17 METABOLIC AND NUTRITIONAL MANAGEMENT OF THE TRAUMA PATIENT
OVERVIEW OF METABOLISM AND NUTRITION IN TRAUMA
The most significant factor influencing the outcome of the trauma patient is the severity of the injury.1 Nutrition care can either assist or complicate recovery. Clinical nutrition science is evolving as further evidence of care is tied to outcomes, incidence of complications, and the length of stay in the intensive care unit (ICU). This chapter addresses evidence-based nutrition management of trauma patients to enhance the nurse’s understanding and ability to be actively involved in an important interdisciplinary aspect of trauma care.
METABOLISM
NORMAL METABOLISM
Carbohydrate and Energy Production
Insulin and glucagon are the two principal hormones that control the blood glucose concentration. After a meal, absorption of glucose raises blood glucose levels, which triggers beta cells of the pancreas to release insulin. The presence of insulin facilitates transport of blood glucose into cells. Potentially life-threatening problems, as might occur in insulin-dependent diabetes, develop rapidly if the body is unable to control the blood glucose concentration within the normal range. Extra glucose that is not used for energy is stored in the form of glycogen in liver and muscle. The process of forming glycogen is called glycogenesis (Figure 17-1). Over time, as cells continue to take up glucose for energy, the blood glucose concentration decreases to the lower end of the normal fasting range. This stimulates alpha cells of the pancreas to secrete glucagon. Glucagon targets hepatic and muscle glycogen stores and triggers glycogenolysis, which is the process of breaking down glycogen to release glucose molecules into the bloodstream to maintain sufficient blood concentration.
The primary role of glucose is to provide an adequate, ready supply of adenosine triphosphate (ATP), the major chemical form of energy necessary for cell life. Glucose is converted to ATP in three steps: first, glucose is broken down to pyruvic acid during glycolysis (+2 ATP); second, pyruvic acid is converted to acetyl coenzyme A (CoA) in the cell mitochondria (+6 ATP); third, acetyl CoA is broken down in the tricarboxylic acid (TCA) cycle and hydrogens (electrons) generated from these reactions enter the electron transport chain (+24 ATP). The second and third steps in this process require oxygen consumption (VO2) and generate carbon dioxide (VCO2), free water, and heat. If sufficient oxygen is not available, pyruvic acid is converted to lactate and less ATP is produced. Hydrolysis of high-energy phosphate bonds in ATP supplies energy for biochemical reactions. Muscle contraction, hormone secretion, membrane transport, and synthesis of new substances are examples of functions that depend on ATP.
Lipid
Four types of body fat are especially important: (1) triglyceride molecules consisting of three fatty acids bound to a glycerol backbone, which are stored in adipose tissue and serve as a reservoir of energy; (2) phospholipids, which form plasma membranes and internal compartment membranes of all cells; (3) cholesterol, a vital structural component of cell membranes and the building material for steroid hormones (e.g., cortisol, estrogens, progesterone); and (4) the essential fatty acids, linoleic acid and α-linolenic acid (converted as needed into eicosapentaenoic acid and docosahexaenoic acid, which is important for cell membrane structure in the brain and retina).2
Protein
Amino acids released during protein degradation are mostly conserved and reused to construct whatever proteins the body currently requires. All leftover amino acids are deaminated. The resulting amino group is converted to urea for excretion and the carbon-chain portion of the degraded amino acid is recycled into acetyl CoA for energy substrate or converted to glycerol to make triglyceride. Of the 20 common amino acids, nine are considered essential because they cannot be synthesized in the body and must be supplied in the diet. These essential amino acids are histidine, lysine, phenylalanine, threonine, tryptophan, the sulfur-containing amino acid, methionine, and the three branched-chain amino acids: isoleucine, leucine, and valine.
Vitamins and Minerals
There are 14 essential vitamins, 12 essential minerals, and a requirement for sodium, chloride, and potassium. These nutrients play unique roles in metabolism of human life and must be consumed in the diet. An overview of the roles of essential nutrients in health and recovery is provided in Table 17-1.3 Nutrient requirements differ among healthy individuals depending on age, body size, activity level, and other factors. The body has intricate homeostatic mechanisms to maintain adequate content of essential vitamins and minerals. For example, calcium homeostasis is regulated by hormones that control the amount absorbed by the intestine, the concentration in the bloodstream, the amount deposited and resorbed from the skeleton, and the amount excreted in urine. Figure 17-2 depicts the risks of adverse effects associated with extreme levels of intake ranging from deficient to toxic.4
TABLE 17-1 Nutrition and Implications for Wound Healing and Recovery
Total Energy | |
Primary functions | Sufficient ATP production to sustain metabolism, spare lean body mass, and promote wound healing |
Indicators for assessment | Indirect calorimetry, calorie counts, body weight, BMI |
Outcomes: undernutrition | Local infection, slowed wound healing, weight loss, muscle wasting (amino acids used for energy), loss of body’s fat pads, increased risk of pressure ulcers at bony prominences, increased dependence on mechanical ventilation, slow progress in physical therapy |
Outcomes: overnutrition | Increased body fat, increased health risk with increasing overweight |
Essential Amino Acids (Protein) | |
Primary functions | Protein synthesis, enzyme activity, growth of new tissue and maintenance of body cell mass, host defense, neural and muscular function, nutrient transport, oncotic pressure; synthesis of glutathione, creatine, taurine, and neurotransmitters; secretion of mucin; gluconeogenesis |
Indicators for assessment | Diet records, blood albumin and prealbumin, arm muscle area, BUN, nitrogen balance |
Outcomes: undernutrition | Delayed wound healing; muscle wasting; edema; dull, dry, sparse, depigmented hair; psychomotor changes; decreased resistance to local infection; weight loss |
Outcomes: overnutrition | Dehydration, azotemia with possible alterations in acid-base balance |
Glucose (Carbohydrate) | |
Primary functions | Primary source of energy, especially for central nervous system, red blood cells, leukocytes, fibroblasts |
Indicators for assessment | Blood glucose, intake records |
Outcomes: undernutrition | Muscle wasting (amino acids channeled to gluconeogenesis), loss of sodium and dehydration |
Outcomes: overnutrition | Decreased resistance to local infections |
Fiber (carbohydrate) | |
Primary functions | Gastrointestinal regularity and preservation of gut barrier as host defense |
Indicators for assessment | Diet analysis, stool consistency and regularity |
Outcomes: undernutrition | Constipation; strain of defecation may increase perianal wound dehiscence |
Outcomes: overnutrition | Diarrhea with increased risk of stool contamination of perianal wounds |
Fats | |
Primary functions | Constituent of cell membrane, needed for cell growth, source of energy for resting skeletal muscle, absorption of fat-soluble vitamins in gut, synthesis of eicosanoids |
Indicators for assessment | Triglyceride level, ratio of essential fatty acids in blood, intake records |
Outcomes: undernutrition | Impaired wound healing, decreased resistance to infection, disruption of epidermal water barrier, hair loss, possible decreased attention and cognition |
Outcomes: overnutrition | Congestion of reticuloendothelial system, increased fat deposition |
Water | |
Primary functions | Nutrient transport, elimination of waste, matrix for chemical reactions, dissipation of metabolic heat, cellular growth and survival |
Indicators for assessment | Intake-output records: abnormal fluid loss (seeping wounds, drains, diarrhea, sweating) or retention (renal failure); blood sodium; osmolality; urine volume |
Outcomes: water deficit | Decreased blood flow to skin, elevated body core temperature, headache, mental confusion, physical fatigue, renal failure |
Outcomes: overhydration | Cardiovascular stress, pulmonary edema and respiratory failure |
Electrolytes (Sodium, Potassium, Chloride) | |
Primary functions | Regulate blood volume; needed for normal blood pressure, acid-base balance, nerve transmission, muscle contraction, and hormone secretion |
Indicators for assessment | Blood electrolytes, intake records |
Outcomes: undernutrition | Physical weakness, anorexia, nausea, drowsiness, irrational behavior, gastric hypomotility, cardiac arrhythmia |
Outcomes: overnutrition | Edema, hypertension, cardiac arrest |
Vitamin C | |
Primary functions | Collagen formation; needed for the function of leukocytes and macrophages; enhances iron absorption; protects DNA, proteins, and lipids from oxidation; preserves integrity of capillary structure; hormone biosynthesis; formation of norepinephrine from dopamine |
Indicators for assessment | Blood vitamin C, intake records |
Outcomes: undernutrition | Collagen synthesis impaired, poor wound healing, infection, fatigue, ecchymoses and petechiae, hair follicles with a hemorrhagic halo, joint pain, swollen or bleeding gums, dry eyes and mouth |
Outcomes: overnutrition | False-negative results for detection of occult blood; diarrhea, flushing, hyperuricosuria, hyperoxaluria, effects on red blood cell transfusions are still unknown, destruction of vitamin B12 |
Vitamin B Complex (Thiamine, Riboflavin, Niacin, Pyridoxine, Folic Acid, Biotin, Pantothenic Acid, Vitamin B12) | |
Primary functions | ATP production; protein metabolism; metabolism of therapeutic drugs; protects cell membranes and DNA from oxidation; nucleic acid synthesis and cell division for tissue growth; cell-mediated immune response; maintains integrity of hepatocytes, adrenal cortex, and nervous system; steroid synthesis and function; gluconeogenesis; neurotransmitter production |
Indicators for assessment | Blood vitamin levels, erythrocyte transketolase activity, urinary excretion of metabolites, intake records |
Outcomes: undernutrition | Slow growth of new tissue; fatigue, muscular weakness, and peripheral paralysis; tachycardia; respiratory distress; conjunctivitis; fissures at corners of mouth; swollen, beefy red tongue; papillary atrophy with smooth appearance; dermatitis; “burning feet” syndrome; neuropsychosis; nausea and abdominal distress; anemia |
Outcomes: overnutrition | Gastric upset, flushing, ataxia, sensory neuropathy, drug-nutrient interaction of folate and phenytoin |
Fat-Soluble Vitamins (A, D, E, K) | |
Primary functions | Cell differentiation and proliferation; integrity of the immune system, particularly the function of T lymphocytes; vision; calcium homeostasis and bone mineralization; protects cell membranes from oxidation; synthesis of prothrombin and clotting factors II, VII, IX, X; regulation of blood clotting |
Indicators for assessment | Blood vitamin levels, intake records |
Outcomes: undernutrition | Impaired epithelialization, cross-linking of newly formed collagen, and poor closure of wounds; dermatitis; peripheral edema; dry, rough inflamed skin; bruising; malaise; night blindness; higher incidence of severe infections; neuromuscular degeneration; anemia; impaired blood clotting |
Outcomes: overnutrition | Headache, emesis, alopecia, dryness of mucous membranes, and blurred vision; loss of muscle coordination; calcification of soft tissue; altered platelet adhesion; nutrient-nutrient interaction between vitamin E and function of vitamin K |
Calcium, Phosphorus, and Magnesium | |
Primary functions | Energy metabolism, protein synthesis, bone mineralization, nerve conduction, muscle contraction, blood clotting, -oxidation of fatty acid |
Indicators for assessment | Blood and urine concentrations, intake records |
Outcomes: undernutrition | Weakness; tetany, tremors, spasms; cardiomyopathy; anemia; nausea and gastrointestinal distress; hypokalemia, refractive to treatment; personality changes; bone demineralization |
Outcomes: overnutrition | Nausea, hypotension, bradycardia |
Zinc, Iron, Selenium, Copper, Manganese | |
Primary functions | Cross-linking of collagen and elastin, cell growth and repair; oxygen transport and energy production; defense against oxidative injury; formation and inactivation of hormones; biosynthesis of catecholamines; urea production |
Indicators for assessment | Blood minerals, ferritin, mean cell volume, hemoglobin, transferrin saturation, erythrocyte protoporphyrin; intake records |
Outcomes: undernutrition | Impaired thermoregulation, immune competence, wound healing, and growth of bone and cartilage; reduced life of erythrocytes; anemia; neutropenia; impaired taste; muscle weakness; cardiomyopathy; koilonchia (spoon-curved nails) |
Outcomes: overnutrition | Constipation, gastrointestinal discomfort, reduced immune function, nutrient-nutrient interaction of zinc and copper, psychiatric disorders, fatigue |
ATP, Adenosine triphosphate; BMI, body mass index; BUN, bood urea nitrogen.
In summary, carbohydrates, fats, and proteins are used as energy substrates. After a meal, the body stores a portion of dietary carbohydrate as glycogen to provide a ready supply of blood glucose between meals and fat serves as a vast reservoir of calories for the body to siphon in times of stress. Normal protein degradation is typically countered by a comparable rate of protein synthesis. The body uses intricate homeostatic mechanisms to absorb and retain sufficient quantities of essential vitamins and minerals. If the body is depleted of nutrients, the resulting cellular dysfunction will eventually give rise to the physical manifestations of malnutrition.5
METABOLIC ALTERATIONS IN STARVATION
Starvation occurs when there is a lack of food intake for several days or longer. The overriding goal of metabolism in starvation is to conserve energy while providing sufficient substrate to fuel processes that are vital for survival. Under these circumstances, the body is forced to lower its metabolic rate and increasingly uses fat and ketone bodies (from partially oxidized fat) as energy substrate to supplement the limited availability of glucose.6
Early Starvation
Similar to the fasted state between meals, blood glucose levels decrease in early starvation accompanied by a fall in circulating insulin levels. Alpha cells in the pancreas respond by secreting glucagon, which stimulates glycogenolysis and the release of free glucose to increase the blood concentration. However, without replenishment, tissue stores of glycogen are depleted in approximately 24 to 72 hours. To some extent, the liver and kidneys are capable of synthesizing de novo glucose from glycerol, lactate, and pyruvate and from carbon segments produced during deamination of amino acids. The process of gluconeogenesis is up-regulated by glucagon. The brain and central nervous system use about 80% of the glucose produced through gluconeogenesis. Other tissues that rely primarily on glucose for energy are red blood cells, white blood cells, bone marrow, and cells of the renal medulla. The remainder of the body, including skeletal muscle, will use both fatty acids and ketones as energy substrate. Therefore, the need for glucose is minimized and protein is spared to some extent. Catecholamine levels (e.g., epinephrine, norepinephrine) are increased during the first several days of starvation. These, along with low concentrations of glucose and insulin, trigger an accelerated release of FFA from adipose tissue and stimulate muscle catabolism for release of constituent amino acids.7,8 The effects of early starvation are outlined in Table 17-2.
Prolonged Starvation
As starvation continues, overall body temperature and metabolic rate decrease further to conserve energy. The brain and other normally glucose-dependent tissues increasingly rely on ketone bodies for energy. As the concentration of circulating ketones increases, a mild metabolic acidosis might occur, but it is usually of little consequence unless the patient is severely stressed. Fat stores eventually become depleted, requiring that even more endogenous proteins be destroyed to meet energy demands, and eventually protein compartments (both visceral and somatic) become seriously depleted. A low concentration of blood proteins decreases the osmotic gradient along the vascular lining, causing fluid to leak into third spaces, resulting in edema, particularly in the lower extremities. A lack of protein also impairs immune competence and the body’s ability to repair physical injury. Weight loss, sunken facial appearance, muscle wasting, and protruding rib bones are evident. Other signs and symptoms of nutrient depletion from prolonged starvation include anemia, fatigue, extreme weakness, decreased tolerance to cold, dizziness, muscle soreness, hair loss, skin changes, reduced coordination, ringing in the ears, apathy for matters other than food, irritability, decreased ability to concentrate, loss of sex drive, and altered personality.5 Tragic circumstances demonstrate that humans will not survive much longer than 60 days without nutrient intake. Death would result sooner if the body did not have the capacity to adapt somewhat to periods when food is scarce. Death ensues when one third of the endogenous protein supply is exhausted, even if some fat stores remain available.
METABOLIC ALTERATIONS IN INJURY
Trauma, thermal injury, and sepsis evoke a hypermetabolic response. This increased energy demand arises from degradation of injured tissue and repair of wounds, which require synthesis of large amounts of proteins and other constituents. Thus the physiologic milieu of critical illness can result in accelerated glycogenolysis, proteolysis, lipolysis, ketogenesis, and gluconeogenesis, depleting the body’s endogenous stores of protein and fat.9 Hypermetabolism coupled with the inability to ingest food set the stage for serious nutrient deficiencies.
Ebb and Flow Phases
The acute period after traumatic injury is characterized in two phases, an “ebb” phase and a “flow” phase. The ebb phase is triggered by blood loss, hypoxia, and pain and may last up to 48 hours during which cardiac output, oxygen consumption, and body temperature decline.10 Glycogen stores are rapidly depleted. Once the patient is adequately resuscitated and the initial insult is being managed effectively, the patient enters the flow phase, characterized by elevated cardiac output, oxygen consumption, elevated body temperature, and hypermetabolism (see Table 17-2).10 Many factors contribute to the intensity of the hypermetabolic response, which may last for weeks. Such factors include the patient’s age, sex, previous health status, extent of the primary injury, the loss of normal barriers to infection, medical complications, and lack of nourishment. The primary drivers of the stress response are blood-borne chemical mediators, liberated directly from the site of injury (Figure 17-3).11 In particular, interleukin-1 and tumor necrosis factor produce fever, tachycardia, and leukocytosis. These cytokines also stimulate release of growth hormone and the stress hormones (glucagon, catecholamines, and cortisol) and cause the liver to increase uptake and retention of iron and zinc.12
Macronutrient Substrate and Depletion
The stress hormones accelerate glucose oxidation and ATP production to provide for the high energy demands of maintaining vital physiologic functions in the flow phase. If tissues become hypoxic, which is common in critical illness, anaerobic conditions are created in the cells. Without sufficient oxygen, pyruvate (from glucose) is unable to convert to acetyl CoA and produce ATP. Under these conditions, pyruvic acid accumulates and reacts with hydrogen ions (NADH + H+) to form lactic acid, which diffuses out of cells into extracellular fluids, resulting in elevated blood lactate concentration.13 The heart muscle is able to take up some of the lactate and use it to produce ATP. The liver takes up another portion of the lactate for use in gluconeogenesis, which is up-regulated by cortisol and glucagon.2 Excessive liver gluconeogenesis is the primary factor contributing to hyperglycemia in unfed trauma patients.13
All aspects of protein metabolism are accelerated in critical illness. Although the synthesis of some proteins, such as the acute-phase proteins (e.g., C-reactive protein, ceruloplasmin) is increased, catabolism of protein is disproportionately greater than anabolism, resulting in net loss of protein (and nitrogen).9,14,15 In particular, albumin, transferrin, and skeletal muscle proteins undergo degradation (triggered by catecholamines and glucocorticoids) at a greater rate than their synthesis. On the basis of studies of intracellular amino acid concentrations in muscle, it has been suggested that some normally nonessential amino acids may become “conditionally essential” after traumatic injury, particularly when kidney function is impaired.16 The protein compartment in muscle suffers considerable loss in critical illness. For example, during the first week after head trauma, approximately 70% of total protein losses are derived from skeletal muscle. Alanine and glutamine comprise approximately 50% of the amino acids released from skeletal muscle,7 which are shuttled to the liver and kidneys for degradation to produce carbon chains for gluconeogenesis. After the first week, nonmuscle proteins make up an increasing percentage of protein losses.9 The hypercatabolic response peaks approximately 10 days after injury and then gradually decreases as medical status improves.15 By day 21 after blunt trauma, patients have lost an average of 6.4 kg of skeletal muscle. Trauma patients may eventually exhibit wasting of arm and calf muscle. The patient’s previous nutrition status, age, and the duration of the hypermetabolic flow phase will influence the total amount of lean body mass catabolized. Young, healthy, muscular patients have a relatively greater degree of protein wasting than leaner patients. It has been hypothesized that a reduction in release of growth hormone from the anterior pituitary gland may contribute to the wasting syndrome that characterizes prolonged illness, particularly in men.12 Proteolysis cannot be substantially modified by feeding during critical illness. Hence, lean body tissue is sacrificed regardless of whether the patient is fed enough to achieve energy balance.9,17
THE NUTRITION CARE PROCESS
Nutrition care should occur as an interdisciplinary, standardized process encompassing five main functions: (1) screen patients to identify those at nutritional risk, (2) assess the nutritional status and needs of high-risk patients, (3) diagnose nutrition problems, (4) formulate nutrition goals, plan to meet those goals, and implement interventions, and finally (5) monitor progress and evaluate outcomes. The model for care endorsed by the American Dietetic Association is presented in Figure 17-4.18 This process is cyclic, so low-risk patients are periodically rescreened and high-risk patients are periodically reassessed. In this way, patients whose risk of malnutrition has worsened since their initial screens are identified for nutrition assessment. Periodic reassessment of high-risk patients is necessary to revise nutrition plans when the patient’s status changes or if there are problems in achieving nutrition goals. Thus, the nutrition care process is designed to meet the needs of the patient throughout each phase of recovery. An added benefit from optimal nutrition care is decreased health care costs.19
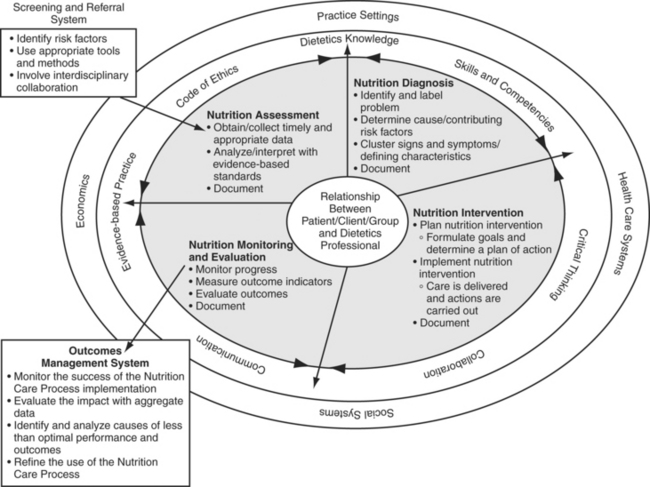
FIGURE 17-4 Nutrition care process.
(Reprinted from Lacey K, Pritchett E: Nutrition care process and model: ADA adopts road map to quality care and outcomes management, J Am Diet Assoc 103:1061-1072, 2003, with permission from the American Dietetic Association, Figure 1, p. 1062.)
NUTRITION SCREENING
Nutrition screening enables the hospital to consistently direct nutritional care to those patients most in need of these resources in a timely manner. Therefore, screening should identify patients who are already malnourished or who are at moderate to high risk for rapid development of malnutrition or a nutrition-related complication. Current standards of The Joint Commission (TJC) specify that all hospitalized patients must be screened for nutritional risk within 24 hours of admission by a qualified individual (Elements of performance for PC.2.1.120).20 This time frame was endorsed by the American Society for Parenteral and Enteral Nutrition Board of Directors, who specified that results from nutrition screening should be documented.21
After traumatic injury, medical information is collected and documented by a succession of health care providers beginning with first responders. Family members often provide initial information about the patient’s medical history, which can be refined once previous medical records are obtained. Nurses are in a strategic position to quickly identify and refer potentially at-risk patients for an in-depth nutrition assessment to determine whether special intervention is required.22,23 Early intervention can prevent malnutrition or help correct existing deficiencies.
Screening Tools and Data Collection
Most trauma centers have created their own admission screening forms. For such tools to be effective, they should be quick, easy to use, and cost-effective. An example of a nutrition screening form that can be completed within 5 minutes is presented in Table 17-3.23 Because screening is not meant to precisely categorize mild- or moderate-risk patients, some overestimation of risk may result to not miss identifying patients who are truly at risk.22 Many screening tools used in clinical practice have not been validated.24–26 Standardized examinations or tests are needed that are capable of reliably identifying all high-risk patients and minimizing the misclassification of low-risk patients.
TABLE 17-3 Example Nutrition Screening Form
Rating* | ||
---|---|---|
Factors to Rate | Minimal Risk | Potentially at Risk |
Body weight | ||
Unintentional change in usual body weight in last 6 months | Less than 10% | 10% or more |
Appearance | Relatively normal | Moderate to pronounced underweight |
Food intake | ||
Appetite and intake | Relatively good | Poor; or physician ordered nothing by mouth; or currently receives nutrition support |
Feeding | Requires little help | Only able to eat with assistance; chewing or swallowing problems |
Tolerance | Good; minor gastrointestinal complaints | Regular vomiting or diarrhea, moderate to severe gastrointestinal complaints |
Seriousness of illness, treatment, intervention | Admission to an ICU |
* If one or more ratings of “potentially at” refer to nutrition specialist for comprehensive nutrition assessmen risk, t. All other patients are rescreened for nutritional risk within 10 days.
Nutrition screening criteria include questions focusing on items that help direct the assessor to dietary or medical imbalances that might result in nutrient deficiencies. Trauma patients with chronic illness need early identification and intervention. For example, chronic renal disease is associated with protein deficiency, which can complicate recovery. The nurse should document relevant observations such as a patient’s emaciated appearance, reflecting depleted fat and muscle stores. Survey instruments are being developed for use in long-term care facilities to identify adult patients with failure-to-thrive syndrome.27
Assigning Level of Risk
On the basis of screening criteria, each patient is assigned a level of nutrition risk (e.g., either “minimal risk” or “potentially at risk”). The traumatic event itself is critical to assignment of risk in that the greater the magnitude of injury, the greater the risk for development of protein-calorie malnutrition. Patients who are not expected to consume food for a prolonged period because of severe traumatic brain injury, bowel resection, or other nutritionally disabling trauma are at high risk for malnutrition. Adults are considered nutritionally at risk if they have any of the following risk factors: (1) involuntary change in body weight of 10% or more within the past 6 months, a change of 5% to 10% within the past month, or having an emaciated appearance; (2) having a body weight above or below cutoff values for normal (e.g., 20% above or below ideal body weight, <19 body mass index [BMI]); (3) a restricted or required diet (e.g., diabetic diet, renal diet); (4) inadequate intake for more than the past week or orders for nutrition support; (5) the expectation that medical condition/injury will prohibit consumption of food for 5 or more days; (6) admission to an ICU; and (7) repeated episodes of nausea, vomiting, or diarrhea. Any one of these indications of nutrition risk would necessitate referral to a nutrition specialist.1 High-risk patients are then provided with a comprehensive nutrition assessment to determine whether a special diet, supplement, or nutritional support is needed. Patients who are at minimal risk should be periodically rescreened according to hospital protocols (e.g., within 10 days, or when the nutritional or clinical status changes).1
Screening for Risk of Pressure Ulcers
Because pressure ulcers can develop in the first week in the ICU, it is important to screen patients for ulcer risk at admission.28 The Braden score (i.e., Braden Scale for Predicting Pressure Sore Risk) is used to categorize the patient’s risk.29 Dietary status is a component of calculating the total Braden score and is scored as follows: 1 = eats less than one third of meals; 2 = eats about 50% of meals; 3 = eats more than 50% of meals or is on nutritional support; or 4 = eats most of every meal. This score is added to the other component scores to arrive at a total Braden score where ≤9 = very high risk; 10-12 = high risk; 13-14 = moderate risk; 15-18 = mild risk; and 19-23 = not at risk.28 Weight status (i.e., BMI) should be assessed as well because underweight increases the risk of ulcer development and weight status is not directly measured by the Braden scoring system. A full nutritional assessment should be conducted on all patients who are substantially underweight or who have Braden scores of ≤14.28
DOCUMENTING THE CARE PROCESS
The level of nutrition risk assigned during screening should be documented on the screening form or otherwise tracked in the referral system. Dietitians or other trained professionals who are responsible for conducting in-depth nutrition assessments of high-risk trauma patients will provide nutrition diagnostic statements of problems and will suggest plans for nutrition care.30 Because this process is highly dependent on collaboration and integration within the health care team, timely, accurate, and relevant communication of nutrition risk, assessment, problems, goals, interventions, monitoring, and progress is vital to ensure the best opportunity to achieve favorable outcomes for the patient.31
Assessment
Those patients who were rated as high risk during nutrition screening should be given an in-depth nutrition assessment as soon after referral as possible (e.g., within 48 hours of admission). TJC specified that hospitals should define in writing the type of data and information gathered during nutrition assessments. The assessment should include the patient’s nutritional status, appropriateness of the route and type of feeding, adequacy of intake, recognition of complications of nutrition therapy, and identification of education and discharge needs.18
Diagnostic Statement
Key concerns that need to be addressed through intervention by the nutrition care team are stated as nutrition diagnostic problems.18 Those most common among high-risk trauma patients often relate to a delay in feeding, underfeeding, feeding intolerance, overfeeding, electrolyte abnormalities, sudden changes in the ability to deliver nutrients through a specified route of feeding, and changes in medical status. Whenever possible, interventions are targeted at correcting the causes of high-priority problems.
Nutrition Plans, Interventions, and Monitoring
After reviewing the nutrition assessment and diagnostic statements, the patient’s physician, nurse, and the nutrition support service develop a comprehensive care plan, tailored to the needs of the individual patient, designed to achieve immediate and long-term nutrition goals. Patient-specific outcome goals must be measurable and realistic. Input is obtained as needed from family members and other relevant health care personnel. Interventions are planned, targeting the highest priority problems first, to achieve nutrition goals within an anticipated time frame. An appropriate route of feeding and type of nutrition support formula, if needed, will be identified. Whenever possible, the application of nutrition interventions should be based on available evidence that is grounded in high-quality research. The American Society for Parenteral and Enteral Nutrition is an expert organization that periodically reviews and evaluates nutrition interventions for critically ill trauma patients and publishes their consensus and guideline statements.19,31
Periodic monitoring and reassessments are conducted to evaluate the efficacy of nutrition therapy. Daily or more frequent monitoring may be required for critically ill patients, especially those at risk for refeeding syndrome and those transitioning between total parenteral nutrition (TPN), tube feeding, or an oral diet.21 For example, electrolyte monitoring may be monitored several times in one day, whereas calorie counts to evaluate progress in achieving energy goals might be conducted every 4 days. In stable patients, monitoring of nutrition support might occur weekly. Nutrition goals and plans may need to change over time to enhance the patient’s recovery and they should address discharge planning, patient and family education about nutrition therapy, and the need for home training.
THE ABCS OF ASSESSMENT
A variety of techniques used to assess nutrition status are available; the most common measures are discussed briefly. The nutrition assessment of a critically ill patient cannot depend on a single parameter but should involve multiple parameters and should take into account the entire medical status of the patient.31 Typically, the nutrition assessment is based on an evaluation of anthropometric measures (height and weight), biochemical data (composition of blood and urine), clinical data (from physical examination and medical records), dietary data (calorie counts), and measures of energy expenditure and fluid status.
ANTHROPOMETRICS AND BODY COMPOSITION TECHNIQUES
Physical Measures
Body size and composition reflect a long-term history of energy and protein status. Anthropometric measures include all physical measures of the body, such as height, weight, weight history, triceps skinfold, and mid arm circumference (MAC). In general, anthropometric data derived from a given individual are interpreted by comparison with normal values obtained from a healthy population of the same sex and age. Weight is the most widely used measure of nutrition status because it is a general indicator of malnutrition. If past weight and height measures are not available, the clinician can measure recumbent length, calculate ideal body weight, and estimate weight on the basis of visual observation of whether the patient appears underweight, normal weight for height, or overweight. Weight relative to height is an indicator of body composition and nutrition risk compared with standards for underweight and obesity. Underweight is defined as having a BMI <18.5, whereas obesity is a BMI of 30 or greater (Table 17-4). Both extremes are associated with greater morbidity. Measures of skinfold thickness using specially designed calipers at specific locations (e.g., triceps, subscapular) are a reflection of the body’s energy storage (fat). In addition, waist circumference is an indicator of the distribution of body fat. The risk for chronic disease, such as diabetes, is increased with excessive deposition of abdominal fat. MAC and arm muscle area (derived from skin fold and MAC measures) are indicators of body protein mass. Measures of triceps skinfold or MAC falling below the 15th percentile of normal are indicative of chronic undernutrition.
TABLE 17-4 Equations Used in Nutrition Assessment
Technical Measures
Bioelectrical impedance analysis (BIA) is used to estimate body composition in healthy young adults but assumes that fluid and electrolyte status are stable and normal. Other methods to assess body composition, primarily used in research, include dual-energy x-ray absorptiometry scanning to determine fat and muscle mass, gamma neutron activation analysis to measure total body protein, and in vivo tritiated water and sodium bromide dilution techniques to measure body water.32
Anthropometrics in Critical Care
Measuring actual body weight and BIA has little value for nutrition assessment in trauma care because abnormal fluid status and body casts, braces, Hoffman apparatus, and other devices confound the measures. Hence, preinjury weight and weight/height ratios, such as BMI, provide a baseline index to estimate nutrient reserves in the trauma patient. In cases of amputation, adjustments are made to subtract the estimated weight of the missing limb to estimate the patient’s weight for purposes of calculating nutrient needs. For a below-the-knee amputation, 6% of preinjury weight is subtracted, and for an above-the-knee amputation, 12% of preinjury weight is subtracted.33 However, for calculating BMI to assess overall energy reserves, the preamputation weight is used. Typically, the early postadmission weight of the patient with multiple traumas is elevated above the preinjury weight as a consequence of fluid resuscitation. Approximately 5 L or more of resuscitation fluid might be retained by the patient by the time he or she is hemodynamically stable, which adds approximately 10 kg in weight. Body weight is then lost at a rate of approximately 0.8 kg/day until the preinjury weight is restored around day 10. Weight loss will be due to loss of fluid, muscle and some fat, depending on injury severity and the adequacy of nutrition support. Patients admitted into the ICU with preexisting severe protein depletion (extremely low muscle mass), often with low body weight, are less likely to survive.34 Such severely malnourished patients should be provided with nutrition support without delay.
Anthropometrics in Intermediate and Rehabilitative Care
Calculating the percentage of usual preadmission body weight is helpful to assess the degree of depletion since admission (Table 17-4). Many patients recovering from critical illness are able to regain body protein in the months after release from the hospital. However, patients with spinal cord injuries continue to incur significant reductions in bone and muscle tissue during the first year after injury. Muscle paralysis and subsequent immobility contribute to changes in body composition. In long-term care, the typical body weight in the spinal cord–injured population is less than normal. It may be appropriate for the spinal cord–injured patient with paraplegia to be 10 to 15 pounds lighter than normal and those with tetraplegia to be 15 to 20 pounds lighter.33 Body size and composition measures may be useful to monitor patients receiving long-term care on an annual basis or when body weight changes more than 5 kg. Depending on the instrument selected, BIA may be acceptable for monitoring changes of body composition for stable patients in long-term care if the equation used is validated for age, sex, and race.35,36
BIOCHEMICAL TESTS AND NITROGEN BALANCE
Biochemical Tests in Critical Care
Before feeding is initiated, routine clinical laboratory tests are reviewed by the clinician to assess the patient’s potential tolerance for carbohydrate, fat, and protein. Depending on the degree of organ failure, patients may have hyperglycemia, hypertriglyceridemia, and other derangements that can limit their ability to tolerate feeding. Biochemical measures during critical illness, however, are not helpful in depicting nutrient stores in tissue. This is particularly true for blood concentrations of hepatic proteins (e.g., albumin, prealbumin) and minerals (e.g., zinc, iron), which decrease during the acute phase response to stress for reasons other than nutritional status. For example, albumin levels below 3 g/dl are common in critical illness and are correlated with increased morbidity, higher costs of care, and death because of the stress response.37 Moreover, blood products can contain prealbumin (transthyretin). Therefore, if the patient receives massive transfusions, the prealbumin test may reflect exogenous prealbumin rather than that synthesized by the patient. Thus, neither albumin nor prealbumin are necessarily reflective of protein status or the adequacy of nutrition support in critical care.38 Similarly, urea nitrogen loss serves as an indicator of the magnitude of injury rather than as an indicator of the amount of protein needed.
In the critical care environment, electrolytes and minerals are monitored one or more times each day and abnormalities are corrected. For example, hyponatremia can be caused by fluid overload during resuscitation or cerebral salt-wasting syndrome in patients with traumatic brain injury. Because treatment is distinctly different depending on the etiology of the disorder, electrolyte disorders such as hyponatremia are carefully managed by the physician (e.g., sodium, chloride, and fluid replacement for cerebral salt wasting).39 In contrast, vitamin levels are not routinely measured. Critically ill adults with multiple trauma receiving specialized nutrition support commonly have hypocalcemia.40 Numerous calculations have been used to estimate serum ionized calcium and “corrected” total calcium, such as the McLean-Hastings nomogram method. However, these estimation methods are not valid for use with trauma patients.41 Serum ionized calcium should be directly measured to assess calcium status.
Blood Proteins and Vitamins in Intermediate and Rehabilitative Care
Once the patient has stabilized and is past the period of acute stress, monitoring trends in prealbumin concentration might reflect nutrition status and the adequacy of feeding.15 The half-life of prealbumin is only 2 to 3 days. Hence, prealbumin concentration decreases with even short periods of underfeeding and increases rapidly with diet therapy. Corticosteroid therapy or renal failure might alter prealbumin concentration independent of nutrition status. In the absence of inflammation in the rehabilitative and long-term care setting, monitoring albumin concentrations over time is a useful indicator of protein status, but the clinician should recognize that blood albumin concentration can decline during physical stress, infection, and overhydration, even when body protein stores are ample. Because albumin has a 14- to 20-day turnover, it is not typically very sensitive to short-term changes in feeding. Yet with prolonged underfeeding of more than 2 weeks, poor protein status is associated with low serum albumin levels.
Nitrogen Balance
Nitrogen is an integral component of amino acids in food and body protein. Nitrogen balance is positive during anabolism when the body accumulates new tissue, such as during pregnancy or during growth in children. Nitrogen balance is negative when the body loses muscle mass, such as during weight-loss diets and in critical illness.15 For typical healthy adults, nitrogen balance is approximately zero when body weight, body composition, and dietary intake are relatively constant and intake is adequate. To obtain data for a nitrogen balance study, food records are kept for 24 hours. During this same period, all urine is collected and analyzed for urea nitrogen (urea reflects the amount of amine groups derived from body protein). Nitrogen balance is calculated by subtracting nitrogen losses (i.e., total urea nitrogen and obligatory nitrogen lost in skin, feces, drains, and menstruation) from nitrogen intake. On average, every 6.25 g of dietary protein contains 1 g of nitrogen. Hence, the amount of nitrogen in the diet is estimated by dividing the total grams of protein consumed by 6.25. Typically, a factor of 4 is used to estimate obligatory losses (Table 17-4).4
Nitrogen Balance in Critical Care
Many trauma patients in the ICU are highly catabolic and lose large amounts of urinary nitrogen because of metabolic stress. In general, the more severe the traumatic injury, the greater the amount of urinary nitrogen excreted. Peak nitrogen excretion typically occurs between 5 and 10 days after injury, which corresponds to the peak period of total body protein loss.9,15 The usefulness of nitrogen balance in the clinical setting is controversial. First, calculating balance depends on small differences between two large numbers of nitrogen intake and nitrogen output, whose measure may be inaccurate.16 Nitrogen output may be underestimated in patients with burns, diarrhea, vomiting, fistula drainage, and other abnormal nitrogen losses unless all body fluid losses are analyzed. Moreover, day-to-day measures of nitrogen balance in critically ill patients can be highly variable even when feeding remains consistent; these measures are not likely to represent the adequacy of nutrition support. Because of difficulties in accurately measuring and interpreting nitrogen balance, further research to develop new methods to better evaluate amino acid requirements after trauma must receive high priority.16
Nitrogen Balance in Intermediate and Rehabiliative Care
Once the patient is transferred to home or the long-term care setting and feeding regimens and intake are stable and consistent, then nitrogen balance may be indicative of the adequacy of feeding. At this point in recovery, one would expect the patient to be moving toward a neutral or more positive nitrogen balance. However, skeletal muscle protein can continue to break down at an accelerated rate during convalescence, even when stress hormones have returned to normal levels. Not all causes of continuing protein catabolism are known. Patients who have spinal cord injury may continue to lose nitrogen because of denervation and disuse of paralyzed muscles. Persistent negative nitrogen balance studies or studies that are not showing progress toward a positive balance might reflect failure of the prescribed nutrition plan, necessitating reassessment of energy and protein requirements.
CLINICAL EXAMINATION AND REVIEW OF SYSTEMS
Clinical data are reviewed to understand how the patient’s past and current medical status may affect nutritional status. Of particular importance is the type and severity of injuries and a current review of systems (e.g., neurologic, pulmonary, renal). Certain medical conditions might indicate a need to modify the route of feeding or the type of nutrients in the feeding solution. For example, maxillofacial trauma may limit the patient’s ability to chew or to take any nourishment orally. A visual examination of the patient contributes to identification of nutrient deficits, excess, or fluid imbalance (Table 17-1). Also, observations of the setting and current circumstances, such as the elevation of the head of the bed, volume of output in drains, whether the patient has gastric tubes, and whether the patient is mechanically ventilated will supplement and update information obtained in the medical chart. An assessment of the patient’s capacity to tolerate feeding and the likely start time for feeding should be made as soon as possible. Most multitrauma patients have disruption of several physiologic systems and have undergone extensive surgical interventions, which render the stomach useless for 48 hours or longer because of gastric ileus. Paralytic ileus is also common initially in the spinal cord–injured population because of autonomic disruption and ischemia at the time of injury.15 Gut function is assessed by tracking the volume of nasogastric aspirate, stool output, abdominal distention, pain or tenderness, and the presence of bowel sounds.42 Abdominal trauma requiring bowel surgeries might eliminate the upper gastrointestinal tract as a route for nutrient intake and a jejunostomy tube for feeding might be required.
Patients with severe head injuries are initially dependent on nutrition support. The risk for swallowing impairment and aspiration is increased with neurologic injury, particularly if the patient was intubated for 2 or more weeks or has a tracheostomy. The level of impairment should be assessed by a trained professional (i.e., speech pathologist). Symptoms of dysphagia that the nurse might observe include drooling, choking, coughing during or after meals, lack of gag reflex, and gurgle noises while talking.33 A patient whose level of consciousness improves may be ready to convert from enteral to oral feedings if the gag reflex returns.
DIET AND FLUID STATUS
Diet Assessment in Rehabilitation
During the rehabilitative phase of care, the nutrition assessment should include a patient/family interview to obtain additional information about the patient’s food, cultural, and meal pattern preferences. Otherwise, nutrition interventions may fail to individualize feeding to the patient.33 The interview also permits clarification and correction of the patient’s nutritional history including weight, intake, activity level, and feeding disabilities.
Fluid Status
Water consumption and adequate body hydration is necessary for metabolism and other physiologic processes, including cognitive function and regulation of body temperature and blood pressure. The average 70-kg man has approximately 42 L of total body water, dispersed primarily in lean body mass with about 3.2 L in plasma.43 For healthy individuals, the requirement for water depends on diet, physical activity, and environmental temperature. Most body water is lost through urination, and evaporation accounts for additional losses from lungs and skin. Increased sodium and protein intakes lead to increased obligatory water loss in urine. Drinking and eating in response to thirst and hunger sensations helps maintain water balance. Men and women typically consume 3.7 and 2.7 L per day, respectively, of total water (in food and beverages).
Plasma or serum osmolality is a widely used hematologic index of hydration status. Normal plasma osmolality is in the range of 280 to 290 mOsmol/kg. Plasma osmolality must be measured directly after a blood sample is collected and centrifuged because changes in pH, dissolved carbon dioxide, and lactic acid during cool storage can decrease and confound measures of plasma osmolality.44 The kidneys help regulate water homeostasis by altering the concentration of urine to excrete more or less water as needed to maintain normal plasma osmolality. On average, healthy women excrete a little more than 1 L per day of urine (30 to 65 ml/h) and men, on average, excrete 1.4 L per day of urine (38 to 75 ml/h).44 The specific gravity of urine (density as mass per volume) can be measured to assess hydration status. During dehydration, urine specimens normally range from 1.013 to 1.029 but can exceed 1.03 if the urine is especially concentrated to conserve water. Values >1.02 should be further monitored. In contrast, when the kidneys dilute urine to rid the body of excess fluid, the specific gravity of urine decreases (1.001 to 1.012).44
Isotope dilution techniques are the gold standard for assessing body water. However, reference tables of normal values for total body water by age and sex still need to be compiled.44 Combined tracer dilution methods with deuterium to measure total body water and bromide to measure extracellular fluid are being used in research hospitals to assess hydration and body cell mass in pediatric patients with thermal injuries.32 In the future, noninvasive whole body scans for total body chloride, potassium, and sodium by neutron activation analysis may be available to calculate intracellular and extracellular body water.
Fluid Status in Critical Care
In critical illness, after initial fluid resuscitation, the need for fluids continues to be increased above normal by thermal injury, hemorrhage, hyperventilation, mechanical ventilation, fever, wound drainage, neurosweats, elevated urine output, and protracted vomiting and diarrhea. Critically ill trauma patients with functioning kidneys have urine outputs of more than 0.5 ml/kg per hour (e.g., ≥35 ml/h for 70-kg man) and are often polyuric, excreting in excess of 2.5 L per day.40 Adding another layer of complexity, the regional distribution of fluid in the body is altered in critical illness, with increased fluid volume in the thoracic region. Moreover, distribution of body water between intravascular and extravascular spaces and intracellular and extracellular spaces is often altered in critical illness. These alterations in fluid status are frequently accompanied by electrolyte abnormalities. The medical team attempts to tightly monitor and control fluid balance during the acute phase of care by calculating fluid input and losses and by tracking measures of electrolytes. Renal failure and diuretic therapy alters the renal response to fluid, and these factors should be considered when interpreting urine study tests such as specific gravity and urine osmolarity.45 Kraft et al46 reviewed the etiologies of fluid and electrolyte disorders in the ICU and provided guidelines for correcting such disorders. In general, overhydration seems to be more common than dehydration in the ICU.
Fluid Status in Intermediate and Rehabilitative Care
In contrast to acute care, dehydration seems to be more of an issue in intermediate and rehabilitative care. The following patients should be monitored regularly for hydration: those who have language barriers, cognitive deficits, require assistance with eating and drinking, have orders for fluid restriction or NPO, sweat excessively, are under restraint, are dependent on nutrition support, or receive medications or feedings that promote diuresis.47 In particular, concentrated tube feedings that are high in protein can cause urea diuresis with subsequent dehydration. In general, urine osmolality near 900 or greater is an indication of dehydration. Metabolic signs of dehydration include elevated blood urea nitrogen (BUN), increased BUN/creatinine ratio, and increased blood sodium levels. Clinical signs of dehydration include oliguria, poor skin turgor, dry mouth and increased thirst, dizziness, weight loss, and an elevated heart rate (to compensate for reduced central venous pressure).48 Dehydration is a risk factor for pressure ulcers because of reduced skin turgor and circulation,49 it can result in constipation, and even mild dehydration of 1% to 2% of body mass can reduce alertness and cognitive function.44 Consensus guidelines are available for assessing hydration status of patients in rehabilitative and long-term care and include strategies for maintaining adequate hydration.47,50
Volume overload is possible during nutrition support, infusion of intravenous fluids, or organ failure. Acute weight gain is a sensitive sign of volume excess and edema may be evident once 2 to 4 kg of fluid weight is gained.45 Note that edema can also be caused by protein deficiency. For ambulatory patients, excess fluid can accumulate in the lower extremities, whereas fluid settles in the sacrum and buttock regions in patients who are bedridden.
ENERGY
Basal and Resting Energy
The amount of energy needed for the involuntary work of the body to sustain life is referred to as the basal metabolic rate (BMR). The BMR corresponds to caloric expenditure (heat production) in an awake, rested state and reflects functional activity of vital organs, gland secretion, cellular metabolism, and maintenance of muscle tone and body temperature. The BMR varies between individuals depending on body surface area, body weight, age, and sex. In general, larger individuals have a greater volume of metabolically active tissue and have a higher BMR. Studies show that women who are matched with men of the same body size actually have a lower BMR because of differences in body composition. Typically, women have a smaller proportion of lean muscle mass (and more fat mass) and therefore less metabolically active tissue. At rest, women expend 0.8 to 1.0 kcal/min (1150 to 1440 kcal/d) and men expend 1.1 to 1.3 kcal/min (1580 to 1870 kcal/d).4 For comparison, the heat released by a burning candle or a 75-watt light bulb is similar, about 1 kcal/min. In stable, mechanically ventilated patients, the energy expenditure measured at rest is not only dependent on body weight and height but is also significantly correlated with body temperature, minute ventilation, and arterial blood saturation.51 Resting energy expenditure (REE) measures of 110% or greater than normal are indicative of hypermetabolism.
Total Energy Expenditure
Physical activity, BMR, and the thermogenic effect of food all contribute to the body’s total energy expenditure (TEE). The thermogenic effect of food is the energy required for digestion and absorption of food and the stimulating effect of nutrients on metabolism but this represents only a small portion of energy. The major variable of energy expenditure in ambulatory patients is the intensity and duration of physical activity. Additional factors that can influence energy expenditure in critical illness are presented in Table 17-5.51–54
TABLE 17-5 Clinical Factors Associated With Altered Energy Expenditure
Clinical Factor | Increases Expenditure | Depresses Expenditure |
---|---|---|
Feeding | < div class='tao-gold-member'> Only gold members can continue reading. Log In or Register a > to continue
![]() Stay updated, free articles. Join our Telegram channel![]() Full access? Get Clinical Tree![]() ![]() ![]() |