Fig. 5.1
Intraoperative photograph demonstrating use of a femoral rotational alignment guide applied to the distal femur and aligned along the posterior condylar axis to assure three degrees of external rotation relative to the posterior condylar axis
Despite the apparent simplicity of choosing instrumentation based on the posterior condylar axis to determine femoral component rotation, this technique has several potential disadvantages. The anatomy of the distal femur varies, and the decision to externally rotate the cutting guides three to four degrees in relation to the posterior condylar axis was derived from mean data. Poilvache et al. [9] measured the angles between the tangent line of the posterior condylar surfaces, the anterior-posterior axis described by Whiteside [10], and the transepicondylar line in 100 knees undergoing TKR. The range of the angle between the transepicondylar line and the posterior condylar line (condylar twist angle) was between −1° and 7° external rotation (Fig. 5.2a, b).
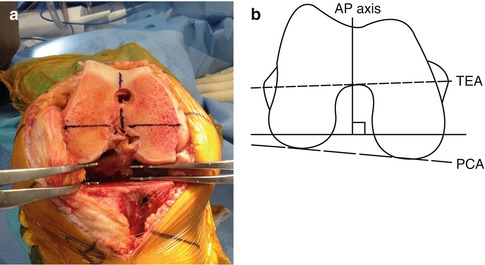
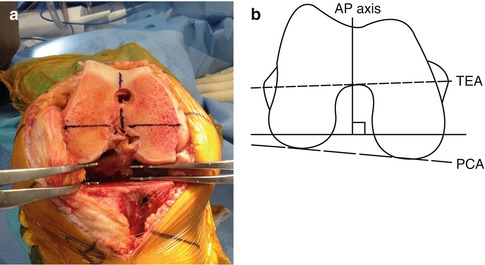
Fig. 5.2
Diagram (a) and intraoperative photograph (b) demonstrating the transepicondylar (TEA), anterior-posterior (AP), and posterior condylar (PCA) axes
The angle formed by the epicondylar and posterior condylar lines presents a variation of −1° to 7°. The usage of a fixed external rotation of 3° is questionable.
Mantas et al. [11] also noted in normal cadaveric femurs that the transepicondylar axis varied from 0.1° to 9.7° externally rotated compared to the posterior condylar axis, with an average external rotation of five degrees. Due to the wide anatomic variation in the relationship of the posterior condylar axis to the transepicondylar axis, a significant percentage of patients would incur greater than two degrees of malrotation (femoral component internal rotation) relative to the transepicondylar axis if the femoral component were automatically rotated three degrees with regard to the posterior condyles [8]. For example, if a patient’s anatomical posterior condylar twist angle is eight degrees and instrumentation is used which places the femoral component in three degrees of external rotation relative to the posterior condylar line, the femoral component will actually be internally rotated five degrees relative to the transepicondylar axis [17].
Use of the posterior condylar axis may also be inaccurate in knees with arthritic deformities and is unavailable in knees requiring revision TKR [18]. Hypoplasia or erosion of the posterior aspect of the lateral femoral condyle in valgus knee deformity will lead to erroneous femoral component internal rotation if the posterior condylar axis is used as the primary determinate of femoral component rotation (Fig. 5.3) [19]. Conversely, the posterior aspect of the medial femoral condyle is often eroded in varus knees with chronic insufficiency of the anterior cruciate ligament which can lead to placement of the femoral component in excessive external rotation if the posterior condylar axis is used. Schurr et al. [12] evaluated the flexion gap stability in a series of 100 TKR implanted using computer navigation. They noted that a rectangular flexion gap would have been obtained in only 51 % of the cases if the posterior condylar axis had been utilized to determine femoral component rotation. Fehring et al. [20] compared the tensioned gap balancing technique with the measured resection technique using fixed bony landmarks such as the PCA for rotational positioning. They observed femoral component rotational errors of at least three degrees occurred in 45 % of patients.
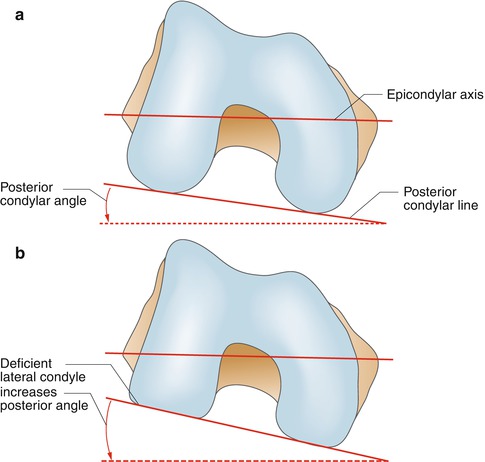
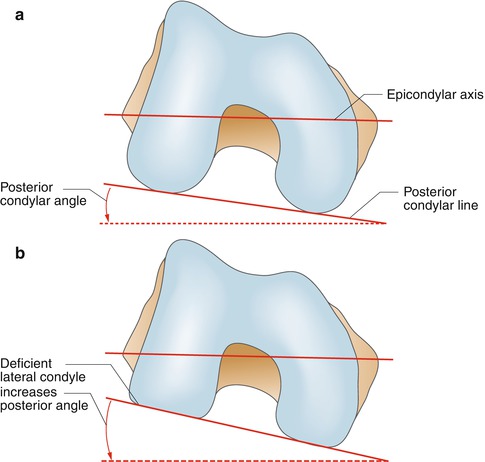
Fig. 5.3
Diagram demonstrating erosion of the posterior aspect of the lateral femoral condyle which will result in erroneous femoral component internal rotation if the posterior condylar axis is utilized to determine femoral component rotation. (a) Without erosion of posterior aspect of lateral femoral condyle. (b) With erosion of posterior aspect of lateral femoral condyle
5.1.2 Anterior-Posterior Axis
The anterior-posterior (AP) axis was first described by Leo Whiteside. The axis is constructed by a line connecting the deepest point of the trochlea with the midpoint of the posterior aspect of the intercondylar notch (Fig. 5.2a, b). Constructing the AP axis relies on normal anatomy of the trochlear groove and intercondylar notch of the distal femur [21]. Arima et al. [22] showed that patellofemoral problems were significantly reduced in knees in which the femoral component was positioned perpendicular to the AP axis as compared with knees in which the femoral component was placed parallel to the posterior condylar axis. Unlike the posterior condylar axis, the AP axis can also be utilized in cases of posterior condylar bone erosion or hypoplasia. Other researchers, however, have noted a wide range of error when using the AP axis as the sole determinant of femoral component rotation. Poilvache et al. [9] found that severe trochlear dysplasia caused excessive external rotation of the femoral component in their study of 100 arthritic knees. Nagamine et al. [23] studied CT scans of 84 knees and found that in normal knees, the line perpendicular to the AP axis was externally rotated 3.5° relative to the posterior condylar axis. They noted that the line perpendicular to the AP axis was rotated externally more in the medial femorotibial osteoarthritis knees compared to that in the normal knees, indicating that the patellar groove was directed distally and medially. They felt that in patients with osteoarthritic knees, there may be excessive external rotation of the femoral component when the AP axis is used to determine rotational alignment leading to coronal plane instability in flexion. Yau et al. [24] also found a 32° range of error (15° external rotation to 17° of internal rotation) using the AP axis to determine femoral component rotation.
5.1.3 Transepicondylar Axis
The transepicondylar axis (TEA) is a line connecting the prominence of the lateral epicondyle to the medial epicondylar ridge (clinical TEA) or the medial epicondylar sulcus (surgical TEA) (Fig. 5.2a, b) [7, 25]. This line approximates the flexion-extension axis of the knee and corresponds to the femoral collateral ligamentous origin [25]. Berger et al. [7] reported that the surgical TEA is a useful landmark in determining the native neutral rotational orientation of the femoral component. Placing the femoral component parallel to the TEA enhances central patellofemoral tracking and has been shown to improve femorotibial kinematics [26–29]. Insall et al. [29] demonstrated better coronal plane stability (lower incidence and magnitude of femoral condylar lift-off) if the femoral component was placed parallel to the TEA axis in a kinematic analysis. Olcott et al. [28] also found that the TEA assisted in obtaining a rectangular flexion gap (90 % using the TEA, 83 % using the AP axis, and 70 % using the posterior condylar axis). It also can be referenced in knees with condylar hypoplasia and erosion as well as in revision TKR.
Unfortunately, studies suggest that surgeons’ ability to accurately and reproducibly identify the TEA is poor. Both the medial and lateral epicondyles can be difficult to reproducibly locate intraoperatively [8, 30–32]. Jerosch et al. [31] demonstrated that when surgeons were invited to mark the epicondyles under experimental conditions, the range of position chosen by the surgeons on the medial side varied 22.3 mm versus a 13.8 mm variance in identification of the lateral epicondyle. Kinzel et al. [32] evaluated the accuracy of epicondylar identification in a series of 74 TKR. Pins were placed in the femoral epicondyles intraoperatively. Postoperative CT scans demonstrated that the epicondyles were correctly identified to within ±3° in only 75 % of the cases. There was a wide range of error (6° of external rotation to 11° of internal rotational error). They concluded that the TEA was an unreliable landmark and should not be relied upon as the sole determinant of femoral component rotation. Yau et al. [24] noted that a range of error greater than five degrees occurred 56 % of the time when the TEA was used to determine femoral component rotation. This was perhaps related to the wide range of error in intraoperative surgeon identification of the femoral epicondyles (28° error range; 11° external rotation to 17° of internal rotation). Siston et al. [33], in a cadaveric study using an imageless computer navigation system, evaluated use of the posterior condylar, AP, and transepicondylar axes to determine femoral component rotation. They demonstrated only 17 % of the actual registered landmarks fell within five degrees of the true epicondylar axis. All 11 surgeons participating in the study registered landmarks that tended to overly externally rotate the femoral component relative to the true epicondylar axis. Benjamin et al. [34] found the posterior condylar axis most frequently corresponded to the rotation alignment of the implanted femoral component, falling within ±1° in 62 % of the patients. In contrast, the registered epicondylar axis was only accurate to within one degree 34 % of the time, and registration of the AP axis was accurate only 26 % of the time.
In summary, substantial error has been demonstrated in numerous reports when measured resection bone landmarks are used to determine femoral component rotation, especially if a single bone landmark is utilized. If a measured resection methodology is used in TKR, utilization of all available bone landmarks is wise.
5.2 Gap Balancing Technique
With the gap balancing technique, ligament releases are performed to correct fixed deformities and bring the limb into approximate alignment prior to determination of femoral component rotation [35]. Either the flexion or extension gap can be balanced first. This section will present a technique for balancing the extension gap initially and an alternative technique for balancing the flexion gap first.
With the gap balancing technique, ligament releases are performed to correct fixed deformities and bring the limb into approximate alignment prior to determination of femoral component rotation [35]. Either the flexion or extension gap can be balanced first.
5.2.1 Gap Balancing: Extension Gap First Technique
The authors prefer to balance the extension gap prior to the flexion gap. The distal femur is initially resected using an intramedullary guide followed by resection of the proximal tibia at a 90° angle to the long axis of the tibia using an extramedullary guide. A spacer block is then placed into the extension gap to determine gap symmetry, soft tissue balance, and lower extremity alignment versus the mechanical axis (Fig. 5.4). All osteophytes, including posterior femoral and tibial osteophytes, must be removed at this point due to their tensioning effect on adjacent ligamentous structures. Waiting to remove posterior osteophytes until after the anterior and posterior femoral cuts have been made can cause flexion gap asymmetry because these posterior osteophytes tension the soft tissues and thereby impact the determination of femoral rotation. Access to posterior femoral osteophytes before the posterior femoral condyles have been resected can be difficult due to excessive tension of the flexion gap. A useful technique to gain access is to perform a limited, four millimeter resection of the one or both posterior femoral condyles with the knee flexed 90° (Fig. 5.5a). A laminar spreader is then placed into the flexion gap to provide distraction and access to the posterior compartment. The posterior femoral osteophytes are then accessed and removed using a curved osteotome (Fig. 5.5b). If malalignment or extension gap imbalance persists, tight ligamentous structures are then released with the knee in extension until the alignment of the knee is neutral and soft tissue balance is symmetric [36].
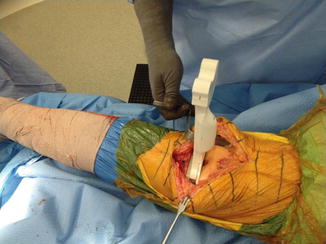
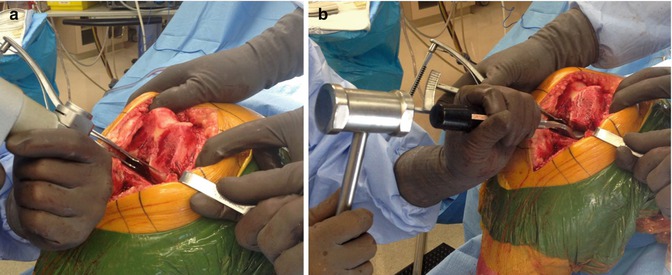
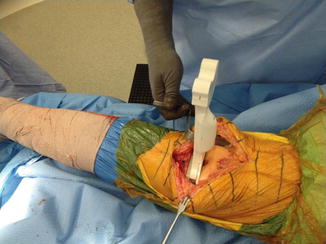
Fig. 5.4
Intraoperative photograph demonstrating assessment of lower extremity alignment and extension gap balance using a spacer block
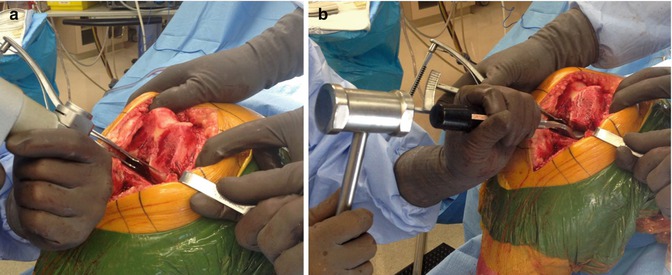
Fig. 5.5
(a) Intraoperative photograph demonstrating resection of 4 mm of the posterior aspect of the medial femoral condyle to gain access to posterior compartment osteophytes. (b) Intraoperative photograph demonstrating removal of posterior femoral osteophytes using a curved osteotome
The flexion gap is then created with a goal of duplicating the dimensions of the extension gap. The knee is placed in 90° of flexion and each collateral ligament is equally tensioned. Ligament tensioning can be accomplished using numerous devices such as laminar spreaders or implant-specific tensioners. The AP femoral cutting block is then positioned parallel to the resected proximal tibia. The block is adjusted anteriorly or posteriorly to create a flexion gap width equal to that of the previously established extension gap (Fig. 5.6a) [20, 21, 37]. A spacer block of identical width of that selected for the extension gap is placed beneath the AP cutting block and on top of the resected proximal tibia before the AP femoral cuts are made to assure flexion-extension gap symmetry (Fig. 5.6b). If the implant system utilized has a femoral component in which the thickness of the distal and posterior aspects of the femoral condyles is not identical, the AP position of the block is adjusted accordingly to assure equal soft tissue tension in both flexion and extension. To increase the precision of this gap balancing method, the authors also construct the transepicondylar and AP axes and use these as secondary determinants of femoral component rotation. If the knee is well balanced in extension, the proximal tibial resection is accurate, and the medial and lateral soft tissue flexion gap stabilizers are intact, the femoral cutting block will be positioned parallel to the resected tibia and transepicondylar axis and perpendicular to the AP axis.
