Chapter 35 Meniscal Allograft Transplantation
Our understanding of the function of the meniscus has evolved over the past half- century. Historically, the meniscus was considered a vestigial remnant of leg muscle that could be removed without any harmful effect.66 Our views of the meniscus changed when Fairbanks23 reported the importance of the meniscus in the protection of articular cartilage of the knee and described the radiologic changes that occur following meniscectomy. Currently, every attempt at preserving the meniscus is made; however, meniscal repair or a partial meniscectomy is not always possible, and total meniscectomy may be the only available option. Meniscal allograft transplantation has become a viable option for patients who develop pain following total or near total meniscectomy. The first meniscal allograft was associated with complete knee transplantation over a century ago.43 In 1984, Milachowski and colleagues45 performed the first modern meniscal allograft transplantation in humans. Since the first meniscal transplantations, our techniques have improved and transitioned from open procedures to arthroscopy-assisted procedures. As of 2002, almost 4000 transplants have been performed worldwide and almost 800 procedures are performed annually.62 The number of meniscal transplants performed each year continues to increase, making it important for the orthopedic surgeon to understand the indications, complications, multiple techniques, and results of this procedure.
Meniscal Anatomy and Function
Anatomy
The structural orientation of the collagen fibers is related to its function. Collagen fibers are primarily oriented in a circumferential manner. Radially oriented fibers function as ties for the circumferentially oriented fibers and resist longitudinal splitting of the meniscus. During axial loading, the femoral condylar surfaces displace the menisci radially because of their concave wedge shape. The menisci convert axial load into tensile strain. Because menisci are anchored anteriorly and posteriorly, this displacement generates circumferential hoop stress that resists extrusion of the menisci from between the femoral condyle and tibial plateau. Biomechanical studies have shown that the meniscus is 100 times stronger and stiffer in the circumferential direction compared with the radial direction. The low circumferential shear strength is believed to be in part responsible for the occurrence of longitudinal tears.56
The menisci are relatively avascular, especially in the inner third of their body. The limited peripheral blood supply mainly comes from the lateral and medial genicular arteries. A perimeniscal capillary plexus within the synovial and capsular tissues of the knee joint arises from these feeding vessels. In the adult, vascular penetration is 10% to 30% of the width of the medial meniscus and 10% to 25% of the width of the lateral meniscus.6 Because the menisci are predominantly avascular, nutrition to the meniscus must be derived through diffusion or mechanical pumping.
Knowledge of the meniscal anatomy, in particular bony attachment sites, is imperative when performing meniscal transplantation. The menisci form a semilunar wedge-shaped structure that fills the void created by the incongruous femoral condyle and tibial plateau. The medial meniscus is an oval-shaped fibrocartilaginous structure covering almost 30% of the medial tibial plateau. The anterior and posterior horns of the medial meniscus are further apart than the anterior and posterior horns of the lateral meniscus. The anterior horn of the medial meniscus is a flat fan-shaped structure that inserts in line with the medial tibial eminence 6 to 8 mm anterior to the anterior cruciate ligament (ACL) tibial insertion. The insertion site is under the patellar fat pad at the junction of the medial tibial plateau and anterior tibia, and often requires anterior fat pad débridement for good visualization. Landmarks demarcating the bony insertion of the anterior horn of the medial meniscus include the anterior border of the ACL tibial insertion, articular margin of the anteromedial tibial plateau, and anterior intercondylar fossa.36 The anterior horn of the medial meniscus is often also secured by the intrameniscal ligament, which connects to the anterior horn of the lateral meniscus. The posterior horn of the medial meniscus inserts directly anterior to the tibial insertion of the posterior cruciate ligament (PCL), on the downslope of the posterior intercondylar fossa, behind the posterior horn insertion of the lateral meniscus. The PCL, medial tibial spine, and articular margin of the posteromedial tibial plateau serve as arthroscopic landmarks for the insertion of the posterior horn of the medial meniscus.
The lateral meniscus covers approximately 50% of the lateral plateau and has a more circular shape compared with the medial meniscus. The insertion site of the anterior horn of the lateral meniscus is directly anterior to the lateral tibial spine and adjacent to the tibial insertion of the ACL. Arthroscopic landmarks include the anterior half of the ACL tibial insertion, lateral tibial spine, and articular margin of the anterolateral tibial plateau. The posterior horn of the lateral meniscus inserts directly posterior to the lateral tibial spine, adjacent and anterior to the insertion of the posterior horn of the medial meniscus. Arthroscopic landmarks identifying the insertion of the posterior horn of the lateral meniscus include the posterior border of the ACL tibial insertion, lateral tibial spine, and articular margin of the posterolateral tibial plateau.36
Function
The meniscus serves several important functions in the knee, including load bearing, shock absorption, joint stability, and joint lubrication. During weight bearing, the meniscus experiences several stresses including tensile, compressive, and shear stresses. The medial meniscus transmits 50% of the joint load of the medial compartment whereas the lateral meniscus transmits 70% of the joint load of the lateral compartment. This increases to 85% of the joint load when the knee is flexed to 90 degrees.2 Radin and colleagues54 have demonstrated that these loads are well distributed when the menisci are intact. Total medial meniscectomy leads to 50% to 70% decreased contact area and an increase in contact stress of 100%.27,37 Because the lateral meniscus carries a higher proportion of the joint load because of the surface area, a lateral meniscectomy leads to a significantly higher increase in contact force compared with a medial meniscectomy. Total lateral meniscectomy leads to a 40% to 50% decrease in contact area and an increase in contact force of 200% to 300% compared with the intact meniscus.7
The biphasic nature of the meniscus allows it to function as shock absorber. Interstitial fluid flow and solid matrix deformation during loading cause the meniscus to act as a viscoelastic material, leading to creep and stress relaxation. Knees with an intact meniscus have a 20% higher shock absorption capacity compared with knees that do not have intact menisci.73 In addition to their other functions, the menisci also play an important role in enhancing joint stability.41 Medial meniscectomy in the ACL-intact knee has little effect on anteroposterior motion, but in the ACL-deficient knee, it results in an increase in translation of almost 60% at 90 degrees of flexion. Force on the medial meniscus increases by 52% in extension and 197% at 60 degrees of flexion in the ACL-deficient knee.3 The conformity created by the meniscus promotes the viscous hydrodynamic action required for fluid film lubrication. This lubrication assists in the overall lubrication of the articular surfaces of the knee joint.56 The multiple functions of the meniscus depend on their microstructure and macrostructure, both of which can be addressed by meniscal transplantation.
Natural History of the Meniscus-Deficient Knee
In 1948, Fairbanks23 described the changes that occur in the knee following meniscectomy, including ridge formation, narrowing of the joint space, and flattening of the femoral condyles. These changes lead to alterations in the biomechanics of the knee joint. Cox and associates19 have studied the effects of partial and total meniscectomy in dogs. Partial meniscectomy led to less severe degenerative changes, with the degree of degeneration directly related to the amount of meniscus resected. In the dogs with total meniscectomy, the degree of degenerative change was directly related to the amount of missing fibrocartilage. It was concluded that the knee menisci function to protect the articular cartilage from degenerative damages. McGinty and coworkers44 have compared the outcomes of patients undergoing total and partial meniscectomy. Using the Fairbank criteria, they found that patients with partial meniscectomy have less narrowing of the joint line compared with patients with a total meniscectomy. They identified early radiographic degenerative changes in 62% of 89 patients treated with total meniscectomy compared with 36% of 39 treated with partial meniscectomy. Because the lateral meniscus carries a higher percentage of the load force of the knee, degenerative changes generally progress more rapidly in the lateral compartment following lateral meniscectomy.35,78
Biology of the Transplanted Meniscal Allograft
Several characteristics of the meniscus make it optimal tissue to transplant. First, meniscal tissue elicits a minimal immune response. Immune reactions have been described in 1.3% of transplants reported in the literature.64 Ochi and colleagues48 placed fresh meniscal allograft in the subcutaneous tissue of mice and evaluated their immune response. They detected no specific antibodies in the serum throughout the 24-week period after grafting. They concluded that in mice, fresh meniscus was not immunogenic. Although the normal meniscus consists of relatively few chondrocytes embedded in an extracellular matrix, it also contains class II and ABH-positive endothelial cells and class II–positive synovial cells.40 These antigens are present at the moment of transplantation and could evoke an immune response in the host that would modulate the results of meniscal allografting. Rodeo and associates57 have performed an immunologic study that identified class I and II human leukocyte antigens (HLAs) on frozen meniscal allografts. Twenty-eight deep frozen and nonirradiated samples were evaluated. Overall, 9 of 12 specimens contained immunoreactive cells, including B lymphocytes or cytotoxic T cells in the meniscus or synovial tissue. No frank immunologic rejection was identified. The immune response to the meniscal allograft does not seem to affect the clinical outcome of the transplantation.
The primary functions of the meniscus can be accomplished, even if the structure is devoid of live cells. The meniscus is for the most part acellular, and most of its function is derived from its structure. The fate of the meniscal cells that accompany the meniscal allograft is unknown. Arnoczky and coworkers4 have studied the cellular repopulation of deep-frozen meniscal allografts. The menisci appeared to be repopulated with cells that originated from the adjacent synovium; however, the central core of the meniscus remained acellular. There was also loss of collagen orientation in the superficial layer of the meniscus. The structural remodeling associated with the cellular repopulation of deep-frozen meniscal allografts may make the transplanted meniscus more susceptible to injury. Jackson and colleagues33 have used DNA probes to determine the fate of donor fibrocytes following meniscal transplantation. The results of this study demonstrated that host cells rapidly repopulate the transplanted meniscus. There is no evidence that these new cells will maintain the extracellular matrix of the meniscus on a long-term basis. Similarly, DeBeer and associates20 have shown that human cryopreserved meniscal allograft donor cells are 95% replaced by host cells 1 year after transplantation. Wada and coworkers76 have noted that frozen meniscal allografts show collagen remodeling coincident with revascularization and cellular repopulation. Types I and III procollagen mRNA levels were elevated, representing active remodeling. These data indicate the adaptation of the repopulating cells from the host to the frozen allograft at 26 weeks after transplantation. In the goat model, Jackson and colleagues34 have shown the proteoglycan concentration to be decreased in the meniscal allograft. They also noted that transplanted menisci undergo gradual, incomplete revascularization, with new capillaries derived from the capsular and synovial attachments. Our understanding of meniscal biology following transplantation has improved, but further research is necessary to answer several unanswered questions.
Graft Preparation and Disease Transmission
Prevention of disease transmission with meniscal transplantation begins with careful donor screening. Transmission of HIV with frozen connective tissue allografts is estimated to be 1 in 8 million.10 Blood and tissue from the donor are sampled at the time of graft harvest and remain in quarantine while being tested for hepatitis B and C, syphilis, and human immunodeficiency virus (HIV). Tissue is procured within 12 hours of death for fresh grafts or within 24 hours if the body is stored at 4° C. Grafts are processed by débridement, pulsatile ultrasonic washing, and use of ethanol to denature proteins. Beginning in early 2005, HIV and hepatitis C screening were performed using direct nucleic acid testing by the polymerase chain reaction (PCR) assay. This method decreases the window that these viral infections can be missed, from 4 to 6 weeks down to 10 days.11 Harvesting is done in a sterile fashion or a clean nonsterile environment and secondarily sterilized. Secondary sterilization methods include the use of ethylene oxide, gamma radiation, or chemical means. Negative effects to the meniscal allograft have been reported with secondary sterilization. Gamma radiation at the level required to eliminate viral DNA may adversely affect the material properties of the meniscus.68 Yahia and Zukor75 have shown that exposure to more than 2.5 mrad of gamma radiation negatively affects the mechanical properties of collagen-containing tissues. Because 3 mrad of gamma radiation are recommended to eliminate HIV DNA, gamma radiation is not recommended. Ethylene oxide has been discontinued because of reports of graft failure and synovitis. Ethylene chlorohydrin, a byproduct of ethylene oxide, has been found to induce synovitis in musculoskeletal allografts.34
The optimal method for meniscal graft preservation has yet to be determined. Currently, there are four primary preservation methods, including fresh, cryopreserved, fresh-frozen (deep-frozen), and freeze-dried (lyophilization; Table 35-1).
Fresh graft preservation leads to logistic issues for transplantation. The short period of time to size and perform serologic testing properly makes finding a suitable recipient difficult. Because of these issues, fresh grafts have limited use in clinical practice today. The question still remains whether fresh graft is superior to grafts preserved using the three other methods. Good results have been reported in patients transplanted with fresh grafts. Verdonk70 has found intact grafts of all 40 transplanted meniscal allografts using follow-up magnetic resonance imaging (MRI). One of the benefits of using a fresh graft includes viable cells present in the graft, which may be important in cartilage transplantation. However, Jackson and associates33 have shown that donor DNA in the transplanted meniscus was entirely replaced by host DNA at 4-week follow-up. The importance of viable cells in the meniscal transplant remains to be determined.
Cryopreservation has provided a useful alternative to fresh grafts in terms of safety and function. The graft is thawed in the operating room just prior to surgery. Arnoczky and associates5 have shown that the material properties of transplanted cryopreserved allografts in dogs are similar to those of normal menisci after 6 months. Cell viability ranging between 5% and 54% has been described in the literature. Gelber and coworkers29 have shown that meniscal cryopreservation does not alter the meniscal ultrastructure or the biomechanical properties. Fabbriciani and colleagues22 reported no difference in appearance or healing between meniscal allografts that were cryopreserved and deep-frozen. The expense and difficulty of this process, along with the uncertainty of donor cell viability, have decreased the popularity of this preservation process.
Fresh-frozen meniscal preservation is a simpler and less expensive method than cryopreservation. Deep freezing has been shown to destroy viable cells of connective tissue and to denature histocompatibility antigens, making frozen allografts less likely to provoke an immune response.9 Milachowski and colleagues45 have reported shrinkage in one of five deep-frozen grafts by MRI. At 48 weeks, deep-frozen grafts showed little revascularization or remodeling, but good preservation.
Lyophilization has been shown to destroy viable cells of connective tissue and to denature histocompatibility antigens.9 Milachowski and associates45 have reported shrinkage in 9 of 10 lyophilized and gamma-sterilized allografts. All lyophilized allografts were remodeled and completely revascularized at 48 weeks. The same group of patients was reviewed 14 years postoperatively.77 Second-look arthroscopy and MRI showed shrinkage of the lyophilized grafts. Gelber and associates29 have evaluated the collagen meniscal architecture of excised meniscal transplants at the time of total knee replacement. They found the fibrils in frozen meniscal allografts to be of smaller diameter and in more disarray compared with nonfrozen controls. These findings may explain the shrinking associated with this type of preservation.
Indications and Contraindications for Meniscal Transplantation
The indications for meniscal transplantation continue to evolve as long-term clinical results become available. Currently, meniscal transplantation is a salvage procedure for patients with meniscus-deficient knees. The goal of meniscal transplantation is to reduce pain and prevent progression of arthritis. Consideration of meniscal transplantation should only be entertained when nonsurgical measures to control pain have been exhausted, including activity modification, anti-inflammatories, injections, and unloading bracing. With concurrent ACL reconstruction, earlier meniscal transplantation may improve clinical outcomes.30,41,61 Veltri and coworkers69 have defined the ideal meniscal transplantation candidate to be skeletally mature, younger than 50 years, post-total or subtotal meniscectomy, with a ligamentously stable knee or planned stabilization, with no evidence of malalignment, and with minimal arthrosis. Although they used 50 years old as the upper age limit in patients with minimal arthritis, this is controversial because physiologic age is more accurate than absolute age.49
Outerbridge published a classification of cartilage lesions associated with chondromalacia patellae in 1961 (Table 35-2).49 This classification system has been modified to describe articular cartilage lesions seen at the time of arthroscopy. It can be used to classify the patient’s arthrosis to determine whether he or she is a candidate for meniscal transplantation. Garret,28 Noyes and coworkers,46 and Cole and colleagues16 have demonstrated improved results in meniscal transplantation in patients whose degenerative changes are Outerbridge grade I or II. Patients with Outerbridge grade III or IV degeneration levels had less predictable outcomes. No studies were able to define whether the poor results found in patients with advanced degenerative changes was caused by the degenerative disease itself or associated knee malalignment.
Table 35-2 Outerbridge Classification
Grade | Features |
---|---|
Original Description* | |
I | Softening and swelling of the cartilage |
II | Fragmentation and fissuring <![]() |
III | Fragmentation and fissuring >![]() |
IV | Erosion of cartilage down to bone |
Currently Accepted Description | |
I | Softening |
II | Partial-thickness fissures |
III | Full-thickness fissures |
IV | Exposed subchondral bone |
* Initial description was based on macroscopic changes of the patella. This system was modified to describe arthroscopic changes in the articular cartilage.
Another critical factor in determining a successful candidate is the mechanical alignment of the knee. Malalignment is defined as valgus or varus asymmetry between 2 and 4 degrees compared with the contralateral knee, or the mechanical axis falling into the affected meniscus-deficient compartment on weight-bearing films. Meniscal transplantation in a malaligned knee causes abnormal pressure on the meniscal allograft, resulting in impaired vascularization, degeneration, and failure of the graft.55 In cases of malalignment, meniscal transplantation is contraindicated until a concurrent corrective osteotomy is performed. Cameron and Saha12 have reported 85% good results in patients undergoing meniscal transplantation and corrective osteotomy. It remains to be determined whether the osteotomy or the meniscal transplantation led to the clinical improvement.
Meniscal transplantation can be considered for patients with concomitant ACL instability. Garret28 has reported significantly improved KT-1000 arthrometer results for ACL reconstruction when performed in combination with medial meniscal allograft transplantation compared with a group of patients who underwent ACL reconstruction only, with persistent medial meniscal deficiency.
The primary absolute contraindication to meniscal transplantation is advanced arthrosis of the meniscus-deficient knee. Other contraindications to meniscal transplantation include previous infection and inflammatory arthritis. Obesity is a relative contraindication to surgery because of the increased force across the joint, leading to an increased failure rate. The indications and contraindications for meniscal transplantation will continue to evolve as long-term results become available. A summary of indications and contraindications is listed in Table 35-3.
Table 35-3 Contraindications to Meniscal Transplantation
Contraindication | Features |
---|---|
Absolute | Advanced arthritisJoint space narrowingFemoral flatteningOsteophytesOuterbridge grades III and IV changesJoint incongruity |
Relative | MalalignmentFocal chondral defectsLigament instabilityObesityRheumatoid arthritisGoutImmune compromiseMetabolic diseaseInfectionLack of commitment to postoperative restrictions |
Preoperative Considerations
Graft Sizing
Good clinical outcomes following meniscal transplantation rely on selection of an appropriately sized meniscal allograft. The tolerance of size mismatch in knees undergoing meniscal transplantation is not known. Although too small a meniscal allograft does lead to uneven contact forces, more meniscus is not always better. The meniscus must be properly sized for optimum reduction of contact pressures. Dienst and associates21 have shown that oversized lateral meniscal allografts lead to greater forces across the articular cartilage, whereas undersized allografts result in normal forces across the articular cartilage but greater forces across the meniscus. They concluded that grafts within 10% of the native meniscus were able to reproduce contact mechanics close to the intact state. Huang and coworkers32 have found increased contact pressures when allografts did not match the native menisci. The greatest predictor of differences in contact pressure was the difference in the width of the menisci. It was concluded that protocols used to select allografts should focus on cross-sectional parameters to match the native meniscus.
Sizing of allografts is done using plain radiography, computed tomography (CT), or MRI or by making direct measurements. The use of the contralateral knee in patients with prior meniscectomy has been described to help sizing. However, Johnson and colleagues38 have shown that there is variability in meniscal size between opposite knees. The most commonly used method for sizing today is plain radiographs. Pollard and associates52 have found that the meniscal width equals the distance (coronal) from the peak of the tibial eminence to the periphery of the tibial metaphysis on anteroposterior films. Medial meniscal length is 80% and lateral meniscal length is 70% of the measured sagittal length of the tibial plateau on the lateral radiograph. Measurement error averaged 7.8% by these parameters. Axial CT scans can size the meniscus without concern for rotation or magnification. However, the patient must endure a much larger load of radiation. Shaffer and coworkers63 have compared the accuracy of plain x-rays and MRI for preoperative sizing of meniscal allografts. Overall, MRI was slightly more accurate than plain radiography; however, only 35% of the menisci measured with MRI were within 2 mm of the actual meniscal size. Carpenter and colleagues13 have compared the accuracy of MRI with both plain radiograph and CT in estimating the size of the meniscal allograft. MRI was found to be the most accurate for meniscal height but consistently underestimated the anteroposterior and mediolateral sizes. Prodromos and associates53 have compared MRI with plain radiography in estimating the appropriately sized meniscal allograft. They concluded that human knee menisci are bilaterally symmetric in size, and direct MRI measurement of the contralateral intact meniscus predicts actual meniscal size better than estimation of size indirectly from measurement of the tibial plateau on which it is located. They proposed contralateral MRI meniscal measurement as a new gold standard to size menisci before transplantation. Although plain radiographs are currently the gold standard, it appears that MRI techniques are improving and will eventually be the study of choice for determining meniscal allograft size.
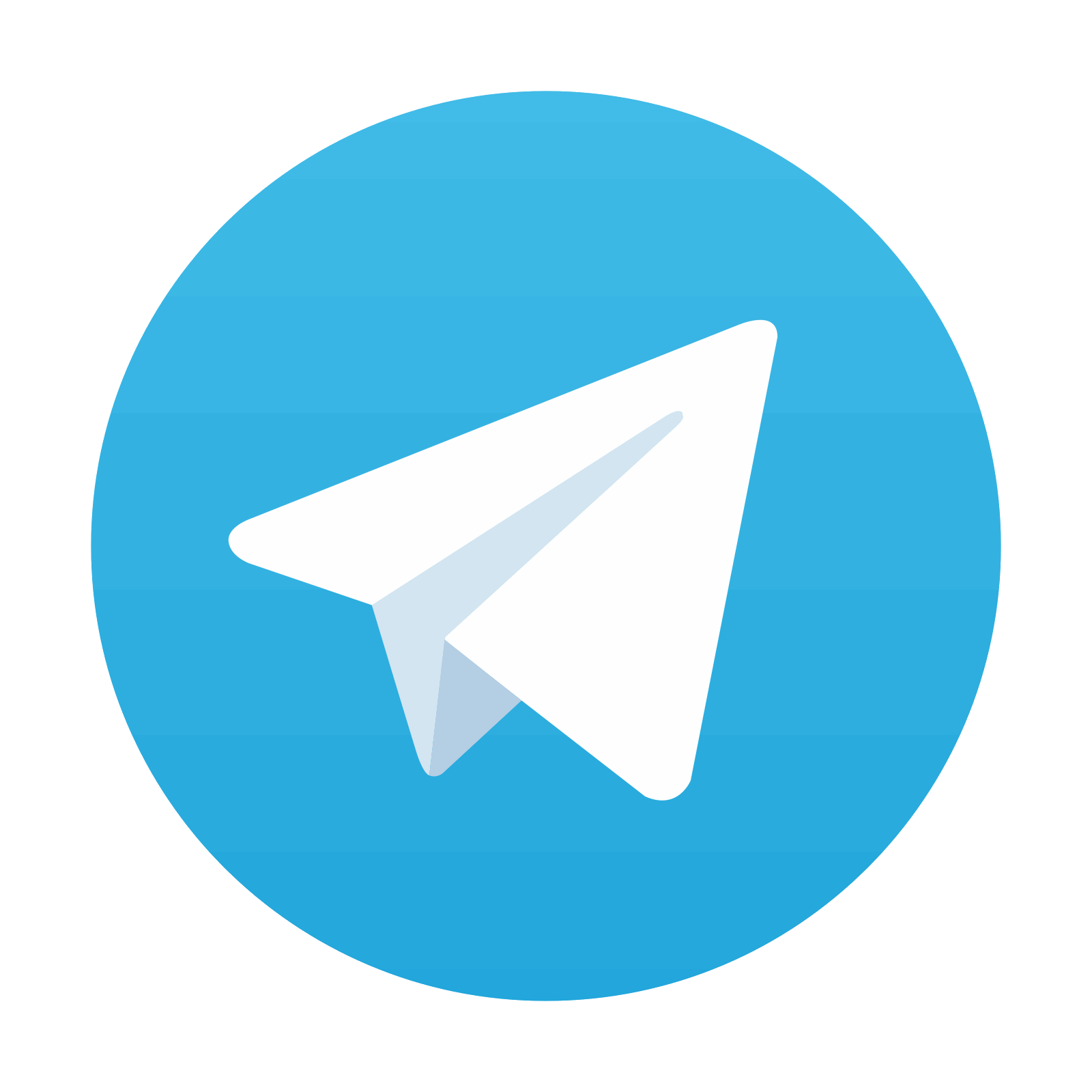
Stay updated, free articles. Join our Telegram channel

Full access? Get Clinical Tree
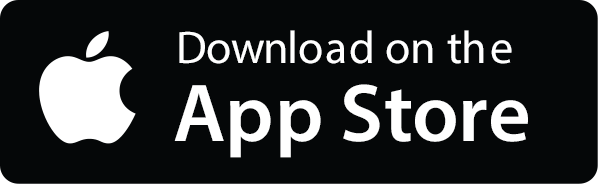
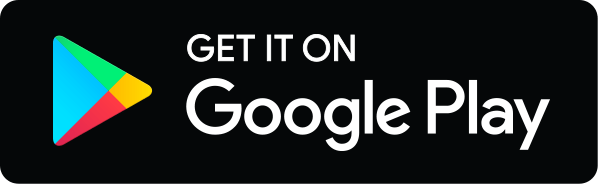