Motor Control, Motor Learning, and Neural Plasticity in Orthotic and Prosthetic Rehabilitation
Michelle M. Lusardi and Donna M. Bowers
Learning objectives
On completion of this chapter, the reader will be able to:
Why think about motor control, motor learning, or neuroplastcity?
Physical therapists and colleagues in rehabilitation help individuals with movement dysfunction improve or adapt their ways of moving so that they are safe, efficient, and satisfied with their level of function in the activities they consider important for their quality of life.1 Many different impairments, across any of the physiological structures and systems of the body, might lead to ineffective or abnormal patterns of movement and interfere with an individual’s ability to carry out activities and participate effectively in their family and social roles.2,3
Several underlying principles influence current thinking about how people move.4 The first is that movement is goal directed. Individuals move in order to accomplish a task or activity that they want to do, during self-care or other activities of daily living (ADLs), as part of their work role, or during leisure activities.5 The second is that there are many different ways to accomplish any task: the central nervous system (CNS) organizes muscles and bodies using available physiological resources in the context of the environment to accomplish any given task; there is no single “best” way of moving.6–8 The third is that each person develops preferential ways of moving: although there are many possible movement strategies available, people tend to move in ways that are most efficient for their own, individual physical characteristics.9 Preferential movement patterns, however, are not always optimal ways of moving. Repetitive motion injuries, for example, may be the result of preferential movement patterns that are not biomechanically effective, stressing tissues until inflammation or permanent deformation occurs.10 Finally, emerging evidence suggests that appropriate interventions have the potential to drive neural plasticity and enhance recovery following insult to the CNS.
When there are impairments of musculoskeletal, neuromuscular, or cardiopulmonary systems, the resources that an individual can bring to movement may be altered, limited, or constrained.11 Because movement is goal directed, an individual with impairments will find a way to accomplish movement goals that “work,” often using a less effective or abnormal movement strategy. These altered strategies are recognized clinically as movement dysfunction.12 The use of ineffective or abnormal movement patterns, over time, can lead to inflammation, tissue remodeling, or even deformity.13 In an individual recovering from stroke who is walking, for example, an “abnormal” extensor synergy of the lower extremity may provide stance phase stability but will impair swing limb advancement, leading to a compensatory circumduction or vaulting step.14 Abnormal tone may contribute to habitual plantar flexion and eventually an equinus deformity.15 Someone with a painful knee or back will alter the way he or she uses those joints, as well as the limbs or trunk, when walking and moving between sitting and standing.16–20 Over time, the individual may develop secondary musculoskeletal problems at distal or proximal joints or become physically deconditioned, compounding his or her movement dysfunction.21,22 An individual with pain, shortness of breath, or a sense of fatigue (whether due to disease or deconditioning) may choose to be less active to “conserve” energy and, as a result, become even more deconditioned, develop soft tissue tightness that impairs flexibility, and lose muscle mass that limits functional strength.23–27 Individuals who are concerned about pain, falling, or injury may also limit physical activity and have similar decrement in physiological capacity and resources.28–30
Physical and occupational therapists use various types of therapeutic exercise (e.g., strengthening, endurance programs, flexibility, balance activities), as well as functional training (often with assistive devices or ambulatory aids, orthoses, and prostheses), in order to minimize movement dysfunction and to remediate or accommodate the underlying impairments.31 To be effective in these interventions, rehabilitation professionals must understand the principles of exercise and the effect that exercise has on the human body.32–34 They must know both the purpose of an orthosis, prosthesis, or assistive device and how the design of the device will enhance or constrain movement and function.35,36 If the goal of rehabilitation professionals is to help those with movement dysfunction learn more effective ways to accomplish what is important to them, they must also be aware of the process of learning, both on a cognitive and a motor level, and integrate this understanding into the interventions they plan and implement.37
This chapter provides an overview of recent thought on motor control, using a dynamic systems perspective. The chapter also reviews the tenets of motor learning and considers practice, augmented feedback, and mental imagery as tools to assist development of new or adapted movement skills. The chapter also consider how physical therapy intervention founded on knowledge of motor control and motor learning can augment neural plasticity and recovery after brain injury. The case studies at the end of the chapter are designed to help readers integrate growing understanding of the principles of motor control and of motor learning into the planning of interventions for movement dysfunction.
Theories of motor control
Human movement has traditionally been examined from two distinct fields of study: a neurophysiological control approach and a motor behavioral approach.4,38–41 The traditional neurophysiological approach explained movement within a hierarchical system of control on the basis of the development of neural mechanisms within the central and peripheral nervous systems and the interaction of sensory and motor systems. The motor behavioral approach examined movement performance from the perspectives largely from the field of psychology. Only in the past few decades have the two fields of study interfaced to bring about newer theories regarding human movement that more fully explain human movement and performance.4,41–43 Recently, there has been an increasing emphasis on the use of therapeutic intervention as a means of driving neural plasticity as a mechanism of recovery following brain injury.44–46
Dynamic Systems Perspectives
Rehabilitation professionals think about the human body as a complex biological system with many interacting elements and subsystems (Figure 3-1). These components have an infinite number of ways to work together in accomplishing a goal-directed motor act.47–49 Because the human body is a dynamic, adaptive, and inherently complex set of subsystems, motor behaviors and ways of moving become more efficient with practice and experience: motor control systems can consistently generate simple and well-organized movement from a complex array of movement possibilities.50,51 The dynamic systems perspective of motor control is founded on understanding of behaviors that physical systems of various types have in common: the ability to change over time, the ability to be adaptive yet have preference for habitual tendencies, and the context of interaction with the environment in which movement occurs.43,52–54 In the human movement system, there is great “motor abundance”; each person has a wide variety of ways to accomplish an intended movement goal (i.e., solve a functional movement problem) in whatever environmental conditions or circumstances that function occurs.55,56
According to Bernstein’s model of motor control, individuals have the capacity “to make a choice within a multitude of accessible trajectories…of a most appropriate trajectory.”57 Dynamic systems theory suggests that there are both opportunities and challenges presented by interaction of the environment and the individual’s will to move.57 Unlike systems that are purely physical, the human biological system is a smart, special-purpose machine able to instantaneously and efficiently work to meet the many parallel and serial functional demands.58–60 In addition, biological systems such as the human body are self-organizing59,61; the mutually dependent and complex processes among the body’s subsystems allow this wonderfully dynamic structure to enact efficient functional movement patterns. Even though it is an inherently multidimensional biological system, the human body prefers to be in a state of relative equilibrium.62,63 This is likely to be the underlying reason that the gait cycle at self-selected (comfortable) walking speed tends to center around one cycle per second, and that most individuals transition from walk to run, as gait speed increases, at nearly the same velocity.64 The human body, as a smart and dynamic biological system, is also intentional; there is a purposeful, goal-directed, and task-oriented nature in most motor behaviors.65 The dynamic systems model defines an intention as a purposeful or desired act that influences (attracts) the human system to organize motor behavior toward the desired outcome, in the context of the environment in which the movement is occurring.66 The organism and the environment are interdependent; each is defined with respect to the other.58,65,66 Although the characteristics of the physical environment are the focus on many studies of goal-directed movement, the social-emotional environment can also influence the emergence of goal-directed movement.67 The combination of resources available to the organism-environment interaction, in conjunction with the individual’s intention, shapes (constrains) how task-oriented motor behavior is organized.
Motor control has been defined by Shumway-Cook and Woollacott as “the ability to regulate or direct the mechanisms essential to movement.”39 The interactive systems that provide resources that an individual uses to initiate and regulate goal-directed movement include the neurological, musculoskeletal, sensory/perceptual, cardiorespiratory, and cardiopulmonary, and the cognitive, learning, and memory systems. Human movement, or motor control, is a product of the interaction of the individual (with all of his or her subsystems), the characteristics of the environment, and the nature of the specific task or goal that the individual is involved in (Figure 3-2). Movement, then, is goal directed and purposeful; it makes use of the innate and learned resources available to the individual and is subject to the influences of the environment in which it is performed. By considering each of these essential contributors to functional movement, rehabilitation professionals can assess potential sources of movement dysfunction, explore alternative movement strategies, and adapt or alter the task or environment to improve movement outcomes.68,69
Resources of the Individual
The first component to consider in this model of motor control is the individual, with his or her ability to think and reason, to sense and perceive, and to actively respond or initiate movement (Figure 3-3). An individual’s cognitive resources include the abilities to critically think and integrate concepts, organize and delay gratification, assign emotional meaning/significance to an activity or circumstance, problem solve, access and use memory, manage attention and focus (especially when engaged in concurrent tasks), and learn.70–72 An individual’s perceptual resources are the products of the ability to receive and process many different types of sensory information (i.e., data) and to integrate and interpret these data at both subcortical and cortical levels of information processing.73–77 An individual’s resources for action include the ability to motor plan and refine motion at cortical and subcortical levels; error control systems of the cerebellum; pyramidal and extrapyramidal motor systems; and the neuromuscular, musculoskeletal, and cardiopulmonary/cardiovascular contributors to “effector” systems.78–93
To illustrate how individual resources influence movement, consider two individuals who are walking to their cars after a major-league baseball game. Their pathway moves across a gravel-surfaced parking area with a slightly sloped and slightly unstable support surface. One person has the “stocking-glove” sensory and motor impairment typical of diabetic polyneuropathy. The other has consumed a few too many servings of beer in cheering his team to victory, making his thinking and motor behavior less efficient than normal. Both are likely to exhibit less efficient postural response and unsteady gait patterns as they return to their vehicles, but for very different reasons. The quality of the sensory data that the individual with diabetic neuropathy can collect may not be sufficient for accurate perception of environmental conditions, and, complicated by distal weakness, her patterns of movement may not meet the challenges presented by the sloped and slightly movable ground surface. The individual who is tipsy has normal data collection ability; however, the temporary impairment in cognitive function (judgment and perception) and less efficient “error control” associated with alcohol consumption lead to motor behaviors that are not matched to environmental demands. Both baseball fans may stumble, walk with a wide base of support, or reach for the support of solid objects as they make their way toward their cars, but the underlying individual contributors to this motor outcome are quite different.
Nature of the Task
The task is the second essential component to the overall outcome or motor behavior performed. A functional task may require an individual to organize goal-directed movement to address one or more of the following motor control goals (Figure 3-4):
• Maintaining or adjusting antigravity posture (static, anticipatory, or reactionary postural control)94–96
• Transitioning from one stable position to another (quasimobility; e.g., moving from sitting to standing)97–99
• Moving a limb or the whole body through space (e.g., reaching, lifting, carrying, walking, stair climbing, walking on inclines, avoiding obstacles, hopping, running)100–102
• Using or manipulating tools appropriate to the task (e.g., assistive devices, objects needed for ADLs)103,104
Any task can be described along a number of different dimensions or continua: it can have discrete beginning and endpoints (e.g., transferring from bed to chair) or be continuous (e.g., walking or running over large distances). They can involve stability or require mobility, occur at various speeds, require different levels of accuracy or precision, and demand different levels of attention or focus (Box 3-1). Repetitive and overlearned tasks performed in predictable (closed or fixed) environments are often executed on a nearly automatic level, requiring little attention; this allows the individual to focus attentional resources on other prioriteis.105 Tasks occurring in a changing (dynamic or open) environment must be flexible or adaptable to be successful and require a higher degree of attention during performance.106,107 Finally, the complexity of the task and the attentional requirements for effective task performance must be considered.108
One way to understand the nature of a task (either broken into components or as a whole) is to examine or classify the task using Gentile’s Taxonomy of Movement Tasks (Figure 3-5).40 The first component considered in the taxonomy is the movement task’s outcome goals: does the task primarily focus on stability of the body, transition between stable positions, or on transport (movement) of the body through space? As an example of a body stability task, consider the ability of an individual learning to use a transfemoral prosthesis to control hip and pelvic position while in single-limb stance on the prosthetic side, while slowly lifting the “intact” limb to place it on a stool or step placed in front of him or her. A transitional (quasimobile) task for this person might be practicing moving between sitting and standing position from various seating surfaces or heights.4 A body mobility task for the same individual might be to learn to use a cadence-responsive prosthetic knee unit by altering gait speed, changing direction, or navigating through a crowded public space.
The next consideration is whether the task involves use of, or interaction with, a tool or object (i.e., is object manipulation part of the task?). In working with persons with spinal cord injuries to develop postural control in sitting, for example, therapists often use catching and throwing activities with balloons and balls of various weights, thrown at different speeds and in varying directions, to provide opportunity to master this body stability skill.109–111 Managing assistive devices (e.g., cane, crutches) during transfers or operating orthotic knee locks or prosthetic knee units during transfers are examples of object manipulation during transitional motor tasks. Learning to use an ambulatory assistive device (e.g., crutch walking in a four-point reciprocal pattern; managing crutches when ascending or descending stairs) is a prime example of a body mobility task that requires object manipulation.112,113
Characteristics of the Environment
The final component of the Shumway-Cook and Woollacott’s model of motor control is the setting, environmental context, or conditions in which the goal-directed movement takes place (Figure 3-6). The physical therapist must examine (and, during intervention, purposefully manipulate) the environmental context in which functional movement occurs. Is the physical environment comfortable to be in while taking part in exercise and other rehabilitation interventions? Is it visually interesting and stimulating but not too distracting or challenging? Is the motor task occurring in an environment that is predictable (i.e., in a closed environment), or is there a degree of variability and possibility of change external to the individual (i.e., an open environment) that will require the individual to monitor and respond more carefully while performing the task?114–116 Therapists must also consider the social-emotional context of the environment that is rooted in the interpersonal interaction: will the individual feel supported and encouraged as he takes risks and makes errors as part of the development of skill in salient activities? Or does the emotional environment contribute to anxiety about receiving negative criticism or a fear of failure?117,118
For the therapeutic application of environmental variables, therapists can consider both macroenvironmental influences (e.g., actual physical conditions that influence task demand, the therapeutic setting, or the involvement of family members), as well as microenvironmental influences (e.g., the level of visual and auditory “noise” present in the therapy room or variations in surfaces over which a client may be sitting or walking). Mastery of a motor task within a single, simple environment does not directly translate into safe performance of the same task under more complex and demanding environmental conditions. Navigating up and down a set of training steps in the physical therapy gym does not mean that the individual with stroke or paraplegic-level spinal cord injury will be functional and safe on a wet, leaf-covered, uneven brick staircase (with no railings) when entering or leaving his best friend’s house or favorite neighborhood hangout.119,120
When working with persons with acquired brain injury functioning at the Rancho Los Amigos cognitive continuum of 4 (confused and agitated), 5 (confused inappropriate), or 6 (confused appropriate), therapists must provide a structured and predictable environment for functional and rehabilitative activities, so that the demands of the environment do not exceed the individual’s ability to monitor and respond to the challenges that the environment presents.121–123 A complex environment can be overwhelming to the individual recovering from brain injury; the structured environment provides opportunity to complete key tasks with minimal frustration and behavioral complications. However, the complexity of the environment must be gradually increased as the individual prepares for discharge. Being able to cross the street safely at a crosswalk with real traffic is a more complex task than managing curbs and walking over a distance in the rehabilitation gym.
Gentile’s taxonomy provides an organizational strategy for intervention planning by rehabilitation professions working with individuals with musculoskeletal or neuromuscular impairments and limitations in performing functional activities. The primary goal of a man who has had a recent stroke may be to climb stairs so that he can return to his home, where the bedroom and bathroom are upstairs. In the early stages of rehabilitation, it may be necessary to first concentrate on static postural control and controlled weight shifting in sitting and standing on a firm support surface (body stability, no object manipulation, predictable environment). As the quality and control of these motor tasks become more consistent, intervention expands to include ambulation in the parallel bars (body mobility, no object manipulation, unvarying environment), then with an assistive device in a quiet hallway (body mobility, object manipulation, predictable environment), and finally in a busy rehabilitation gym in which the individual must anticipate and react to others in the environment (body mobility, object manipulation, changing environment). As postural control becomes more efficient on level surfaces, task demand is increased by increasing speed or attempting more challenging surfaces such as stairs, inclines, and stepping over obstacles; this may initially occur in a relatively predictable environment but must eventually occur in an “open” situation in which the individual must dynamically react to or navigate around other persons and objects.
Skill Acquisition Models
Movement has also been examined from the behavioral perspective, with a focus on quality of motor performance and acquisition of skilled behavior. Researchers with this perspective are interested in the influence of cognitive-information processing and cognitive psychology on motor behavior. They concentrate on the acquisition of skill, the learning processes associated with skills, and the refinement process of skills across applications. Early on, studies of skill acquisition focused on orientation to the task; as the field developed and expanded, focus shifted toward understanding the process of skill development. This led to formulation of the concepts of motor memory and schema. Adams’ theory of feedback-based learning was a catalyst for later research, which gave rise to Schmidt’s schema theory for motor learning.
Current motor control theory has become a blend of the various bodies of study presented in this section, integrating neurophysiological, dynamic systems/ecological, and behavioral models. This shared interest gave rise to the study of motor learning, which is concerned with the adaptation and application of movement strategies to altered or novel functional, behavioral, and environmental contexts.
Theories of motor learning
Although models of motor control focus on how the biological system organizes and adapts movement as it occurs, models of motor learning consider how the individual comes to understand and consistently perform a particular behavioral task. The outcome of effective motor learning is mastery of skilled behaviors so that the individual can function appropriately in his or her physical and social environment. Most models of motor learning are founded on four distinct notions about learning:
In order to effectively master a novel motor task or recover from disease-disrupted motor function, the individual must52,124:
Rehabilitation professionals must be careful to distinguish between the concepts of motor learning and motor performance. Motor performance is the observable action or behavior that can be measured (rated) qualitatively or quantitatively by an observer.41 As health care professionals who focus on function, therapists are quite skilled at examining motor performance, and determining whether an individual is moving normally or is coping with some form of movement dysfunction. Physical therapists use both subjective ratings (e.g., ratings of perceived exertion; using the terms “poor, fair, good, normal/excellent” to describe static postural control, dynamic balance ability, or endurance), and objective performance-based scales and measures (e.g., self-selected and fast walking speeds, Timed Up and Go times, Functional Reach distances, Dynamic Gait Index scores, 6-minute walk test distance, Gross Motor Functional Measure scores, among many others).125–131
In contrast, motor learning refers to the process that leads to changes in the quality, consistency, and efficiency of motor performance of a given individual. This process is not easily measured except by considering consistency or how other dimensions of performance of the task change over time. Comparisons of baseline performance to postintervention performance indicate changes in quality of performance. Although motor performance tends to transiently improve after a single practice session, we cannot be confident that learning has occurred until performance becomes consistent after multiple sessions over a period of time.132,133 Improvement in motor performance to the level of consistency infers that effective motor learning has occurred. Motor learning has occurred when the task can be accomplished in various ways as situations demand.
Evolution of Models of Motor Learning
Initial models of motor learning were published in the early 1970s, the most prominent being Adams’ closed-loop theory and Schmidt’s schema theory.134,135 Both models assume that, as a result of the motor learning process, the brain develops generalized motor programs: rules for timing and sequencing of muscle activity for key tasks.132 The closed-loop theory proposes that sensory information generated from movements occurring during performance of functional tasks provides feedback necessary to build the memory and perceptual traces that guide and refine subsequent performance of the task.134 In contrast, schema theory suggests that an open-loop process occurs, in which a general set of rules for a particular movement is developed (motor recall and sensory recognition schema) over time. Such schemas allow the individual to continuously compare actual outcomes of movement with anticipated (feedforward)/predicted outcomes via error detection and correction mechanism.135,136 According to schema models, variability of practice must occur to establish and strengthen the movement schema over time.137,138
In the 1990s, Newell proposed an alternative ecological model of motor learning (resonant with Bernstein’s dynamic systems model of motor control) that suggests individuals use a problem-solving approach to discover the optimal strategy to produce the task (performance) given both environmental and task influences.139,140 By exploring the perceptual motor workspace during practice, individuals begin to recognize salient sensory/perceptual cues as they explore movement options that might lead to successful task completion. In viewing a demonstration, perceptual information helps the learner better understand the nature of the task and task-related movements that need to be mastered. Perception during (knowledge of performance) and perception after (knowledge of results) task-related movement provides intrinsic feedback that assists the problem solving process in the development of optimal strategies for the task at hand. Therapists can provide augmented information (explicit cues and extrinsic feedback) to facilitate an individual’s search for optimal strategies. In this way, the perception (salient cues about the task and the environmental) and action (adaptive motor performance of the task.) are linked so that task-relevant connection is established.145
Refer to Shumway-Cook and Woollacott132 and Schmidt and Lee128 for a more detailed discussion of these theoretical models.
Temporal Considerations
Motor learning has also been explained through a temporal perspective in which learning occurs in stages over time. Various three- and two-stage models have been proposed to describe the process for acquisition of a skill and for adaptability or generalization/transfer of the skill (Table 3-1).
Table 3-1
Comparison of Concepts in the Major Models of Motor Learning
Models | Descriptive Stages/Movement Characteristics | ||
THREE-STAGE MODELS | |||
Fitts and Posner142 | COGNITIVE Early skill acquisition through trial and error; performance highly variable to find most effective strategy for task | ASSOCIATIVE Refinement of skill; performance less variable and more efficient | AUTONOMOUS Low attention necessary for task; transfer or adapting skill to other environments; performance of skill during multiple task demands |
Vereijken, Whiting, and Beek54 | NOVICE Discovery of task constraints; restriction of degrees of freedom to simplify task | ADVANCED Release of some degrees of freedom to coordinate movement; adapt tasks to environmental demands | EXPERT All degrees of freedom released; exploitation of mechanical forces to complement environmental forces |
Larin144 (Refers to children) | DISCOVERY Verbal-cognitive stage; physical and verbal guidance necessary | INTERMEDIATE Motor stage; independent performance, greater consistency | AUTONOMOUS Skilled performance; economy of effort; task adaptable to environment |
Shumway-Cook, Woollacott Systems Model132 | STAGE 1 Co-contraction to constrain degrees of freedom to reduce the number of body segments or joints to be controlled during movement | STAGE 2 Gradual release of degrees of freedom of limb segments results in gradual increase in control and flexibility of the body during movement | STAGE 3 Mastery of the skilled movement with automaticity and variability during performance, allowing adaptation and transfer of skill in response to changing conditions/demands |
TWO-STAGE MODELS | |||
Gentile148 | EXPLICIT LEARNING Attainment of action-goal; conscious mapping of the movement’s structure; rapid stabilization of performance | IMPLICIT LEARNING Dynamics of force generation; active and passive force components finely tuned unconsciously; gradual change in performance | |
Manoel and Connolly147 | ACQUISITION Formulation of the action plan; understanding task and how to accomplish it; stabilization of action | ADAPTATION Task and environment interaction; task is fluid with range of options to cope with new situations; breakdown of stable task and reorganization for new action plans |
Three-stage models generally describe the earliest stage as the discovery stage, in which an understanding of the nature of a task is developed through trial and error, sometimes with guidance.54,128,137–144 In this initial stage of motor learning, the need for attention is high, and there is significant trial and error-related variability in task performance early on, with an eventual understanding or selection of the best plan for the task for that individual. Once a plan has been settled on, the second stage of motor learning focuses on refinement of the performance; error during performance decreases while efficiency of performance increases, but attention is still required and distraction is often problematic, interfering with performance.145 In the third and final stage of motor learning, the individual can generalize or adapt the learned skill to changing environmental demands; much less attention to task is required, and the task can be performed effectively in various ways under multiple environmental demands.
Building on the work of Lereijken and colleagues, Shumway-Cook and Woollacott describe a system-oriented three-stage model, integrating principles of dynamic systems motor control, human development, and the ecological model of motor learning.132,144 Early in motor learning, individuals constrain (freeze) the degrees of freedom among limb segments (joints) involved in the task as a means of reducing task difficulty; this freezing cocontraction around joints results in relatively accurate movement, although the movment typcially has a high energy cost.146 As learning occurs, there is a tendency to “unfreeze” joints sequentially such that movement becomes more fluid and energy-efficient. With mastery, the individual employs freedom of movement to fluidly perform the task and can adapt to changing characteristics of the environment.147
Two-stage models of motor learning focus on (1) acquisition of the skill and (2) adaptation or application of the skilled motor behavior.148 The initial phase consolidates the first two components of the three-stage models: Acquisition and refinement of performance occur within the same stage. Variability of performance occurs through internal demands within the individual’s attempts to find and select a preferred action/strategy before refinement. In the second stage performance is variable due to external demands of the environment: The person must adapt the task accordingly; this is quite similar to the final component of each of the three-stage models.149
Implicit and Explicit Aspects of Motor Learning
To understand the process of motor learning, it is important to consider the principles underlying all learning processes: how new information is translated into memory to become useful. One can think about learning as the process of acquiring new information and new skills; memory then is the product or outcome of the learning process.150 Learning is one of the major drivers of plasticity within the CNS, stimulating formation of new synapses as well as refinement of existing neural connections throughout the brain.151,152 This plasticity is evident as information is moved from our short term (working) memory into initial long term memory stores which become better established, and more resistant to disruption, with practice and experience (Figure 3-7).153
Learning theorists describe two major categories of learning: implicit (nondeclarative) and explicit (declarative) learning (Figure 3-8).154 Both categories lead to functional and physiological changes in synapses of involved areas of the spinal cord, brainstem, and forebrain.155 One aspect of motor learning falls into the category of implicit procedural learning: it requires trial and error (discovery) in a relevant and functional context.155 Consistently improved task performance over time and situation provide evidence of effective procedural learning. Neural structures thought to be necessary for implicit procedural learning include cortex of the frontal and parietal lobes, nuclei of the basal ganglia, and cerebellar cortex and nuclie.156–158 Recent work has also identified that the hippocampus in involved in perceptual components of procedural learning.159 Explicit (declarative) learning is founded on attention and conscious thought and can be described or demonstrated by the learner.160 Neural structures involved with explicit learning include prefrontal cortex, cingulate gyrus (limbic system), head of the caudate nucleus, medial temporal lobes, and hippocampus.161 The hippocampus plays a key role in motor learning because it contains a cognitive-spatial map of the typical areas in which humans function.166 Early motor learning is strengthened, as evidenced by changes in output of the primary motor cortex, when explicit learning of sequences is associated with implicit learning.162–164
Once sequential aspects of the task are well understood, the need to pay close attention during motor performance diminishes, and the skill becomes less effortful as it transitions toward automaticity.165 As automaticity increases, the ability to attend to simultaneous tasks also increases.166,167 There is growing evidence that the transition from early motor learning of complex, sequentially organized tasks to automaticity requires more time and practice as a person ages.168–171 Encouragement and feedback that focus on building perceptions of capability (self-efficacy) with respect to better performance than peers appears to enhance motor learning in both young and older adults.172,173
Key motor learning concepts that physical therapists working with individuals new to prosthetic or orthotic use include practice conditions and schedule; the role of variability, contextual interference, and feedback on skill acquisition; development of automaticity of performance; as well as retention and transfer of the newly learned motor skill (Box 3-2).128,132 Each is discussed in the following section.
The importance of practice
Common to all models of motor learning is the concept of practice: Motor learning cannot occur unless the individual has an opportunity to gain experience through repeated attempts (both successful and unsuccessful) at accomplishing the desired movement task.174 Much of the research literature in the area of motor learning is devoted to exploration of practice and the optimal conditions or configurations in which it occurs. Conditions of practice can be classified or designed in several different ways. The type of practice used can influence the efficacy of motor learning that occurs, as well as its carryover or generalizability to similar tasks or environmental conditions.
Variability
In a complex and variable world, there are innumerable ways to respond to challenges that are encountered. Over the life span, typically developing individuals explore many movement options to accomplish salient task goals, developing a set of action plans that demonstrate both automaticity in performance and adaptability to variations in task or environmental constraints (i.e., the mastery of bipedal locomotion, over different surfaces, at various speeds, in closed versus open environments).175,176 Discovery learning and the feedback/feedforward provided by error during repeated practice across conditions with differing constraints allows the individual to develop an understanding (perception) of the common elements of the task wherever and whenever it is performed.174,177,178 This perception provides the flexibility to select from a range of task-specific options and adapt movement in response to variations encountered in daily life; variability in skilled movement is an adaptive resource responsive to variation in environmental demands.179
The movement patterns of individuals with neurological or neuromuscular dysfunction, however, often demonstrate hypervariability or hypovariability.176,180 Too much or too little variability is problematic: Perception of the nature of the task and of interaction between task and environment may be limited or altered, and acquisition of skill may be challenged by the constraints associated with altered muscle performance and motor control.181–183 Functionally, this contributes to a reduced ability to adapt (vary) performance in response to changing environmental conditions. The rigidity characteristic of Parkinson disease, for example, creates “super stability” of the trunk and extremities that interferes with mobility (locomotion) and postural control when task conditions change (i.e., the need to increase speed, walk through doorways, or to walk on inclines).181,184 In persons with stroke, impaired ability to move the involved upper extremity was accompanied by difficulty recognizing action of the corresponding limb when observing others or a computer model.185 In persons with right hemisphere stroke, impairments of attention and perception challenge the ability to develop the set of task-specific movement options necessary for adaptive function in response to variations in environment and context.186,187 While implicit motor learning can be successful after a stroke, movement during acquisition stages is often slower and more variable in both blocked and random practice conditions.188 Variability in movement is also altered in children with hypertonic and dyskinetic cerebral palsy, and in those with developmental coordination disorder.179,182,189
In rehabilitation, therapists set up opportunities for discovery motor learning for patients that are designed to enhance the likelihood of discovering, from among all possible movement options, the set of movements most likely to result in successful task performance.175,176 This is accomplished by manipulating the environmental and task constraints in relevant and meaningful ways to actively engage the individual in iterations of the task, with the goal of promoting both perceptual understanding and a usable set of action options for completing the task.175,190,191
Practice Conditions: Blocked, Random, or Serial?
One way to describe practice is by whether it occurs in a blocked or random sequence. Blocked practice (also known as constant practice) is characterized by separate but subsequent repeated trials of the same task.132,174 For blocked practice, the task is repeated under consistent environmental conditions. Modified blocked practice involves repeating the motor task three or more times in one condition before altering conditions or context in which practice of the same task is repeated. During blocked practice, the individual concentrates on performance of a single task; this focus reduces overall demand on working memory. As a result, quality of performance tends to improve substantially over successive bouts of blocked practice. In healthy adults, acquisition of skill appears to be effective (performance becomes more accurate over a single practice session) with blocked practice, however retention of the skill over time and transfer of the skill to differing conditions is not as strong.192 Blocked practice appears to have a positive impact on retention of skill for children younger than 10 years; this is thought to be a function of emergence of information processing capability in late childhood and early adolescence.193,194
For the individual with transfemoral amputation working on stance control on the prosthetic limb, a blocked practice session might include stepping up onto a stool with the intact limb for 10 trials (repetitions), while standing in the parallel bars. The level of difficulty of the activity could be advanced by setting up an additional practice session, asking the individual to perform a similar task while supporting himself or herself with a straight cane to a practice curb or step outside the parallel bars. Theoretically, practice in the parallel bars would provide a model to use when performing a similar activity outside the parallel bars.
Random practice (also described as variable practice) is characterized as practicing a set of tasks in which order and perhaps difficulty of tasks varies across bouts of practice.132,174 Because of the variation encountered during random practice, there is less improvement of performance in a given practice session (compared with blocked practice); however, there is greater retention of what has been learned over time. Theoretically, random practice creates contextual interference (the work of keeping many options available in working memory over time) that actually enhances learning and mastery over time despite poorer immediate performance.174,192 While this may be counterintuitive, the efficacy of contextual interference on mastery of complex movement tasks is well supported.195–199 One of the proposed mechanisms for enhanced retention in random practice is increased attentional demand that results in better use of perceptual understanding to prepare for movement.197,199 The degree of similarity of tasks (distraction) undertaken during a practice session can also be a source of contextual interference; greater attention is required to discriminate between tasks with similar but distinct characteristics.198 The improvement of retention occurs even when the context or characteristics of the task are somewhat altered; thus random variable practice may enhance transfer of learning.174
To practice the primary task of stance phase stability using a random practice order, the individual with a transfemoral prosthesis would be involved in an ongoing session of gait training. As this person walked the length of the parallel bars (or across the gym), he or she might be asked to step up onto a stool or over an obstacle at a different point in the walk and to change direction or speed on randomly delivered commands. The walk itself might be repeated 10 times (practice trials), with a step up onto or over the stool and changing speed and direction at a different point in each of the 10 trials of walking. These trials of stepping up or over and altering speed and direction do not occur sequentially but instead are interspersed throughout the entire ambulation event.
A third practice condition called serial practice can be thought of as a blending of the blocked and random practice order. Serial practice is a collection of different tasks performed sequentially, from a designated starting point to a defined ending point, always occurring in the same order, and repeated as a whole set of movement tasks.132,174
In a serial practice session for an individual learning to use a transfemoral prosthesis, the therapist may instruct him or her to repeat a specific sequence of movements such as the following:
1. Rise from a seated position and take three steps toward an obstacle in your pathway.
3. Complete three more gait cycles and turn around to the right.
4. Walk back toward the obstacle, stepping over it with the prosthesis first on the return.
5. Continue walking back to the chair, turn, and sit down.
The original task of stepping onto the stool with the right foot has been embedded into a series of different (but somewhat related) tasks performed in the same order over multiple trials.
Another way to classify practice is by the relative period of time spent in active practice versus rest time between practice sessions. In conditions of massed practice, there is more time spent over a practice trial than there is rest time between trials. Fatigue may be a factor in decreases in performance over repeated practice sessions if rest periods are insufficient. In conditions of distributed practice, the amount of practice time is less than or equal to the amount of rest time between trials.132,174 Given evidence of better retention and transfer of skills with longer rest periods between practice trials (i.e., distributed practice), rest appears to be more than a period of physical recovery; it may also enhance consolidation of perceptual schemas and action rules associated with the skill that has been practiced into memory.200 What is not yet understood is how much practice time and how much rest is optimal for tasks of different complexity or learners with various resources or impairments.
Part vs. Whole Task Training
Many functional tasks have sequential, recognizable subcomponents. The task of rising from a chair, for example, may require moving forward toward the edge of the seat, changing foot position, leaning forward to shift body weight from the ischial tuberosities toward the feet, lifting off the seat, accelerating quickly upward into standing, and then establishing postural control in the upright position.201 The task of walking can be divided into stages including weight acceptance in early stance, stability during single limb stance, preparation for swing at the end of stance, and initiation through completion of limb advancement during swing phase.202 When working with individuals who have neurological, neuromuscular, or musculoskeletal related movement dysfunction, therapists often use task analysis to determine what subcomponents of the task are problematic. The therapist might opt to practice the problematic components of the task to build skill before attempting the entire task (part to whole training) or to practice the entire task repeatedly (whole task training). In partial task training, the task is divided into separate parts, and each part is explained or modeled as distinct components of the task, while in whole task training the entire task is explained verbally or modeled (demonstrated) in entirety from beginning to end. The decision to structure training as part versus whole is influenced by level of difficulty of the task; the degree that the individual has already mastered some of the task components; the individual’s ability to attend to the task, his or her level of motivation and frustration; and safety considerations as the task is attempted. Tasks that are serial in nature lend themselves in part to whole training; spending time practicing complex or difficult task components (ending the session by putting all of the components together to perform the whole task) often leads to better retention and transfer than the same amount of time spent practicing the entire task.174,202,203 Tasks that are continuous, such as carrying a tray while walking through the cafeteria, are not as easily separated into components because of the degree of coordination and interplay necessary among task components. When coordination, timing, and interaction must be learned, whole task training appears to be more efficacious.174,203,204
Relationships: Practice, Retention, and Transfer
The effectiveness of the various practice conditions on motor learning has been the subject of many studies in psychology, movement science, and rehabilitation. As we consider the evidence that these studies present to us (to determine their clinical relevance and possible application), it is important to note the specific outcome of practice that is being investigated. Are the researchers focused on change in quality of performance during practice trials (i.e., skill acquisition within a session) or in carryover of understanding of the task from one practice session to another (i.e., postpractice performance or retention over time)? This distinction is particularly important for rehabilitation professionals to keep in mind.
How, then, do rehabilitation professionals determine whether the interventions they have implemented have resulted in effective motor learning and skill development? Rather than focusing on improvement in a single session, we look instead at the development of automaticity and the ability to adapt performance across sessions and circumstances. Although performance over repeated trials within a practice session is often observed, this is not a reliable indicator that motor learning has occurred. Consistency in motor behavior (as the product of the learning process) across sessions and over time suggests that there has been retention (consolidation), a relatively permanent change in motor behavior. The ability to transfer what has been learned and apply the set of movement options across situations appears to be related to the opportunity to practice under a variety of environment conditions and constraints.
Scientists who study motor learning hypothesize that individuals who develop flexible learning strategies through random practice and whole-task training are better able to transfer learned skills to novel situations. This is critical for the individuals we care for, who will ultimately need to perform skills beyond the rehabilitation practice environment (rehabilitation settings) as they return to the real world environment of their homes and community. Keeping this in mind, rehabilitation professionals need to carefully consider and choose the practice conditions that will lead to the best possible functional outcomes for individuals for whom they care.
Intrinsic and Extrinsic Feedback
A second key concept in motor learning paradigms centers on the provision of feedback during practice trials (Box 3-3).205 As movement occurs, it generates intrinsic (inherent) feedback that the CNS (especially the cerebellum as a system interested in coordination and error control) compares with the sensation that it “anticipates” (feedforward) will or should result from the movement. Extrinsic (augmented) feedback refers to information about the movement performance that is provided by an external source before, during, or after the movement. If rehabilitation professionals understand the “what, when, why, and how” of extrinsic feedback (and combine them with appropriately structured practice), they will be much more effective in facilitating motor learning as well as the individual’s ability to problem solve or adapt a motor skill. Rehabilitation professionals must determine what type of information is most appropriate (knowledge of performance or knowledge of results) for the individual they are working with, as well as how and when the feedback would best be provided (feedback mode and schedule).
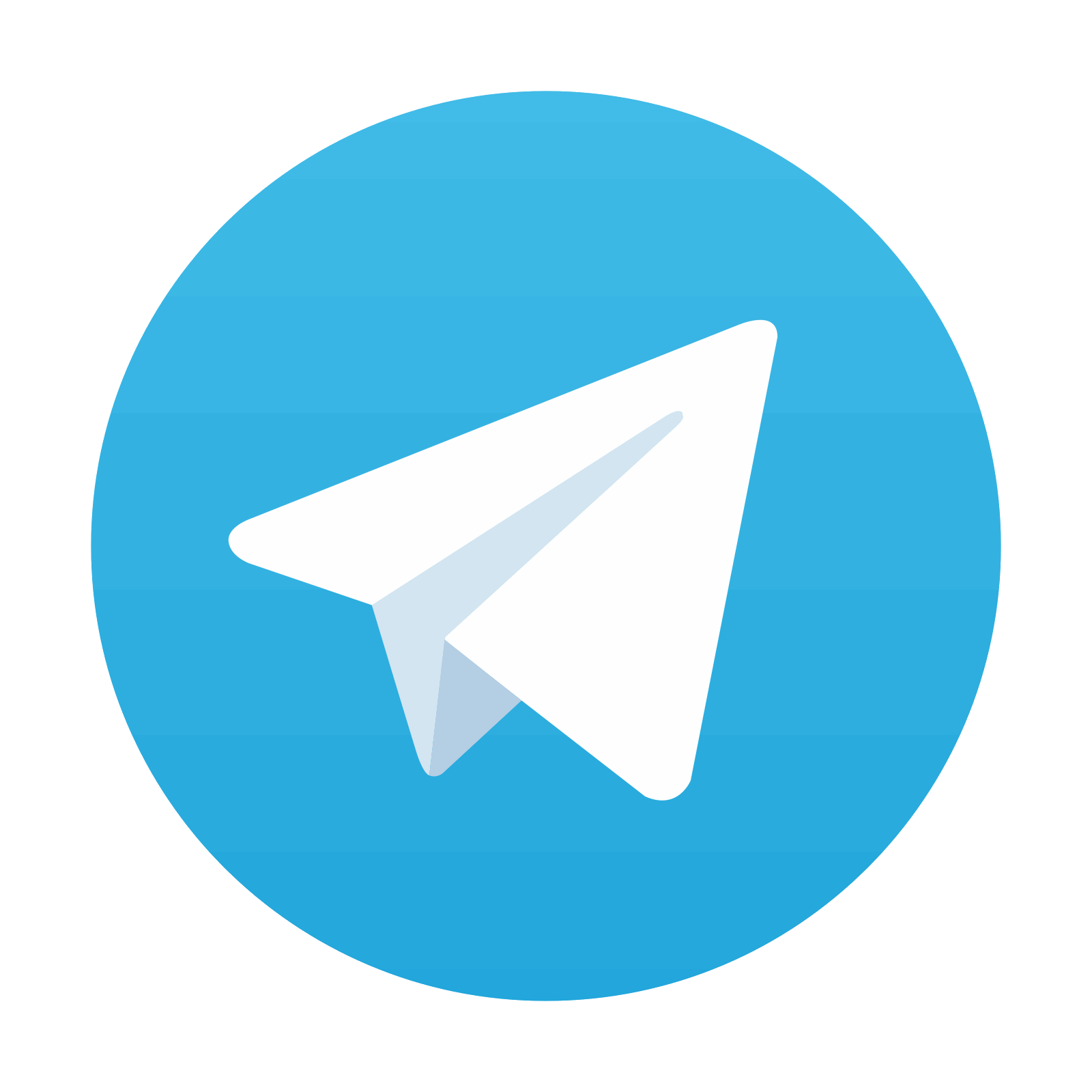
Stay updated, free articles. Join our Telegram channel

Full access? Get Clinical Tree
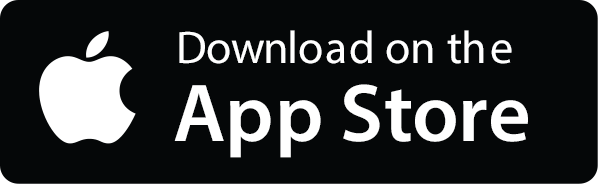
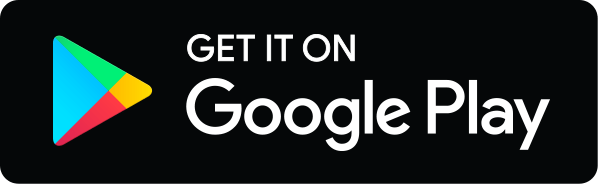