the emergence of these behaviors. The underlying premise, the foundation for their elaborate descriptions of motor development, was based on maturational processes in the CNS. Gesell, a pioneer in developmental research, was a proponent of the theory that nature drives development.13 He proposed that growth is a process so complicated and so sensitive that intrinsic factors are solely responsible for influencing development. He used the evolutional thinking of Darwin and Coghill to explain changes in motor behaviors. Coghill,14 in his work with salamander embryos, reported that motor behaviors, like swimming, emerge in an orderly sequence as connections of specific neural structures appear. Coghill concluded from his observations of emergence of behaviors in the salamanders that human infant motor behaviors appear in a predictable sequence and at predictable chronological ages. With Coghill’s research as the foundation for his thinking, Gesell embraced the concept of a hierarchical organization of the CNS. He believed that the emergence of motor behaviors was contingent on maturation in the CNS and concluded that only after the emergence of higher-level neural structures would complex motor behaviors appear. Within this constrained theoretical perspective, extrinsic or environmental stimuli, human or otherwise, were thought to have little impact on the appearance of motor behaviors. Gesell concluded that infant development is preprogrammed and linear, emerging at predetermined stages or periods in time.13 Perhaps his greatest contribution to motor development was the conceptualization of milestones as markers to evaluate infant behavior. Although McGraw was a proponent of ontogenic development as one variable influencing motor development, she did not believe, as Gesell did, that it was the sole determinant.15 Rather, McGraw attempted to explain the emergence of motor behaviors through environmental influences as well as CNS maturation.2 She examined the temporal and qualitative aspects of motor skill acquisition through her study of Jimmy and Johnny,16 a study of twin brothers in which one twin was provided an exercise program, whereas no intervention was afforded the other twin. She found temporal and qualitative differences in the boys’ acquisition of motor skills and attributed differences in acquisition of these behaviors to disparities in practice opportunities. Sufficient evidence exists to support the premise that although some predetermined processes occur at relatively similar points in development, not all motor behaviors emerge at the same biological, chronological, or psychological age in every individual. Although motor milestones provide information regarding outcome, no information can be derived about the process of attaining motor skills from those specific milestones. Perhaps a more realistic explanation may be that emergence of new skills occurs out of a need to solve specific problems within the environment. Working within this context, it is evident that traditional theories of development and maturation fail to adequately encapsulate the innate variability in human development.9,10 Within the last few decades, researchers have used more current theories of development when designing studies involving infants and young children.17–19 These investigators examined the process of skill acquisition rather than using traditional methods that assess outcome as a measure of motor development.20 Although early pioneers in developmental research described development as linear, uniform, and sequential, Thelan and Smith21 depict development as “messy,” “fluid,” context-sensitive, and nonlinear. Linear and nonlinear dynamics are derived from mathematics. Linear dynamics is described by the proportional relationship of the initial condition to the outcome, whereas no such proportional relationship exists in nonlinear dynamics. Nonlinearity is used to describe complex systems, in this case biological or more specifically human systems.22 Within these complex systems exists a level of unpredictability, given the interactive and interdependent nature of biological systems. Thelan and Smith21 suggested that, although traditional theories of development support the premise that behaviors emerge in accordance with a relatively fixed temporal sequence, an organism may exhibit “precocial” abilities when the context is altered and the behavior emerges earlier than expected. These authors stated that immature systems exhibit behaviors that are variable and easily disrupted. Although development of some organisms in a controlled laboratory environment may reflect more traditional perceptions of development, outside, in a more naturalistic environment, development is more likely to be flexible, fluid, and tentative. Thelan and Smith also found that factors most likely to have an impact on performance are the “immediacy of the situation” and the “task at hand” rather than “rules” of the performance. Given this perspective, Thelan and Smith21 identified six goals as essential to developmental theory. These goals are as follows: 1. To understand the origins of novelty. 2. To reconcile global regularities with local variability, complexity, and context-specificity. 3. To integrate developmental data at many levels of explanation. 4. To improve a biologically plausible yet non-reductionist account of the development of behavior. 5. To understand how local processes lead to global outcomes. 6. To establish a theoretical basis for generating and interpreting empirical research.21 (p. xviii) Thelan and Smith urged developmental researchers to devise paradigms that attempt to explain development in terms of diversity, flexibility, asynchrony, and “the ability of even young organisms to reorganize their behavior around context and task” (p. 18).21 Contemporary theorists inferred that developmental changes are nonlinear and emergent and may be the result of the interactive effects of intrinsic and extrinsic variables. This divergence from traditional thinking compelled avant-garde scholars to propose new theories.21,23,24 Investigators described behaviors as complex, interactive, cooperative, and reflects an ability to organize and regroup around task and context, rather than conforming to a rigid structure and rule-driven hierarchy, as many earlier cognitive researchers believed.21,24 Contemporary theorists exploit technology to derive a model to explain variability and flexibility in developing, mature, and aging populations.24 Systems theory, first described by von Bertalanffy in 1936, was not discussed in great detail until 1948. In 1954 von Bertalanffy and colleagues from three other professions met to discuss systems movement.25 Theorists then applied systems theory to a variety of human and nonhuman systems. As theorists became acquainted with systems theory, they became more receptive to alternate theoretical proposals of growth and development in living organisms. Systems theory may be applied as a transdisciplinary model examining relationships of structures as a whole.26 “The notion of a system may be seen as simply a more self-conscious and generic term for the dynamic interrelatedness of components”26; von Bertalanffy proposed this theory to more adequately describe biological systems, investigate principles common to all complex organisms, and develop models that can be used to describe them.26 Principles that embody general systems theory include nonsummative wholeness, self-regulation, equifinality, and self-organization.26 Contrary to systems theory in disciplines such as traditional physics, in which systems are said to be closed, von Bertalanffy suggested that biological systems are open and modifiable and that changes in the system are the result of the dynamic interplay among elements of the system.26 Embedded in the general systems theory is nonlinear dynamics, a concept in which behaviors are not described as the sum of their parts. Thus within a nonlinear model, a mathematical model is derived.24,27 Characterized within this model is the notion that systems may change in a sudden, discontinuous fashion. During development a small increase or decrease in one parameter leads to changes in the behavior. This abrupt change, identified as a bifurcation, causes the system to move out of its previous state and toward a new state of being. Throughout development, periods of rapid differentiation or change occur when an organism is most easily altered or modified. These periods were identified by Scott28 as “critical periods.” Physiological systems are most vulnerable during these periods and may be seriously affected by both intrinsic and extrinsic factors acting on the system. These periods occur at different times for different body systems. Understanding systems theory and the concept of critical periods is crucial to all aspects of motor development. As scientists began to revisit theories of motor development, they discarded some of the traditional theories and embraced contemporary concepts of nonlinear dynamics.24,25,27 Proponents of nonlinear dynamics contend that modifications in motor behaviors are the result of dynamic interactions among the musculoskeletal, peripheral and central neuromuscular, cardiovascular and pulmonary, and cognitive and emotional systems.26 These interactive, multidimensional elements are vulnerable to changes in organizational and behavioral abilities (system) over time.28,29 Some theorists propose that as skills are acquired and organizational or behavioral changes occur, the system is driven to identify the most efficient and effective strategy to produce motor behavior(s).28,29 Yet others purport that variability implies that typical healthy individuals may use a variety of strategies to produce the same behavioral outcome and that variability is an indication of the individuals’ flexibility in responding to unpredictable perturbations.24,30 Implicit in nonlinear dynamics is the concept of critical periods in development.28,29,31 Investigators suggested that interventions imposed during a critical period may more easily positively or negatively modify the behavior. Recognizing the crucial role systems theory and critical periods play in development is vital to comprehending how developmental skills emerge. The multifactorial nature of nonlinear dynamics illustrates the complexity of development and the difficulty in identifying the appropriate variables that influence motor skill development. With use of concepts previously described, it is reasonable to expect that a small change in any subsystem may result in a large change in a motor behavior. This is evident in work by Thelan and colleagues examining stepping in infants 8 weeks of age.29,31–34 They reported that introducing a small change in one element of the system, identified as a small weight applied to an infant’s leg, resulted in the infant being unable to step. The authors deduced that small changes in one subsystem, in this case the musculoskeletal system, may result in a change in the outcome. This lends support to the hypothesis that modifying one aspect of a multicomponent system, especially during a critical period, may cause the system to evolve into an entirely new behavior. Menopause may be another critical period. During menopause, decreases in hormone production are thought to lead to osteoporosis and cardiac disease.35 Examples described previously provide evidence across the life span that the dynamic interplay within a system and among systems may significantly influence emergence and disappearance of behaviors. Although research in the beginning of the twentieth century was heavily focused on development of the very young, studies during the latter part of the century were directed toward research on aging. Technological advances in medicine have dramatically increased life expectancies. During the twentieth century, the number of individuals in the United States older than 65 years old grew from 3 million to 35 million.36 Perhaps the most significant statistic is that the oldest old grew from 100,000 in 1900 to 4.2 million in 2000.36 By 2011 the Baby Boomer generation will begin turning 65 years old, and the number of older individuals will increase sharply between 2010 and 2030.36 By 2030, Americans over the age of 65 years will represent nearly 20% of the population, and by 2050 the number of individuals over the age of 85 years could grow to 21 million.36 Given this incredible demographic transformation and that current policymakers are, in large part, the generation directly affected by these statistics, a significant paradigm shift in funded research has evolved over the past quarter century. “As such, aging and death are inseparable partners to growth and development”37 (p. 32). Recognizing that a critical mass of Americans are entering older adulthood, terminology that operationally defines and is then applied consistently when referring to the aging population or an individual is imperative. Age can be described in terms of chronological age and biological age.38 Chronological age is the period of time that a person has been alive, beginning at birth. In infants it is measured in days, weeks, or months, whereas in adults it is expressed in terms of years and at times decades. Although chronological age is measured in terms of temporal sequencing, biological age is related more to functioning and physiological aging of organ systems.39 For example, a triathlete may have biologically younger cardiovascular and pulmonary systems than same-age peers who do not perform high-level aerobic activities. Another example might be a child who underwent precocious puberty. Precocious puberty, identified as puberty earlier than 8 years of age in girls and 9.5 years in boys, results in acceleration in a biological system before same-chronological-age peers.40 Physiological changes include elevated hormonal levels, which would then stimulate development of breast tissue and early menstruation in girls. Changes in the musculoskeletal system include early closure of the epiphyseal plates, resulting in significantly smaller stature. Conversely, these young women’s reproductive cycles are significantly skewed. Women would also have menopause and aging issues associated with hormonal changes earlier than other women of the same chronological age. Although no consistent method has been established for measuring biological age, there is general agreement that a wide variability of biological aging exists and that a number of factors contribute to accelerated or decelerated biological aging. “Aging refers to the time-sequential deterioration that occurs in most animals including weakness, increased susceptibility to disease and adverse environmental conditions, loss of mobility and agility, and age-related physiological changes” (p. 9).41 Although Goldsmith’s description of aging is typically viewed as an inevitable fact of life, there is scientific evidence and theoretical support for the idea that age-related changes will eventually be more medically treatable than previously thought.38,41,42 Scientists are hesitant to attribute a decline in functional movement in older adults to a decline in physiological systems or to diminished opportunities for practice or conditioning.4 Rowe and Kahn reported that “with advancing age the relative contribution of genetic factors decreases and [of] the nongenetic factors increases” (p. 446).4 Age-related factors that are modifiable may be used to identify individuals who may or may not age successfully. For instance, lifestyle choices, including diet, physical activity, and other health habits, and behavioral and social factors have a potent effect and accelerate or decelerate aging. Evidence to support this was initially derived from a 10-year study conducted by Rowe and Kahn.43 The authors identified three critical factors that contribute to aging successfully: avoidance and absence of disease, maintaining cognitive and physical functioning, and “sustained engagement in life.”43 Recently researchers suggested that Rowe and Kahn’s classification of successful aging is too restrictive and may lead to classifying individuals with relatively minor health problems as unhealthy. McLaughlin and colleagues44 suggested that a critical variable in defining successful aging is first identifying what the goal is for measuring successful aging. Only then can researchers determine how best to define and measure successful aging. Although controversy exists regarding defining successful aging, factors that contribute to successful aging hinge on higher levels of physical activity, increased social interactions, and positive perception of health, as well as no smoking, chronic diseases (arthritis, diabetes), or impaired cognition.45,46 Consequently, developing healthy behaviors early in life may be critical to maintaining good health and may play a significant role in successful aging. Factors associated with aging are generally identified as either age related or age dependent. Age-dependent changes within organ systems are observed in individuals at a similar age, whereas age-related changes may be accelerated or decelerated in same-age individuals on the basis of intrinsic or extrinsic factors related to lifestyle. Just as variables associated with lifestyle (extrinsic factors) influence aging, genetics (intrinsic factors) also play a significant role. From a genetic perspective, structural and functional changes are generally thought to be a consequence of aging and are therefore predictable and consistent across physiological systems. Variables thought to influence the genetic potential for longevity include environmental factors such as toxins, radiation, and oxygen free radicals. Free radicals are highly reactive molecules produced as cells turn food and oxygen into energy.47 In summary, the use of biological age rather than chronological age may be a more accurate reflection of an individual’s true age. Scientists view aging as a progressive accumulation of changes over time that increases the probability of disease and death.48 Given that portrayal of aging, researchers have proposed myriad hypotheses regarding aging. Aging theories evolved because there is no single factor or mechanism responsible for physiological aging.38 Biological aging theories, similar to developmental theories, are attributed to complex, underlying mechanisms.38,41,42,49 Although theorists attempt to classify aging theories, these theories are rarely mutually exclusive. Some theories were formulated around control of physiological functioning, others around cellular changes, and still others around genetic causes. Neuroendocrine theory is based on the premise that hormones play a significant role in aging.42,49 Hormones are vital to repairing and regulating bodily functions. Hormone production decreases significantly during aging and limits the body’s ability to repair and regulate itself as effectively. Although hormonal decline is one plausible explanation for age-related changes, it does not account for all changes. Harman50 proposed the free radical theory on the basis of his investigations that examined the effects of radioactive materials on human tissue.50 Harman reported that when human tissue is exposed to radiation, a byproduct is formed. He identified the byproduct, an unstable compound, as a free radical. Over time, human tissue with free radicals showed evidence of biological defects consistent with accelerated aging. Harman postulated that accumulation of free radicals in human tissue may also occur as a part of the normal aging process. This became known as the free radical theory of aging.50–52 Free radicals are highly reactive molecules that damage proteins, lipids, and deoxyribonucleic acid (DNA). In some instances the free radicals combine with enzymes and turn into water and a harmless form of oxygen that moves harmlessly through the cells.51 In other instances the oxygen binds with intrinsic or extrinsic sources that influence the aging process. Scientists have suggested several different ways that free radicals influence aging through intrinsic and extrinsic mechanisms.51,52 An example of an intrinsic mechanism would be chronic infections that extend phagocytic activity and expose tissues to oxidants, creating cumulative oxidative changes in collagen and elastin. Extrinsic sources of free radicals include environmental toxins—for example, industrial waste and cigarette smoke. Human exposure to intrinsic and extrinsic free radicals causes large numbers of reactive oxygen molecules to interact with DNA, leading to mutations thought to be the cause of a variety of diseases, including cancer, atherosclerosis, amyloidosis, age-related immune deficiency, senile dementia, and hypertension. Although some scientists suggest that aging has many factors that can accelerate or decelerate the process, other scientists suggest a much simpler, preprogrammed theory, known as the Hayflick limit.38,51–53 Hayflick and Moorhead53,54 proposed that there is a finite number of times that a normal cell is capable of dividing. Current thinking is that cells are capable of dividing up to 50 times. Cell division is recognized as one way in which cells age and, after attaining the maximum number of divisions, finally die. The factor thought to limit a cell’s ability to divide infinitely is the presence of telomeres. Telomeres are minute units at the end of the DNA chain.53 Each time a cell divides a small amount of the telomere is used in the process. Eventually, when cells have exhausted the supply of telomeres available, the cell is unable to divide and cell death ensues. Telomerase, a substance that can lengthen telomeres, is available in human cells. Typically, telomerase is switched off in all cells except the reproductive cells. The availability of telomerase in reproductive cells allows for many more divisions than previously observed in the Hayflick limit. In addition to the presence of telomerase in reproductive cells, scientists have also discovered that telomerase remains active in cancer cells. Both reproductive and cancer cells divide well beyond the 50-division limit. Consequently, scientists are now working toward activating telomerase in all cells to slow or stop aging. If scientists are successful in activating telomerase in other cells, it may stimulate skin cell regrowth for burn patients and cure diseases that result from failure of aging cells to divide, as in macular degeneration or Hutchinson-Guilford progeria syndrome.55,56 The downside of this is that scientists may have a difficult time controlling the telomerase and in fact may see more uncontrolled cell growth—cancer—one of the greatest threats to prolonged existence. Although many aging theories are directed at mechanisms that negatively influence aging, other theories are focused on factors that have a positive impact on aging processes. One such process is the caloric restriction theory.50,52,53,57 Liang and colleagues,57 with use of several genetic mouse models, investigated the impact of dietary control on the life span. The authors reported that the mice did, in fact, have their life spans extended when their dietary intake was controlled. Although these findings are potentially significant, given the small sample size and model examined, these data were not generalizable to all species. The researchers suggested that these preliminary data provide a foundation for scientists to examine whether dietary control will extend the life span in humans as it did in the mouse models. Although a large body of literature exists examining the underlying mechanisms associated with aging, it seems inconceivable that any one mechanism is responsible for age-related changes. More likely is that aging may be attributed to multiple factors, including lifestyle choices, in combination with the physiological and environmental factors.58 Garilov and Gavrilova58 conducted an exhaustive review of aging theories and concluded that additional research is necessary to further elaborate and validate existing aging theories and dispel unlikely theories. In summary, scientists are unsure how much of the decline in motor behaviors in older adults is attributable to true decline in physiological systems, how much is attributable to expected decline, how much to a decreased ability to perform skilled behaviors under variable conditions, and how much to decreased practice or conditioning.59–62 This suggests that physical or occupational therapy intervention may provide older adults with strategies to positively influence successful aging rather than being applied only after a negative outcome of aging is realized or a neurological insult has occurred. Skeletal muscle tissue first appears during the fifth week of embryonic development and continues to develop into adulthood.53,63 During this early period of embryonic development, the differentiation of musculoskeletal system is rapid: during the fifth week of embryonic life the limb buds appear, by the seventh week muscle tissue is present in the limbs, and limb movements emerge as early as the eighth week of prenatal life.40,64,65 Whereas many of the structural aspects of the musculoskeletal system are formed prenatally, muscle and bone continue to grow into adulthood. Motor skill acquisition involves considerable variability among young children from age 5 months through 3 years. During this time, the rate of growth of muscle tissue is reportedly two times faster than that of bone.66 Distinct differences also exist in temporal differentiation of the muscular systems of males and females of the same chronological age. Through adolescence, boys show evidence of a significantly greater increase in fiber size compared with girls.67 In addition, differences exist in the age at which the number of muscle fibers dramatically increases. Girls reportedly have a steady increase in the muscle fibers from 3.5 to 10 years of age. In contrast, boys have two periods of rapid differentiation in the number of muscle fibers. The first period occurs from birth until 2 years of age and the second from ages 10 to 16 years.67 Although the pace slows considerably, muscle fiber development continues in men and women well into middle adulthood. Age-related changes evident in the musculoskeletal system include decreased fiber size, loss of muscle mass, denervation of muscle fibers, decline of total muscle fiber number, and decreased quantity of fast-twitch fibers.68–70 Muscle mass decreases beginning at around age 50 years, and by age 80 years up to 40% of muscle mass has been lost.71 Muscle force production likewise decreases at a rate of about 30% between 60 and 90 years of age. Additional musculoskeletal changes documented in older adults include decreased tensile strength in bone, reduced joint flexibility, and limited speed of movement. Decreased muscle mass in a person older than 60 years may be attributed to decreased size, fewer type II muscle fibers, and an increase in fat infiltration into the muscle tissue.72,73 Clinically these factors manifest as reduced muscle force production during high-velocity movements. Currently, scientists are examining the premise that, as an individual ages, muscular changes are more likely attributable to decreased motor activity levels and are age related rather than being solely age dependent.69,72 Acknowledging that investigators had previously found that muscle power deteriorates more quickly with age, scientists set out to measure training effects in older adults.61,74 These investigators concluded that with training older adults were capable of improving strength, power, and endurance. The skeletal system, similar to the muscular system, experiences periods of growth, stability, and degeneration. The immature skeletal system is composed primarily of preosseous cartilage and physes (growth plates).75 More simply, bone in infants and young children is flexible, porous (lower mineral count), and strong with a thick periosteum.75,76 Given these properties of immature bone, a child is less likely to have a fracture because the periosteum is strong and consequently the bones absorb more energy before the break point is reached. In addition, if a fracture does occur, healing is usually quicker because callus is formed faster and in greater amounts in children than in adults. A primary difference between the child’s and the adult’s skeletal system is the presence of the growth plate complex in children. Whereas primary ossification occurs prenatally, secondary ossification is not complete until the child reaches skeletal maturity, generally at age 14 years in girls and 16 years in boys.76,77 Even after bones have attained their full length, they continue to grow on the surface. This is termed appositional growth and continues throughout most of life. During childhood and adolescence, new bone growth exceeds bone resorption and bone density increases. Until age 30 years, bone density increases in most individuals, and bone growth and reabsorption remain stable through middle adulthood. Later in adulthood, resorption exceeds new bone growth and bone density declines.78 Women exhibit more loss of bone mass than men do.79 Decreased bone density in women is generally attributed to differences in the types and levels of hormones present. Although the difference is most significant during menopause, premenopausal women still lose bone density at a higher rate than their male peers do. Osteopenia is the presence of a less-than-normal amount of bone and, if not treated, may result in osteoporosis. Progressive loss of bone density, observed into older adulthood, is commonly identified as osteoporosis. Osteoporosis is more common in women than in men and is a major cause of fractures and postural changes in both sexes.80 Overall, much of the growth in the musculoskeletal system is related to demands placed on the system. Intrinsic and extrinsic forces imposed on the musculoskeletal systems of typically and atypically developing children may lead to structural and functional differences in their respective skeletal structures. Consequently, temporal sequencing, acquisition, and characteristics of motor behaviors emerge differently in typically and atypically developing children. Similarly, age-related changes in older adulthood may be accelerated in direct proportion to decreased levels of activity.81 Older adults who maintain more active lifestyles and place greater physical demands on their musculoskeletal systems are more likely to have an improved bone density and muscle mass than their peers who are not as active.82 Sarcopenia, the age-related loss of muscle mass, affects strength, power, and functional independence in older adults.72,82 Although these changes are observed in many older adults, the degree of the muscular changes varies.70 Researchers examining sarcopenia in older adults reported that men are affected more by sarcopenia than women are.72,83 In fact, men with sarcopenia manifest four times the rate of activity limitations than do men with a normal muscle mass. Changes in the cross-sectional area of muscles directly affect the force production of a given muscle; consequently, as the cross-section of a muscle diminishes, its ability to produce force decreases. As an individual ages the number and size of the muscle fibers decrease, resulting in a reduction in strength.80 Although this is true in all muscles, the impact is greater on muscles of the lower extremities than in those of the upper extremities.72 Although strength is critical to musculoskeletal function, flexibility is equally as important. Flexibility incorporates joint motion and the extensibility of the tissues that cross the joint. The degree of flexibility changes across the life span as a direct result of aging and activity level.10 Changes in flexibility are evident throughout life: limited at birth, increasing until the individual approaches adolescence, and then gradually decreasing. Exceptions may be seen in athletes, dancers, and other individuals involved in activities that incorporate flexibility training. Loss of flexibility as a consequence of age may have a negative impact on functional independence in older adults. Flexibility is thought to be directly proportional to the amount, frequency, and variability of motor activities performed. As activity increases, so does flexibility. Conversely, as individuals exhibit decreased levels of motor activity, often associated with age, flexibility decreases.78 By age 70 years, flexibility is thought to have decreased by 25% to 30%.70 Although this was purported to be age dependent, it may be more likely that it is age related.69 Regularly performing exercise directed toward improving strength and flexibility can reverse the effects of inactivity for most individuals, even those older than 90 years of age.69,81 As scientists continue to examine functional changes across different systems as a consequence of age, physical and occupational therapists must educate individuals regarding the importance of embracing a physically active lifestyle and methods to enhance quality of life at each stage in an individual’s life (see Chapter 2). Although all systems contribute to an individual’s health and wellness across the life span, the cardiovascular and pulmonary systems play a key role (see Chapter 30). The cardiovascular system is composed of the heart, lungs, and associated vascular complex. It is responsible for pumping blood through the coronary, pulmonary, cerebral, and systemic circulations, with the goal of perfusing all bodily tissues for the delivery of oxygen and vital nutrients and picking up waste products for elimination. The pulmonary system is responsible for oxygen transport, gas exchange, and removal of airborne pollutants that may enter during respiration (see Chapter 30). The interdependent nature of the cardiovascular and pulmonary systems is evident in the fact that, each minute, all of the body’s blood travels through the lungs before being returned to the left side of the heart for ejection into the systemic circulation.84 Because of this relationship, changes in heart function can dramatically affect lung function, and vice versa. In addition, these two systems are connected as part of a larger closed pressure-volume loop through the peripheral circulatory structures. Likewise, any alteration in the function of the peripheral vessels will affect both the heart and lungs, and vice versa. The function and homeostasis of the cardiovascular, pulmonary, and peripheral vascular systems are influenced by both internal and external forces.84 Internal mechanisms of control are based on the autonomic nervous system, the relative health of the anatomic structures involved, the growth and development of the structures, and the behavioral and emotional adaptations of a particular individual. All those internal mechanisms are subject to changes with growth and development, aging, and the unique life experiences of an individual. Growth and development primarily affect the physics of the system by altering volumes, lengths, smooth and myocardial muscular tension, and physiological capacitance within the system to support the growing body. Numerous effects of aging have an impact on the adaptability of the system. Behavioral and emotional responses influence both autonomic and volitional cardiovascular and pulmonary reactions to stress. External forces include movement environment and activity level, which alter the gravitational forces on the closed pressure-volume system. An increased activity level causes exercise stress, which requires an altered demand for oxygen and nutrients to the structures providing the work. Finally, emotional stress needs to be considered as an external factor. Behavioral responses to stress can affect functional movement and cause maladaptive coping mechanisms on any or all systems. As with anything, the age, cognitive status, and relative health of an individual will dictate the potential success of these endeavors. Because oxygen transport and exchange are the primary requirements for sustaining life, efforts toward maximizing the efficiency of the cardiovascular and pulmonary systems represent a fundamental component of therapeutic practice. It is critical that no matter where a patient falls in the life span, strategies for screening, prevention, and rehabilitation of the cardiovascular and pulmonary systems be incorporated into a comprehensive plan to promote optimal mobility and independence.85 It is essential for therapists to keep in mind that all interventions have a direct or indirect impact on these systems and that it is their responsibility to monitor and manage those responses to maintain safety. A detailed understanding of the anatomy of the heart, lungs, and vessels, as well as the physiology and interrelationship of the organs involved, is essential to the practice of both physical and occupational therapy. Refer to Chapter 30 for additional information. For pediatric therapists, the added knowledge of normal growth and development of these structures is critical. From weeks 3 to 8 of fetal life, the cardiac structures are formed.63,64,86 All other structures of the cardiovascular system are fully developed and functional shortly after birth. Although the left and right ventricles are of similar size at birth, by 2 months of age the muscle wall of the left ventricle is thicker than that of the right ventricle.87 This is attributable to the fact that the left ventricle is responsible for pumping blood to the whole body, requiring a higher internal pressure and contractile force, whereas the right ventricle is responsible for pumping blood only to the lungs, a relatively low-pressure function in a healthy individual. It bears mentioning that the heart’s function begets structure. Therefore if function becomes impaired, the structure is likely to adaptively change. For example, if the resistance in the vascular system from the right ventricle to the lungs becomes increased, the right ventricle must pump harder, with a greater volume of blood, to overcome the resistance.88 Over time, this will increase the size of the ventricular walls because the myocardium is muscular tissue that is as equally capable of hypertrophy as skeletal muscle tissue. Structurally, the heart doubles in size by year 1, and its size increases fourfold by year 5. Many of the changes associated with size are complete by the time the child has reached maturity. Recall that cardiac output (CO) is equal to stroke volume times heart rate. As the size of the heart increases (increasing the volume capacity for each stroke), the heartbeat decreases and the blood pressure increases.89 Heart rate in a newborn infant is generally 120 to 200 beats per minute (bpm), 80 bpm by 6 years of age, and 70 bpm by 10 years of age.87,90 Systolic blood pressure (defined as maximal pressure on the artery during left ventricular contraction or systole) is 40 to 75 mm Hg at birth and increases to 95 mm Hg by 5 years of age.87 Blood pressure continues to rise into adolescence. The capacity to maintain exercise for longer periods and greater intensities increases through early childhood. Although cardiovascular disease is generally associated with adults, children as young as 5 years of age may show signs of or be at risk for cardiovascular disease if they do not engage in regular aerobic activity.87,91 Development of the pulmonary system occurs late in prenatal and early postnatal life.90 As the lungs increase in size, tripling in weight during year 1, the capacity and efficiency increase while the respiratory rate decreases.90 Although the vital capacity of a 5-year-old child is 20% of an adult’s, this is not usually a limiting factor during exercise.89 Overall, aerobic capacity increases during childhood and is slightly higher in boys than in girls. The overall work capacity of children increases most dramatically from 6 through 12 years of age.89 Peak oxygen consumption is achieved early in adulthood and changes in direct relation to activity levels. Lungs of an average adult at rest take in about 250 mL of oxygen every minute and excrete about 200 mL of carbon dioxide.92 As activity decreases in older adulthood, so do the structural and functional capacities of the cardiovascular and pulmonary systems. Many of these changes are a result of decreased elasticity of the tissues, decreased efficiency of the structures, and decreased ability to increase workload. CO decreases approximately 0.7% per year after age 20 years so that by age 75 years the CO is 3.5 L/min, down from 5 L/min at age 20 years.92 Functional changes include a decrease in the overall maximum heart rate from 200+ bpm through young adulthood to 170 bpm by age 65 years.87 Older adults have less elastic vessels, and resistance to the blood volume increases. Consequently, older adults reach peak CO at lower levels than do younger individuals. These cardiovascular changes may be compounded by inactivity, resulting in decreased capacity to perform activities that raise metabolic demands and increase the requirement for oxygen transport.93 The impact of these normal aging responses, however, can be reduced through structured aerobic and anaerobic activities. Conversely, physiological performance of the cardiovascular and pulmonary systems improves in response to growth and development. The nervous system encompasses the CNS and the peripheral nervous system (PNS). The CNS includes the brain and spinal cord, and it is responsible for all bodily functions. The PNS includes both the autonomic and the somatic nerves and is responsible for transporting impulses to and from the CNS.5 The capacity for humans to produce behaviors far beyond those of other animals is directly related to the complex abilities of the CNS and interneuronal communications. Over the past two decades, technological advances have enabled neuroscientists to dramatically improve their understanding of the molecular changes in the nervous system over time.94 Development of the CNS is coordinated through intrinsic influences involving the temporal and spatial coordination of synaptic connections with genetic processes, along with extrinsic or environmental factors. Initially, development of the CNS is dependent on precise connections formed between specific types of nerve cells and begins with the recruitment of cells that form the neural plate, which gives rise to the neural tube, and then differentiation of regions of the brain begins.5,95 Changes in the nervous system are predicated on critical periods, or times when different regions of the brain are sensitive to change, and occur across the life span.5,28 Differentiation of cells in the nervous system begins during the embryonic period and continues throughout adulthood.5,94 Development of the nervous system during embryonic life involves the overproduction of glial cells and neurons that, after they are no longer useful, die. Additional developmental changes noted in the nervous system include increased myelination within the brain and an increase in neuronal size.63 Much of the growth may be attributed to these changes in the nervous system and may account for the development of the infant’s brain, which increases to one half the size of the adult brain during the first year of life. Neural development, particularly in the cerebral cortex, documented early in development may emerge out of environmental demands and the need to solve problems (tasks). Consequently, experiences can alter neural networks, and more complex experiences lead to increasing complexity of the neural structures.96 Whereas researchers long supported the premise that decline of the nervous system begins generally after age 30 years, more recent studies indicate that adults, even older adults, can form new neural connections and grow new neurons as an outgrowth of learning and training.94,97 Before the work of Eriksson and colleagues, researchers and clinicians believed that structural changes in older adults, such as decreased numbers of corticospinal fibers, intracortical inhibition, and neuronal degradation in centers in the CNS, particularly the cerebellum and basal ganglia, were inevitable.98 In contrast, findings from a study conducted by Draganski and colleagues99 challenged traditional constructs that the only possible changes in the adult human brain were the result of negative changes caused by aging or pathology. Instead these researchers suggested that a direct relationship existed between learning a novel task, juggling, and structural changes in the gray matter. The authors caution that these structural changes were task specific and limited to the training period. Reexamination of magnetic resonance imaging (MRI) scans, after 3 months of no training, demonstrated that subjects no longer displayed the same structural changes as during juggler training. Loss of neurons in the centers controlling sensory information, long-term memory, abstract reasoning, and coordination of sensorimotor information negatively affects function. For some individuals this may not have significant implications. For others, CNS changes create serious functional losses. Alterations in the CNS, including altered neural control and decreased efficiency in temporal sequencing of muscle synergies, may play a role in postural instability and impaired sensation. Together these changes can result in falls.68 Although the CNS, similar to other bodily systems, may have the capacity to compensate for some age-related changes, the degree of compensation may be modulated by the complexity of the task and continuation of “practice” over time. Although some investigators have reported that neuromuscular systems in older adults may not be as flexible as systems in younger adults, new studies examining changes in mature and aging systems are still in the early stages. Neuromuscular systems in older adults may not be as capable of rapidly reorganizing muscle synergies to produce variable functional responses.98 The researchers did say that this may be related not solely to the aging neurological system but to other factors including experience, cardiovascular and musculoskeletal fitness, and current level of functional independence. Other scientists suggested an alternative view that repetition of motor activities may stimulate new growth in dendrites located proximal to neurons previously lost.68 The authors were quick to add that, although the pathways or connections may be activated, this may or may not result in improved functional ability. Implicit in performance of many functional activities is cognition. If changes in cognition coexist with changes in other systems, it may be difficult to accurately interpret the underlying causes. Cognition may be defined as awareness, perception, reasoning, and judgment.100 Cognitive development involves processes of perception, action, attention, problem solving, memory, and mental imagery. Action, from the perspective of physical or occupational therapy, may be referred to as functional movement(s) and incorporates all the processes described previously to successfully perform a specific task. Jean Piaget, one of the most recognized scientists in developmental psychology of the twentieth century, was particularly intrigued with how biological systems affect what individuals “know.”100,101 He observed interactions among children of different ages and hypothesized that younger children’s thought processes were different from those of older children as evidenced through the differences in responses between them to the same questions. Piaget proposed that cognitive development moved in a linear, stagelike progression, each stage of which involves radically different schemes.101 He suggested four stages of cognitive development, identified as sensorimotor state (infancy), preoperational (toddler and early childhood), concrete operational (childhood and early adolescence), and formal operational (adolescence and adulthood).100 He proposed that (1) sensorimotor behaviors stimulate cognitive development and (2) problem solving as a measure of cognition enables infants and young children to identify and modify motor behaviors. Piaget’s theory of cognitive development focused around how humans adapt within the environment and how these adaptations or behaviors are controlled.101 He postulated that behavioral control is mediated through schemas or plans, generated centrally. These schemas provide a representation of the world in an effort to formulate an action plan. At birth, infants’ earliest schemas are organized around reflexive behaviors that are modified as the infant adapts to the affordances and constraints of the environment. Piaget suggested that adaptations occur through two processes: assimilation and accommodation.101 He defines assimilation as a process of altering the environment around cognitive structures. An example of assimilation is when an infant, initially breast-fed, is transitioned to bottle feeding. Accommodation refers to changes of the cognitive structures to meet changing demands of the environment. Accommodation may be involved when an infant transitions from nutritive sucking (breast or bottle) to nonnutritive sucking (pacifier). Rather than postulating that infants are reflexive beings with little or no volitional movements early on, it may be more appropriate to view infants as competent beings with volitional and complex behaviors present at birth.102 Brazelton reported that a newborn infant turns toward the mother’s voice rather than toward an unfamiliar voice. In addition, research conducted by Meltzoff and Moore103 provides evidence supporting the complex nature of infant behavior. They found that infants as young as 2 to 3 weeks of age can imitate facial gestures performed by adults. Their work was supported by subsequent studies performed by independent investigators using different procedures and in different environments.104 These findings, contrary to Piaget’s proposal that infants were not capable of imitative behaviors until 1 year of age, provided scientists with a new perspective on infant behavior. Contemporary researchers approach developmental theory from a dynamic and nonlinear model.21,95,96,105 Over the past 10 years, advances in technology (e.g., diagnostic imaging, functional magnetic resonance imaging [fMRI], magnetoencephalography [MEG], event-related potentials [ERPs]) have dramatically improved the ability to document change within the developing brain.95 These technological advances coupled with developmental paradigm shifts and computer modeling have led developmentalists to propose new theoretical frameworks to explain cognitive development. One model, called neuroconstructivism, incorporates intrinsic constraints and abilities of the CNS at the most basic cellular level with extrinsic influences involving environmental experiences and interactions.95,96 Fundamental to the neuroconstructivist theory is the principle of context dependence, in which representations emerge in direct response to the structural changes in the cognitive system. Embedded within neuroconstructivism is the concept of the infant as interactive, in contrast to more traditional developmentalists’ perception of the infant as passive. Experiences that individual infants engage in vary through processes involving competition and cooperation. The processes employed during development may result in differing pathways or trajectories of development through which the outcome or behavior is realized. Despite the variability in the individual developmental trajectories, the behavioral outcome is often similar. Current theories lend support to the concept that the cognitive system integrates multimodal input to process, interpret, store, and retrieve information as a mechanism for information processing and problem solving.100 Changes in cognition, defined as relatively permanent changes in behavior, cannot be measured directly but rather must be inferred from changes observed across multiple systems. The integrative nature of movement, cognition, and perception is evident in developmental psychology literature.106 Given that these domains are interrelated, one area cannot be examined in isolation of other interrelated systems. Acquisition of motor skills is the primary mechanism for evaluating cognition and perception in prelinguistic children. In addition, as individuals grow older, changes in any system may influence functional movement. Finally, when examining functional movements, therapists must always consider the individual’s cognitive and perceptual abilities. As higher-level cognitive processing skills become apparent, the child can accurately identify relevant cues, filter irrelevant cues, and process information more efficiently. One such higher-level cognitive processing skill is executive functioning. Adolescence signals a period during which executive functioning begins to mature.107 This period may be characterized as critical in CNS development. During this critical period, production of mature, adult-like decisions requires selective attention and increased integration of information via the prefrontal cortex. During the maturation process, adolescents may exhibit inconsistent decision making, resulting in less-than-optimal outcomes. By young adulthood, as the individual approaches maturity, optimal executive decision making becomes more consistent. Another example of the multidimensional processes involved in higher-order tasks such as functional movement is found in a study conducted by Hazlett and Woldorff.108 They proposed that implicit in motor tasks are concepts of cognition including attention, perception, and information processes. This multimethodological approach examined (1) the influence of attention on sensory and perceptual processing, (2) the executive control of attention by higher centers of the brain, and (3) the processes underlying multisensory integration and the mechanisms by which attention interacts with such integration processes.108 A new paradigm that embraces the concept that memory and cognition do not deteriorate as part of normal aging is a topic of discussion in scientific literature.109 This perspective was proposed after Gould and colleagues109 conducted a study that found that adult primates continue to develop new brain cells throughout life. The addition of new neocortical neurons throughout adulthood provides a continuum of neurons of different ages that may form a basis for marking the temporal dimension of memory. These late-generated neurons play an important role in learning and memory of older adults. Changes in cognitive function are often revealed during tasks that require processing and retrieval of cognitive or motor memory. Consequences of aging include slowed information processing and increased time necessary to perform motor skills. Even though learning may take more time in older adults, once a behavior is learned, retention is similar to that of younger individuals. Of significance for older adults is delayed performance of long-standing tasks such as driving a car, which may have serious consequences for the driver, passengers, or others in the immediate vicinity of the vehicle. Delays in processing and task execution pose risks to the older adult or individuals with CNS deficits and may affect the individual’s level of independence and quality of life.110
Movement analysis across the life span
Theories of development
General systems theory
Biological and chronological age
Aging
Theories of aging
Physiological changes in body systems across the life span
Musculoskeletal system
Cardiovascular and pulmonary systems
Neurological system
Cognitive system
Stay updated, free articles. Join our Telegram channel

Full access? Get Clinical Tree
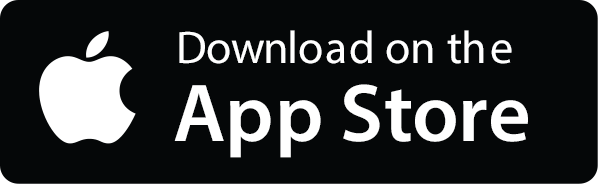
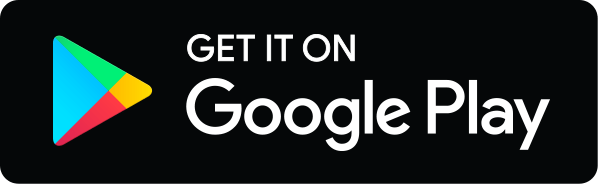