Figure 5.1 A pressure threshold inspiratory muscle trainer illustrating the spring-loaded poppet valve that provides the training load to the inspiratory muscles. (From POWERbreathe International Ltd, with permission.)
Because of its flow independence, training using IPTL can be undertaken effectively without the need to regulate breathing pattern. In addition, IPTL using a device with a mechanical poppet valve is both portable and easy to use.
Expiratory pressure threshold loading
Expiratory pressure threshold loading (EPTL) requires individuals to produce an expiratory pressure sufficient to overcome a positive pressure load and thereby initiate expiration. As is the case with IPTL, loading can be imposed at a quantifiable intensity by providing near-flow-independent resistance to expiration. This type of training has been far less extensively studied than IPTL, but EPTL has been shown to generate increases in expiratory muscle strength in both healthy people (Suzuki et al, 1995; Baker et al, 2005; Griffiths & McConnell, 2007) and patients with COPD (Weiner et al, 2003b; Mota et al, 2007) and multiple sclerosis (Smeltzer et al, 1996). Weiner and colleagues also demonstrated an improvement in the endurance performance of the expiratory muscles following EPTL (Weiner et al, 2003a).
Concurrent IPTL and EPTL
The results of studies that have imposed pressure threshold loads upon the inspiratory and expiratory muscles within the same breath cycle suggest that this type of concurrent loading may be counter-productive. For example, when IPTL was added to EPTL after a period of 4 weeks in well-trained rowers, the increase in inspiratory muscle strength over the subsequent 6 weeks of concurrent training was just 13%, which is less than half the value typically attainable when IPTL is implemented without concurrent EPTL (Griffiths & McConnell, 2007). Similar findings were made in young swimmers who undertook simultaneous IPTL / EPTL (Wells et al, 2005). After 12 weeks of training with incrementally increasing inspiratory and expiratory loads, inspiratory and expiratory muscle strength improved by only 8%. This was identical to the change shown over the same period in the sham-training control group. However, one study in healthy elderly people demonstrated improvements in both MIP and MEP following concurrent IPTL / EPTL (Watsford & Murphy, 2008). In this study, the selection of the training load was dictated objectively for the inspiratory load, with the expiratory load being adjusted arbitrarily to a level that was ‘tolerable’. This may explain the fact that significant improvements in MIP and MEP were observed in this study, but not in the one by Wells et al (2005). Generally, these data suggest that care is required when using concurrent IPTL and EPTL, as loading both phases of the breathing cycle simultaneously can generate suboptimal improvements in respiratory muscle strength.
Endurance training
Voluntary isocapnic hyperpnoea training
Voluntary isocapnic hyperpnoea (VIH) training requires individuals to maintain high target levels of ventilation for up to 30 minutes. To prevent hypocapnia, participants may simply rebreathe through a dead space. However, most studies have used more elaborate apparatus that supplies supplemental oxygen to avoid hypoxaemia while maintaining isocapnia. Training sessions are typically conducted 3 to 5 times per week at ~ 60–90% of maximum voluntary ventilation (MVV). Using VIH in healthy people, several investigators have shown increases in endurance during sustained isocapnic ventilation (Boutellier et al, 1992; Boutellier & Piwko, 1992; Markov et al, 1996; Spengler et al, 1999; Stuessi et al, 2001; Leddy et al, 2007; Wylegala et al, 2007; Verges et al, 2008b; Verges et al, 2009), maximum sustainable ventilation (MSV) (Leith & Bradley, 1976; Belman & Gaesser, 1988) and MVV (Leith & Bradley, 1976; Belman & Gaesser, 1988; Spengler et al, 1999; Leddy et al, 2007; Wylegala et al, 2007; Verges et al, 2009). The latter is consistent with improvements in peak velocity of muscle shortening. Typically, pulmonary function indices such as vital capacity and forced expiratory volume in 1 second remain unaffected by VIH (Spengler et al, 1999; Stuessi et al, 2001; Leddy et al, 2007; Wylegala et al, 2007). However, one recent study showed vital capacity increased significantly after VIH (Verges et al, 2009). Studies in patients are less numerous, but VIH appears to elicit similar changes to those observed in healthy people, when implemented in patients with neuromuscular disease (Rassler et al, 2007) and COPD (Mador et al, 2005).
Voluntary isocapnic hyperpnoea is a relatively time-consuming (typically 30 minutes per session) and physically demanding mode of RMT requiring a high degree of motivation. It also requires supplemental carbon dioxide or partial rebreathing in order to prevent hypocapnia (low carbon dioxide levels). Although VIH improves indices of respiratory muscle endurance, it does not improve the maximal pressure-generating capacity of the respiratory muscles (Leith & Bradley, 1976; Verges et al, 2008a). The influence of VIH is thus confined to the velocity (flow) axis of the force–velocity relationship of the inspiratory muscles (Romer & McConnell, 2003).
Summary
The preceding evidence points to resistance training as providing the most versatile training stimulus, as judged by the range of adaptions elicited (strength, power, shortening velocity and endurance). It is also the most time efficient and least arduous method of improving respiratory muscle function. The following section will consider commercially available training equipment, which will provide the final pieces of information required to select a suitable training method i.e., those of portability and relative cost.
PROPRIETARY TRAINING EQUIPMENT
The first part of this section will describe the range of mechanical principles that have been exploited to load the respiratory muscles. This will be followed by a description of the commercial products that utilize these basic principles. However, the latter is limited to those products that have been tested in placebo-controlled trials on patients and / or healthy young people, and shown to generate statistically significant improvements in at least one aspect of respiratory muscle function, as well as being published in peer-reviewed professional journals. The merits and limitations of each device will be summarized in order to assist the selection of the most appropriate device(s) for a given application.
Respiratory training equipment falls into two main categories: devices that impose a resistance-training stimulus and devices that impose an endurance-training stimulus.
Resistance training
These products fall into three main classes on the basis of how the load is generated:
Passive flow resistance devices
The mechanical products in this class employ simple dials that allow the user to select inspired airway orifices with differing surface area; the smaller the surface area, the larger is the inspired resistance. However, because these loads are passive, and generated by the inspired air flow (no flow = no load), they are highly sensitive to the influence of inspiratory flow rate, which makes loading unreliable. Because of their simplicity and cheapness of manufacture, the mechanical versions of these products are the most abundant proprietary devices on the market. However, because of their inherent limitations this class contains only one product that meets the requirements for inclusion in this summary, viz., the Pflex® (Respironics Inc. USA; Table 5.1). The main advantages of the Pflex® are its price (less than £15, at the time of writing) and convenience. However, training load and progression are impossible to quantify without providing simultaneous feedback of inspiratory flow rate using another piece of equipment. When used in this way, so-called ‘targeted flow resistive training’, there is no difference in the quality of the improvement in strength that can be achieved compared with pressure threshold loading products (Hsiao et al, 2003).
Table 5.1
Comparison of evidence-based proprietary respiratory muscle training equipment*
1 = poor; 5 = excellent. A = top ranked; E = bottom ranked. No score indicates no evidence.
Training quality and versatility
Participant comfort and convenience
*Please refer to the Author’s Conflict of Interest Statement on p. 138.
The addition of pressure measurement, other electronics and software to a simple flow resistor make load setting reliable and quantifiable, but also adds considerably to the cost and bulk of the equipment. The only product of this type to be supported by published data is the TrainAir® (Project Electronics Ltd., UK), which is based on the Test of Incremental Respiratory Endurance (TIRE) technique (Chatham et al, 1995) described above. The equipment requires interface to a laptop, and its cost (about £500) makes it the preserve of specialist clinics. The training is also very time consuming and strenuous. In its favour are the continuous biofeedback of training intensity, and built-in assessment of inspiratory muscle function.
Dynamically adjusted flow resistance devices
This is a new approach to respiratory training that, at the time of writing, is supported only by unpublished pilot data. However, a description is included here because this method of loading will become more widely used in the future. As described above, the inherent limitation of a passive flow resistor is that variations in flow rate result in variations in load; this has been overcome in the new generation of flow resistance devices by using continuous, dynamic adjustment of the flow resistor. In essence, the surface area of a variable flow orifice is varied within a breath according to the prevailing respiratory flow rate. The controlled variable can be either the pressure load or the respired flow rate. Currently the only product of this type available is the POWERbreathe® K-Series (POWERbreathe International Ltd.), which was launched in 2010. The product ranges in price from £250 to £450, with the higher-priced products being downloadable and programmable by the user, as well as providing real-time computer-based biofeedback during training. The ability to make within- and between-breath adjustments makes this type of device extremely versatile from the loading perspective. Of the many unique features offered by this method is the ability to taper the load according to the prevailing strength of the muscles. In the case of inspiratory loading, for example, the load can be profiled to maintain it at the same percentage of MIP irrespective of lung volume. This means that the premature curtailment of inspiration (breath ‘clipping’) that occurs with heavy-pressure threshold loading does not arise; thus, higher loads and higher tidal volumes can be achieved. As with other electronic devices, price makes this method the preserve of specialist clinics.
Pressure threshold devices
There are two products of this type that meet the requirements for inclusion in this summary. One has been applied to both the medical and sports settings (POWERbreathe®, POWERbreathe International Ltd.), the other to just the clinical environment (Threshold®, Respironics Inc.). Both products are supported by extensive, high-quality published research. The principal differences between the products are their loading ranges (see Table 5.1), mouthpiece, separation of inspiratory and expiratory flow paths and price (Threshold about £14 vs POWERbreathe® about £30). The loading range of the Threshold® renders it unusable by anyone whose baseline maximal inspiratory pressure (MIP) exceeds ~ 60 cmH2O. This is because its load-setting range of 9 to 41 cmH2O is unable to accommodate an adequate training load (~ 50% MIP) for individuals with a MIP of > 80 cmH2O. Thus, someone starting training with a MIP of 60 cmH2O, and improving by 30%, will rapidly reach the limits of the spring to provide an adequate training stimulus. The other product (POWERbreathe®) is supplied in a range of models with load settings spanning 17–98 cmH2O, 23–186 cmH2O and 29–274 cmH2O; there is also a model that is approved for UK National Health Service prescription (POWERbreathe® Medic). The POWERbreathe® product also separates inspiratory and expiratory flow paths such that the inspiratory valve is protected from expirate. Finally, the Threshold® (like the Pflex®) provides only a hard plastic tube mouthpiece that makes it challenging for some users to maintain an airtight seal, whereas the POWERbreathe® has a flexible flanged mouthpiece that is both comfortable and airtight; it can also be interfaced via a facemask. The low price of these devices make them ideal for use by patients in a domiciliary setting.
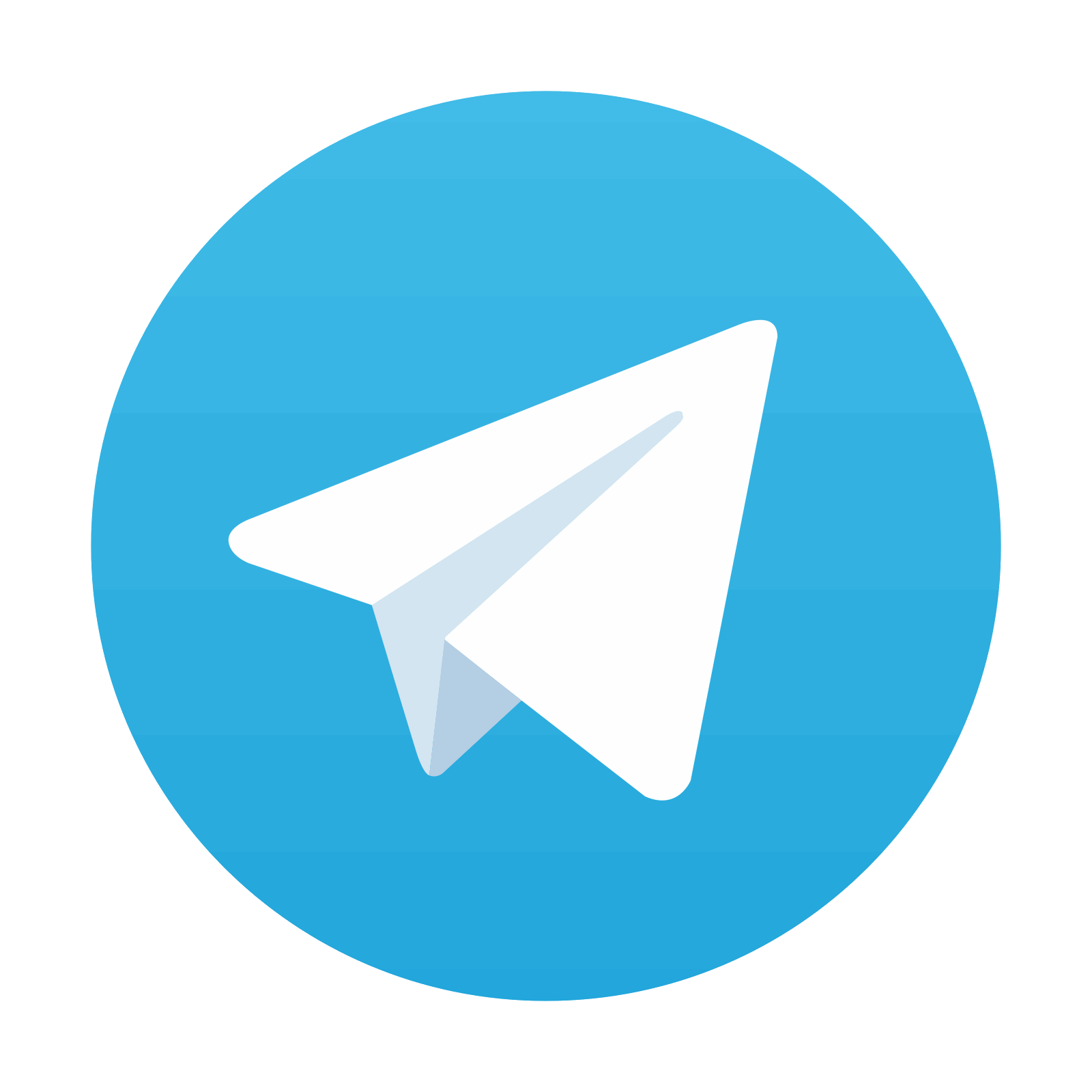
Stay updated, free articles. Join our Telegram channel

Full access? Get Clinical Tree
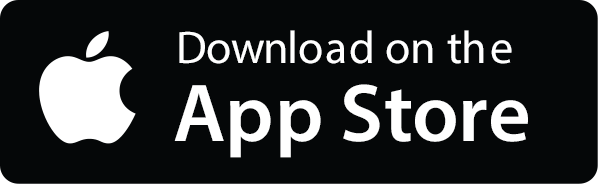
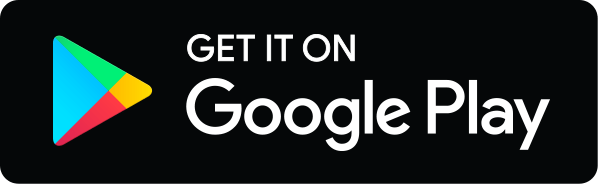