Thomas P. Schmalzried
Mechanisms of Total Hip Arthroplasty Failure
Failure is the opportunity to begin again more intelligently.
—Henry Ford
INTRODUCTION
Total hip arthroplasty (THA) is widely recognized as one of the most cost-effective interventions in medicine today. The impact that these procedures have on quality of life has been well documented.1 The definition of failure of a prosthetic joint is a function of the expectations and the limitations of the technology. A distinction should be made between failure of the arthroplasty, a material or device failure, and the limitations of the technology. Reoperation for any reason has generally been considered the clinical hallmark of failure. By this definition, a prosthetic hip arthroplasty that was revised after years of satisfactory function would be classified a failure. Such cases, however, represent the limitations of the technology.
As advances in technology reduce the occurrence of aseptic loosening, wear, and osteolysis (inflammatory bone resorption), less frequent causes of reoperation such as infection, recurrent instability, modularity, and material failure will become relatively prominent.
REASONS FOR REOPERATION
The Swedish National Hip Arthroplasty Register (2003)2 reported that the total number of revision THAs (involving exchange or extraction of one or both components or any part of the prosthesis) from 1979 to 2003 in Sweden was 21,367, of which 17,881 (84%) were the first revision. The indications for revision were aseptic loosening in 73.9%, deep infection in 7.9%, dislocation in 7.5%, periprosthetic fracture in 5.7%, technical error in 2.5%, implant fracture in 1.5%, miscellaneous 0.6%, and pain in 0.3%. In the United States, Clohisy et al.3 reported on 430 revisions between 1996 and 2003. Reoperation was performed for aseptic loosening in 55% of cases, 14% for instability, 13% for osteolysis with well-fixed implants, 7% for infection, 5% for periprosthetic fracture, 1% for soft tissue impingement, 1% for loose (recalled) implants, and 1% for implant fracture. Paprosky et al.4 reported on 270 revisions of cementless components. The indications for revision of 157 acetabular components were osteolysis or loosening in 71%, dislocation in 16%, component migration in 6%, pain in 2.5%, infection in 2.5%, and malposition in 2%. On the femoral side, 113 cementless components were revised for osteolysis or loosening in 71%, pain in 11%, dislocation in 9%, malposition in 5%, and infection in 3%.
ASEPTIC LOOSENING
A continuous interplay of multiple factors occurs in complex prosthetic-biological systems such as total hip arthroplasties, making it difficult to separate loosening that occurs primarily as a result of mechanical forces from loosening that occurs as a direct result of an adverse biologic response.
Cemented Acetabulum Autopsy studies of cemented all-polyethylene acetabular reconstructions indicate that the earliest evidence of loosening is resorption of the bone immediately adjacent to the cement mantle circumferentially at the intra-articular margin, in continuity with the joint pseudocapsule. An expanding front of bone resorption then proceeds from the intra-articular surface toward the dome of the acetabular reconstruction. Histological analyses have revealed that progressive bone resorption is caused by a foreign-body macrophage inflammatory reaction fueled by subcellular particles of polyethylene invading the cement-bone interface. Evidence in support of a mechanical basis for the initiation of loosening was lacking. Cement fragmentation appears to be a result of loosening and not the initiating event. However, there may be mechanical factors, such as neck-socket impingement, that contribute to loosening in specific cases. Once stability is lost, additional motion can only be detrimental.5 There is substantial evidence indicating the central role of volumetric wear of polyethylene in cemented acetabular component loosening. Experience has demonstrated a higher incidence of loosening with larger femoral head diameters, which in turn have correlated with higher volumetric polyethylene wear. Volumetric wear is correlated to the number of polyethylene particles generated.6
An increased incidence of cemented acetabular component loosening has been reported with metal-backed components.7 This has also been related directly to a significantly increased rate of polyethylene wear.8 Metal backing introduces at least one additional interface (polyethylene-metal). In addition, other variables potentially contribute to increased wear in these cases including reduction in the thickness of the polyethylene by the addition of the metal backing, increased polyethylene stress from the metal backing, and an increase in the amount of third-body wear.
The fundamental variables affecting the long-term maintenance of the cemented acetabular fixation include (a) the integrity of the initial cement-bone interface (i) for mechanical stability and (ii) as a barrier to joint fluid and particulate debris and (b) the volumetric wear rate (the rate of particle generation) by the bearing surfaces. This illustrates the significance of the interplay between multiple factors including implant materials and designs, surgical techniques of implantation, and the amount of use of the implant by the patient in determining outcome.
Cemented Femoral Components Autopsy studies of the femoral side indicate that the earliest evidence of loosening in many patients is separation of the stem from the cement, the so-called debonding, which can be associated with cement fractures arising from pores and/or defects in the cement mantle.9–11 Mechanical testing of autopsy specimens indicates low axial micromotion with consistently greater rotational displacements. This highlights the importance of the high torsion stresses that occur during stair climbing and rising from a seated position,12,13 which force the femoral component into a more retroverted position.11 The occurrence and consequences of such stem-cement debonding are influenced by stem design and surface finish as well as surgical technique and patient factors.14,15 Collarless, tapered stems with a polished surface demonstrate high survival without any bond between the stem and the cement. Such components are designed to subside slightly within the cement mantle and transfer more uniform compressive stresses and less tensile stresses to the cement mantle.16 For at least one implant design, the same stem with a higher surface roughness had higher loosening and osteolysis rates than its counterpart with a lower surface roughness.17 Stems with a roughened surface and higher stemcement interface bond strength are more likely to induce localized tensile failures (cracks) in the cement mantle.18 If such a stem debonds, relative motion between the rough metallic surface and the cement mantle generates numerous particles of metal and cement. These particles can gain access to diaphyseal bone through cracks or other defects in the cement mantle, resulting in localized osteolysis and clinical failure.15
Regardless of implantation duration, intimate contact of bone with cement has been a consistent finding in retrieval studies. As originally described by Charnley19 a second medullary canal forms between the endosteal surface and the outer cortex. Endosteal bone remodels into circumferential lamellae, which become osseointegrated with the cement mantle, forming a thin inner cortex. The inner and outer cortices are connected by a series of radial struts of trabecular bone. The second medullary canal that forms may appear as a radiolucency between the cement mantle and the outer cortex on radiographic analysis.20 Thus, the normal age-related process of endosteal remodeling does occur, but it is modified by the presence of the femoral implant and this remodeling pattern does not result in loosening.
In contrast to diaphyseal cement-bone radiolucencies, the etiology of proximal femoral cement-bone interface radiolucencies is distinctly different. Soft tissue interposition commonly occurs in the proximal 1 to 2 cm of the femoral cement-bone interface.21,22 This tissue is similar in gross and microscopic appearance to the tissue of the joint pseudocapsule and characterized by numerous plump macrophages laden with small polyethylene wear particles. Proximal femoral bone resorption, commonly seen on standard anteroposterior radiographs involving the medial femoral cortex (the so-called calcar resorption), is essentially circumferential. Thus, a similar inflammatory process of periprosthetic bone resorption occurs in a roughly centrifugal fashion on both sides of the joint.21 The effect of the process on component fixation is different, however, on the femoral as compared to the acetabular side. In the hemispherical acetabulum, the majority of the surface area available for fixation to bone is near the intra-articular margin, whereas the more cylindrical shape of the femur distributes the fixation area over a greater distance from the articulation. Thus, 2 cm of circumferential bone resorption at the proximal femoral cement-bone interface does not generally result in femoral implant loosening because of the extent of the femoral cement-bone interface that remains intact. If the 2 cm of the acetabular cement-bone interface adjacent to the hip articulation is disrupted by bone resorption, then the great majority of the acetabular interface surface area will be disrupted and mechanical stability will be compromised.
Femoral components with inadequate initial cement fixation, or a grossly deficient cement mantle, progressively loosen at the cement-bone interface. Interfacial motion generates particles of cement, bone, and metal, which can fuel osteolysis. Polyethylene wear particles can migrate along such disrupted interfacial planes and further contribute to osteolysis.
Cementless Acetabular Components Radiographic and retrieval studies indicate that a similar process of marginal bone resorption occurs with cementless acetabular components.21,23–27 In contrast to cemented acetabular components, however, such inflammatory periprosthetic bone resorption usually does not result in cementless acetabular component loosening. A multicenter review of 1,081 primary total hip arthroplasties using a cementless acetabular component with a mean follow-up of 81 months reported aseptic loosening in 0.6% (7/1,081) and pelvic osteolysis in 2.3% (25/1,081). Revision for polyethylene wear and pelvic osteolysis was performed in 1.7% (18/1,081).28 A single institution’s experience with 4,289 primary total hip arthroplasties using cementless hemispheric porous-coated components indicates 15-year survivorship rates, using revision for cup loosening as an end point, above 90% for all designs. Acetabular component revision was performed for aseptic loosening in 0.35% (15/4,289), while reoperation for polyethylene wear and pelvic osteolysis was performed in 1.8% (77/4,289).29
Cementless acetabular components are characterized by bone loss that progresses away from the interface into the soft cancellous bone of the pelvis resulting in localized osteolysis.27,30 This bone loss can occur along access channels around the periphery of the implant or provided by screens and screw holes in through the cap. A consequence of this phenomenon is that substantial bone resorption can occur around rigidly fixed and clinically asymptomatic components.27 Such extensive and progressive pelvic bone resorption can necessitate reoperation even though the component is well fixed.31,32
Cementless Femoral Components Autopsy retrieval analyses of well-functioning, cementless, cobalt-chrome, porous-ingrowth femoral components have been reported. Similar to the neocortex that forms around cemented femoral components, a layer of bone grows onto or into the bioactive surface and is connected by radial struts to the endosteum.33 For implants with bone ingrowth, the maximum relative motion between the cortex and the porous coating was 40 mm. The amount of tip motion was dependent on the extent of the porous coating; maximum tip motion for proximally porous-coated implants was 210 µm. As seen with cemented femoral components, stress shielding was associated with bone remodeling changes that resulted in regional reductions in bone mineral content ranging from 7% to 78%. The greatest decrease in bone mineral content consistently occurs adjacent to the proximal third of the femur.34,35 Such stress shielding has not been a cause of clinical failure.36
Similar to cemented femoral components, inflammatory bone resorption can occur in the proximal femur.21 The ramifications of this process for the long-term fixation of cementless femoral components are dependent on the amount and distribution of porous coating and ingrowth. In a component with limited proximal porous coating, inflammatory bone resorption in the proximal femur may play a role in the loosening of a component that had previously been rigidly fixed by bone ingrowth.37 In a component with extensive porous coating, this same process has not resulted in loosening. However, in such cases, osteolytic destruction of the greater and/or lesser trochanter can result in fracture (Fig. 4-1).38
FIGURE 4-1. This female patient has had these total hip replacements (polyethylene sterilized by gamma-in-air radiation) for about 9 years. The reconstructions functioned very well and she regularly participated in aerobics classes. Note the eccentric position of the heads within the acetabular components and the extensive periprosthetic osteolysis with fracture of the right greater trochanter and destruction of both lesser trochanters. All of the cementless components are well fixed.
Compared to cemented femoral components, localized endosteal bone resorption (osteolysis) occurs earlier and more frequently in the femoral diaphysis adjacent to cementless femoral components with noncircumferential proximal porous coating.21,39 This occurs because joint fluid and wear particles have easy access to the diaphyseal endosteum along access channels when lack of bone ingrowth or ongrowth occurs between patches of porous coating. Diaphyseal osteolysis is rarely observed in implants with a proximal circumferential bioactive surface and does not occur with stable, extensively porous-coated stems.
In contrast to theoretical concerns, frictional torque from the intended motion of the femoral-acetabular bearing has not been demonstrated to play an important role in either femoral or acetabular component loosening.40
POLYETHYLENE WEAR AND OSTEOLYSIS
It is important to distinguish among the fundamental wear mechanisms, adhesion, abrasion, and fatigue; the changes in the appearance (morphology) of the bearing surfaces, referred to as wear damage; and the conditions under which the prosthesis was functioning when the wear occurred, which have been termed the wear modes.41
Mode 1 wear results from the motion occurring between the two primary bearing surfaces, as intended, such as the prosthetic femoral head moving against the polyethylene acetabular bearing surface. Mode 2 refers to the condition of a primary bearing surface moving against a secondary surface, which is not intended. An example of this wear mode would be when a femoral component penetrates through a modular polyethylene bearing and rubs against a secondary surface, such as the metallic acetabular shell.41–47 Mode 3 refers to the condition of the primary surfaces moving against each other, but with third-body particles interposed. In Mode 3, the contaminant particles directly abrade one or both of the primary bearing surfaces. This is known as three-body abrasion or three-body wear. The primary bearing surfaces may be transiently or permanently roughened by this interaction.
Mode 4 refers to two secondary (nonprimary) surfaces rubbing together. Examples of Mode 4 wear include impingement of the prosthetic femoral neck on the rim of the acetabular component48; wear due to stem-cement or bone-cement interface motion or from relative motion of a porous coating, or other metallic surface, against bone49–54; relative motion of the external surface of a modular polyethylene component against the metal support, so-called back-side wear; fretting between a metallic substrate and a fixation screw44,55–57; fretting and corrosion of modular taper connections58; as well as extra-articular sources.35,59,60 The particles produced may be composed of bone, polymethylmethacrylate, metal alloys, metallic corrosion products, or hydroxyapatite.49,58,61,62 Wear particles produced by Mode 4 wear can induce an inflammatory reaction as well as be transported to the bearing surfaces and induce three-body wear (Mode 3).58, 59, 63,
Mode 1 wear is a consequence of the intended motion of the joint prosthesis, whereas Modes 2, 3, and 4 are generally unintended. The operating conditions in vivo are variable and several types of wear can occur simultaneously. There are many variables that influence polyethylene wear in vivo and, consequently, polyethylene wear rates are highly variable. Although wearing through of a polyethylene bearing can occur, revision is more commonly necessitated by progressive osteolysis that occurs in association with wear.
In a prosthetic joint, polyethylene wear particles are dispersed in the fluid around the joint. Conceptually, the effective joint space includes all periprosthetic regions that are accessible to joint fluid and, thus, accessible to wear particles.21 The limits of the effective joint space are determined by how intimate the contact is between the prosthesis and the bone and how this contact varies within a given reconstruction. This variability determines the access routes for joint fluid and particles, to and along various interfaces, into bone, and through soft tissues as well. Joint fluid flows according to pressure gradients, following the path of least resistance. Patterns of joint fluid flow influence the shape and extent of osteolysis. The local concentration of particles is a factor in the local inflammatory reaction and, hence, the degree of bone resorption in that location. As bone is resorbed, a larger space is produced, encouraging preferential flow of joint fluid and wear particles into that location, which fuels further bone resorption in that area, leading to an expansile lesion. If the rate of particle production is low, or if joint fluid is more evenly distributed, there will be a slower rate of bone resorption, accompanied by a fibroblastic response, resulting in a more linear pattern of bone loss that often follows the contours of the implant.
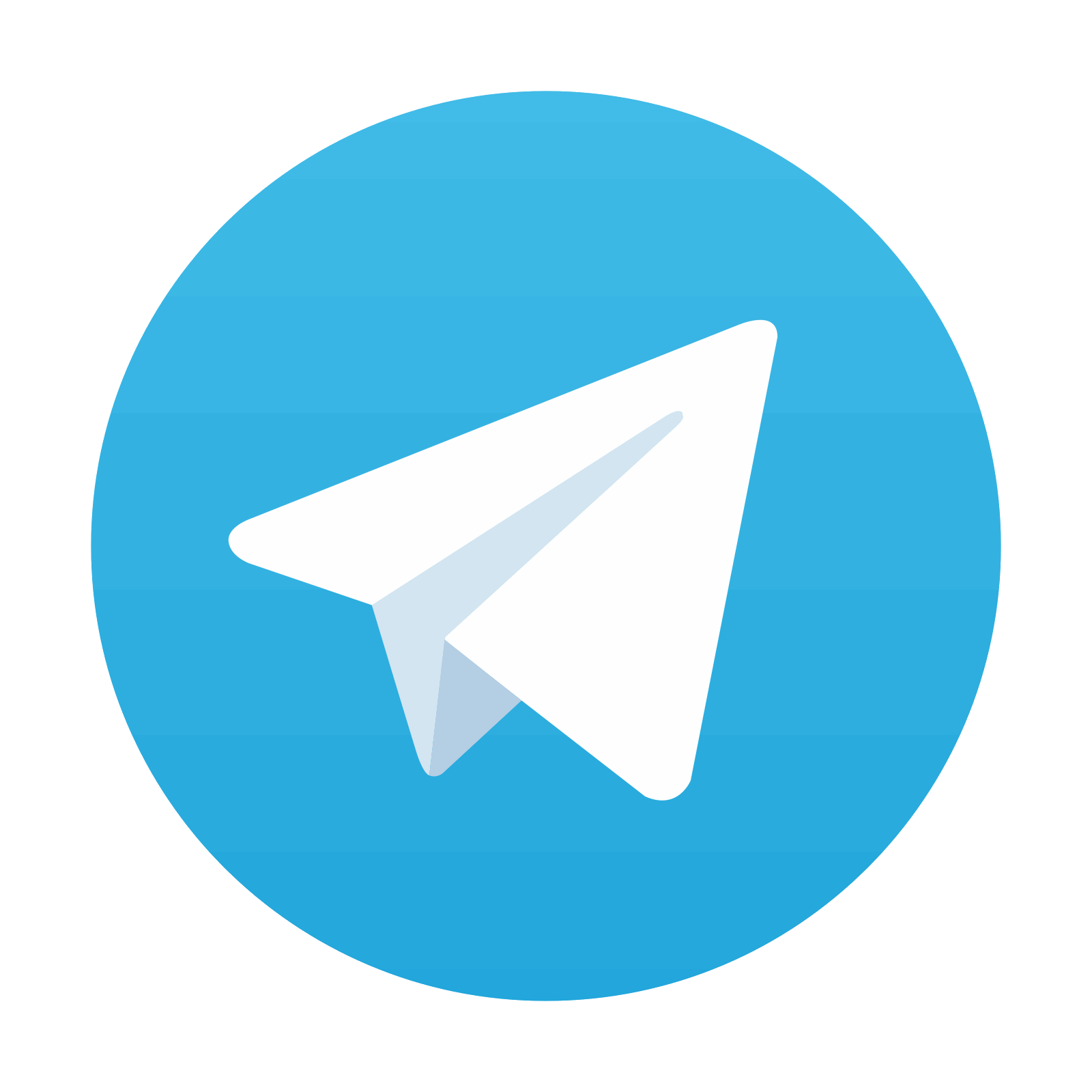
Stay updated, free articles. Join our Telegram channel

Full access? Get Clinical Tree
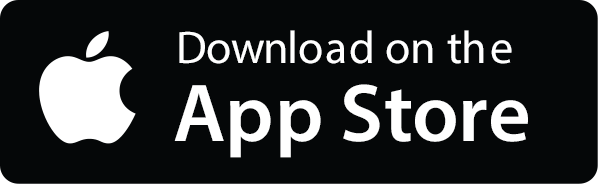
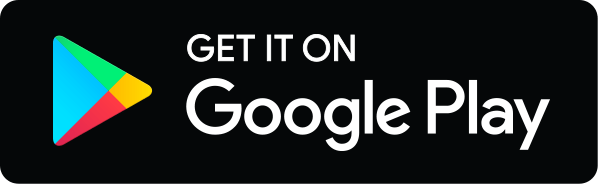