Materials in Hip Surgery
Bioactive Coatings for Implant Fixation
Dale R. Sumner and Amarjit S. Virdi
Key Points
The nature of the implant surface and the health status of the host affect the biological response and the ability to attain secure implant fixation. Currently, it is not clear if it would be helpful to use different bioactive coatings to maximize the response from different patients (personalized medicine).
Introduction
The need to replace and restore diseased tissues has paralleled the increase in longevity of the general population. This is particularly true in dentistry and orthopedics, where structure and function have been restored by prosthetic implants. These procedures have been enormously successful and have improved the quality of life of a large number of people. This success, for the most part, can be attributed to the body’s own capacity to integrate with the implants. In return, implant materials and surface characteristics have been adopted that most efficiently elicit body responses. The surface of the implants can be modified in a variety of ways, either directly or by placing a coating to support fixation to the host bone.
Here, we review bioactive coatings for implant fixation. The term bioactive suggests a biological effect on surrounding tissue. The term coating implies the presence of a covering layer. Thus, a bioactive coating is a treatment for an implant that creates a surface layer meant to impart a biological effect. Some of the early references to bioactive coatings refer to bioglass in 19781 and to hydroxyapatite2 coating of metal implants in 1980. For our purposes, the most important biological effect of a bioactive coating is one that leads to improved long-term mechanical fixation of the implant to the host. Primary mechanisms include enhanced bone-implant contact, improved architecture of peri-implant bone, and reduced chance of infection (Fig. 10-1).
Figure 10-1 Potential mechanisms of action of bioactive coatings.
The focus on enhanced long-term mechanical fixation of the implant as the desired goal is based on the supposition that secure coupling of the implant and the host bone will contribute to long-term clinical success. Even if a bioactive coating does not allow an implant to perform its intended function for a longer period (i.e., improve survivorship), decreasing the time needed to obtain fixation would be beneficial.
In the most general sense, the implant surface can vary by topography, chemistry, and surface energy.3 In Chapter 9 of this text, considerable attention is given to topography at the macro level, as that chapter focuses on the use of porous metal coatings for implant fixation. Here we focus on various means of altering surface topography at the micrometer and nanometer scale, altering the surface chemistry, and functionalizing the surface through the addition of peptide motifs, growth factors, or other agents to modify the response of local cells and tissues.
Basic Science of Bioactive Coatings
Definitions
Bioactive coatings have one major long-term function: to ensure long-term mechanical fixation of the implant to the host skeleton. The primary mechanisms by which this can happen include enhanced bone regeneration, increased bone-implant contact (even in the absence of enhanced bone regeneration), and inhibition of bacterial colonization of the implant surface. As depicted in Figure 10-1, these mechanisms are not mutually exclusive. Although it is possible to impart antibacterial properties directly to implant coatings, it is also likely that enhanced bone-implant contact indirectly inhibits infection by reducing the chance of bacterial colonization. Experimental data demonstrate that improved bone-implant contact impedes ingress of particles along the bone-implant interface.4–6 Therefore, improved bone-implant contact not only provides stability to the implant, it also reduces the risk of implant loosening by preventing wear particle debris from migrating along the implant and causing peri-implant osteolysis.
Three key terms defining the interaction between bioactive coatings and bony tissues are osteoinduction, osteoconduction, and osteointegration.7
2. Osteoconduction refers to bone growth on the surface of the implant, although the term has also been defined as the ability to facilitate angiogenesis and new bone formation in the context of bone graft substitutes.8
3. Osteointegration was originally defined as direct bone-implant contact at the light microscopic level (i.e., a static result of the dynamic process of osteoconduction), but more recent definitions refer to rigid mechanical fixation of the implant in the face of functional loading.7 For implants lacking a porous surface or other surface topography that can impart resistance to shear and tensile forces, direct bone-to-implant contact is presumably important for attaining mechanical fixation. For implants with a porous surface, interlocking of bone tissue with the porous surface can provide rigid mechanical fixation even in the absence of direct contact between the bone and the underlying implant surface.
Biological Response to Implants
All implants are perceived by the body as foreign objects; therefore, they elicit a biological response as a “nonself” material to counter any adverse effects. This reaction is based on factors related to the implant and factors related to the host. We discuss here implant-related factors that are dependent on the physical, chemical, and biological characteristics of the implant surface. We do not discuss in any detail tissue-related factors such as the implant site, patient gender and age, tissue integrity, and systemic conditions. However, the reader should appreciate that the biological response to a given implant should not be assumed to be uniform across all patients.
In general, the body’s reaction to an implant is determined by the characteristics of its material of manufacture. The term bioinert implies that the reaction to the implant is absent. In actuality, the introduction of bioinert materials causes an interaction with surrounding tissue that can result in a minimal response. The outcome is the formation of a fibrous membrane that encapsulates the implant with no effective bonding. Bioactive materials, on the other hand, trigger a reaction with adjacent tissue to initiate a cascade of events leading to synthesis of new extracellular matrix, which under the best circumstances forms in close contact with the implant and leads to mechanical fixation. It is worth noting that if the implant elicits an adverse reaction, such as causing cell death in surrounding tissue, it is referred to as a toxic material.
In Situ Modification of Implant Surfaces
Introduction of biomaterials at the site of implantation initiates a series of events that occur on varying time scales and length scales. These events play critical roles in the eventual outcome that may result in acceptance or rejection of the biomaterial. In the orthopedic field, experience is sufficient to allow the design of materials with minimal chance of rejection, but an appreciable knowledge gap has been noted in the conditions required to maximize osseointegration and the long-term success of joint replacements. To this end, it is important to understand interactions between the implant and cells and tissues in immediate proximity to the implant.
Even before an orthopedic implant is placed in the body, the implantation site is subjected to local trauma during surgical preparation. This process causes mechanical disturbance of tissue organization and to some extent cell death due to shear stress and heat generation. It is also inevitable that the surgical procedure breaks blood vessels and results in bleeding. Therefore, this process triggers an inflammatory response that influences the implant through changes in cytokine/chemokine status and cell kinetics in the area. In addition, at this stage, there exists an opportunity for microbial infection that would adversely alter the healing process. Overall, the implant surface encounters a hostile environment that challenges its biocompatibility.
In simple terms, we can consider bioactive implant coatings as ex vivo modifications of surfaces to enhance osseointegration; however, it is worth bearing in mind that these surfaces are subject to additional changes in vivo that may lower or elevate this bioactivity. It is nearly impossible to study the relevant contributions of initial ex vivo modifications and subsequent changes due to in vivo events. Let’s consider the earliest events that occur on the implant surface following implantation. According to Kasemo and colleagues, the surface is subject to modification almost immediately after placement at the surgical site.9,10 Water molecules present in the physiologic fluid interact immediately with the implant surface and form a double layer. This interaction happens within a few nanoseconds after the implant surface is exposed to body fluid and depends on the hydrophilic/hydrophobic properties of the surface (i.e., its wettability). The aqueous layer then attracts cations and anions to form a complex that adsorbs protein biomolecules from the surroundings.
The interaction of endogenous proteins with the implant surface depends on its geometric, chemical, and electrical characteristics.11 For example, rough surfaces provide greater area for proteins to adsorb than smooth surfaces. The local surface charge due to the distribution of cations and anions determines the adsorption of proteins with corresponding charges. The complexity of the physiologic fluid indicates a very heterogeneous distribution of proteins on the implant surface. Once a protein is adsorbed, its conformation may change, thereby exposing active sites for cell binding and intracellular signaling. The composition and organization of this protein layer determine the specificity of this interaction. The scenario described here implies that uniform cell response occurs at the implant-tissue interface once the protein layer is deposited and the cells have become attached. In fact, the composition of this protein layer is very dynamic and may change constantly over the whole process of tissue neogenesis adjacent to the implant. The composition of this layer is determined by many factors, including possible degradation of the surface material or adsorbed proteins and arrival of new proteins during the repair process and competitive binding.
Bone Repair and Regeneration
It is useful to briefly review the regenerative context in which implant fixation is seen (Fig. 10-2). Implant fixation occurs via the intramembranous pathway.12 Careful ultrastructural examination of the titanium-bone interface in a rat model has shown that mineralized bone begins to form de novo a short distance from the implant surface at day 5.13 Bone formation is not initiated on the implant surface or from existing bone surfaces. These early bone spicules are later encased by lamellar bone, which achieves direct contact with the titanium surface by day 14. It is possible that bioactive coatings may promote direct initial bone formation on the implant surface, but studies at this level of detail are not yet available.
Figure 10-2 Photomicrograph at an early time point following placement of a hydroxyapatite-coated titanium implant in a rat model (7 days). Note the presence of woven bone near the implant, which is an integral part of the reparative stage of the regenerative response following surgery. This is an undecalcified plastic-embedded section, ground to approximately 50 µm and stained with basic fuchsin and toluidine blue (scale bar = 100 µm).
The marrow ablation model provides a relatively simple means of investigating the intramembranous pathway and is typically performed in the mouse or rat.14 In this model, medullary space in the long bones is accessed by drilling a hole in the cortical bone or the condyle. The marrow content is then mechanically removed by vacuum or is flushed out with sterile saline. Although other models can be used to study implant fixation,15,16 the rat ablation model has proved particularly useful because it allows investigation of the reparative cascade in detail. The response to marrow ablation surgery typically is divided into three major overlapping phases: (1) inflammation, (2) repair, and (3) remodeling,14,17,18 paralleling the concepts of fracture healing.19 Although we will not describe details here, some agents or bioactive coatings affect only one of these phases, while other agents or coatings may have more pervasive effects. Although our understanding of the histologic changes characterizing these phases is well established, more information on the molecular and cellular mechanisms is now being gathered, including temporal and, to a limited degree, spatial patterns of gene and protein expression following marrow ablation.17–20 It is likely that the bioactive coatings manipulate or alter these cascades, but this is a relatively unexplored area.21 One of the important concepts is that bone repair mimics embryonic development. This is not exactly the case19,22; however, parallels are evident, and it does seem likely that improvements in understanding of the bone regeneration response in both the absence and presence of an implant23 will ultimately lead to novel strategies for improving implant fixation.
Surface Topography
Surface structure is known to influence the long-term mechanical fixation of implants. Chapter 9 in this volume covers millimeter scale surface structures as provided by porous metals. Here, we describe surface topography at the micrometer and nanometer scale. Most studies have been performed in the context of dental implants24–26; thus, a majority of the work has been performed on commercially pure titanium. Less information is available on titanium alloys and cobalt-based alloys, which are frequently used in orthopedics.
Implant surface topography is manipulated by using subtractive processes such as polishing, blasting, acid etching, or oxidation, and by using additive processes such as coating with calcium phosphates, use of titanium via plasma spray, and ion deposition.24 These treatments alter various quantitative characteristics defining the implant’s surface roughness and topography, including the arithmetic mean deviation of a profile (Ra) or surface (Sa)—the most frequently reported characterization parameter. In an extensive review of the literature, Wenneberg and Albrektsson24 reached the following conclusions:
It should be noted that changes in surface roughness are often accompanied by changes in surface chemistry such as charge or wettability, and it is often difficult to determine which factor is responsible for the observed effect.25 Despite this limitation, a growing body of literature examines the response of various cell types to biomaterial surfaces in vitro.3,27,28 These studies are often designed to answer one of two basic questions:
1. What surface characteristic will lead to the best osteointegration?
2. Which cell or molecular pathway is involved in how cells interact with surfaces?
For the first question, there is often an underlying assumption that a particular in vitro endpoint such as cell adhesion, migration, or proliferation will predict the in vivo behavior. In some cases, correlative studies now involve both in vitro and in vivo work. Variation in a number of experimental details, including characterization of the implant surface, the cell population(s) studied, the culture media used, the timing of observations, and the plating density, makes it difficult to compare individual studies. Nonetheless, a considerable amount has been learned about how cells interact with artificial materials. It is becoming clear that topographic features, surface chemistry, and surface energy affect protein attachment to the implant and, therefore, influence cell attachment, shape, proliferation, and differentiation.3 Because of their important role in cell attachment, the integrins are key players through which cells transfer information from the implant surface to influence cell behavior.29
The reported experience with roughened surfaces in orthopedics is meager. In a comparison of porous coated and grit-blasted femoral stems in THA in which both types of components were further treated with a hydroxyapatite coating, the authors reported no differences in fixation or peri-implant bone remodeling at 2 years.30 Because of the use of the hydroxyapatite coating, it is not possible to make a direct comparison of the roughened surface performance versus the porous coating performance.
Surface Chemistry
In terms of alterations in surface chemistry, we include discussions of many treatments that are meant to act through mechanisms not related to surface topography, although, as already noted, it is not always clear that these two variables can be considered independently. These surface alterations include calcium phosphate coatings, bioactive glass coatings, oxidation, and surfaces functionalized with other bioactive agents such as peptides, growth factors, and antibacterial materials. The term biomimetic deserves definition because it sometimes is used to describe a particular way of creating a calcium phosphate coating and sometimes is used to describe the more general concept of creating a surface that demonstrates particular functional characteristics. In orthopedics, the best investigated and most frequently used means of altering surface chemistry is to apply a calcium phosphate surface to the implant.
Calcium Phosphate Surfaces
Interest in using calcium phosphates (CaPs) as bone graft substitutes and for implant coatings has been considerable26,31 (Fig. 10-3). The term hydroxyapatite (HA) refers to a particular form of CaP found in bone, and some CaPs mimic the structure and composition of bone HA. Thus, not all CaPs are HAs. CaP coatings are bioactive because they are osteoconductive and have been shown to improve bone-implant contact and mechanical fixation of implants in the presence of interface gaps of up to 2 mm.32 CaPs are attractive as coatings for joint replacement implants because of lack of toxicity, lack of an inflammatory response even to particulates, and direct bone bonding.33 Some concern has arisen that pieces of CaP coatings can reach the joint surface and cause third-body wear.
Figure 10-3 Photomicrograph at 4 weeks following placement of a hydroxyapatite-coated titanium implant in a rat model. Note the presence of lamellar bone with a row of osteoblasts (closed arrow) and a region of bone resorption that appears to extend into the hydroxyapatite coating (open arrows), underscoring the dynamic nature of the bone-implant interface. This is an undecalcified plastic-embedded section, ground to approximately 50 µm and stained with basic fuchsin and toluidine blue (scale bar = 100 µm).
Hydroxyapatite coatings seem to induce direct bone-implant bonding even in the presence of some micromotion at the interface, and low-crystallinity HA coatings may be more beneficial for early bone ingrowth. Improved bone ingrowth is associated with inhibited migration of polyethylene particles along the interface.6 When these HA coatings are resorbed, no loss of implant fixation is apparent.
The plasma flame spray method is the most commonly used technique commercially for coating implants; it results in coating thickness in the range of 20 to 250 µm. A limitation of the plasma spray technique for applying CaP coatings is that the method is “line-of-site,” meaning that surfaces not facing the source remain uncoated; there is also concern about occluding the openings of porous coated surfaces. CaP coatings can be created by a biomimetic process in which CaP nucleates from supersaturated solutions called simulated body fluids.34 This technique creates thinner CaP coatings of varying composition that can cover all surfaces of implants with more complex surface topographies (i.e., porous coatings).
In vitro studies have shown that CaP surfaces promote osteogenic differentiation of human mesenchymal stem and MG-63 cells (a model of osteoblasts) even in the absence of osteogenic additives to the media.35 These authors found elevated alkaline phosphatase activity and gene expression and increased gene expression of a key osteogenic transcription factor, Runx2.35 Because of the importance of cell interaction with the substrate (which might mimic cell–extracellular matrix interactions in vivo), these authors investigated focal adhesions and found that the number and size of cellular structures were reduced, but their mobility was increased, when MG-63 cells were cultured on a calcium phosphate surface rather than tissue culture plastic or titanium.35 Although it is not possible to attribute the response definitively to surface chemistry as opposed to topography, the ability to direct differentiation of cells without using exogenous growth factors is potentially very significant.
An extensive in vitro study examined the response of three cell types to different formulations of calcium phosphate in the presence and absence of adsorbed BMP-2. All formulations were of similar composition (80% hydroxyapatite and 20% β-tricalcium phosphate), with varying microstructure (pore size and porosity), crystal size, specific surface area, ability to adsorb proteins, and surface roughness. Experiments showed that the ability to concentrate proteins with increased surface area improved the ability to differentiate cells along the osteogenic lineage.36 Cell function was not dependent upon micropore size, crystal size, or surface roughness. Although the conclusions are valid only for the conditions studied, this paper nicely demonstrates the complexity of sorting out factors involved in controlling cell behavior and the caution that needs to be applied when extrapolating to other surface types or cells. Some of these conditions have been associated with the ability of these different materials to promote bone attachment or to induce bone formation in vivo.36
Here, we briefly review the clinical data because there is now a 20+-year history of use of CaP as a bioactive coating in joint replacement. We are aware of nine studies in which the in vivo fixation of calcium phosphate–treated tibial components of total knee arthroplasty was compared with fixation of similar untreated components (Table 10-1). In all of these studies, implant fixation was assessed with roentgen stereophotogrammetric analysis (RSA), a technique that allows the study of implant migration and inducible motion at much finer sensitivity than is attained with conventional radiographic analysis. Findings tend to show that calcium phosphate coatings were associated with less implant migration, although the differences were not always statistically significant. In addition, it has been reported that HA-coated tibial components without adjuvant screw fixation had a much lower incidence of radiolucent lines at the bone-implant interface than did comparable uncoated tibial components that had adjuvant screw fixation.37
Table 10-1
RSA Studies of Tibial Component Migration in Total Knee Arthroplasty
HA, Hydroxyapatite; MG, Miller-Galante; RSA, roentgen stereophotogrammetric analysis; TCP, tricalcium phosphate.
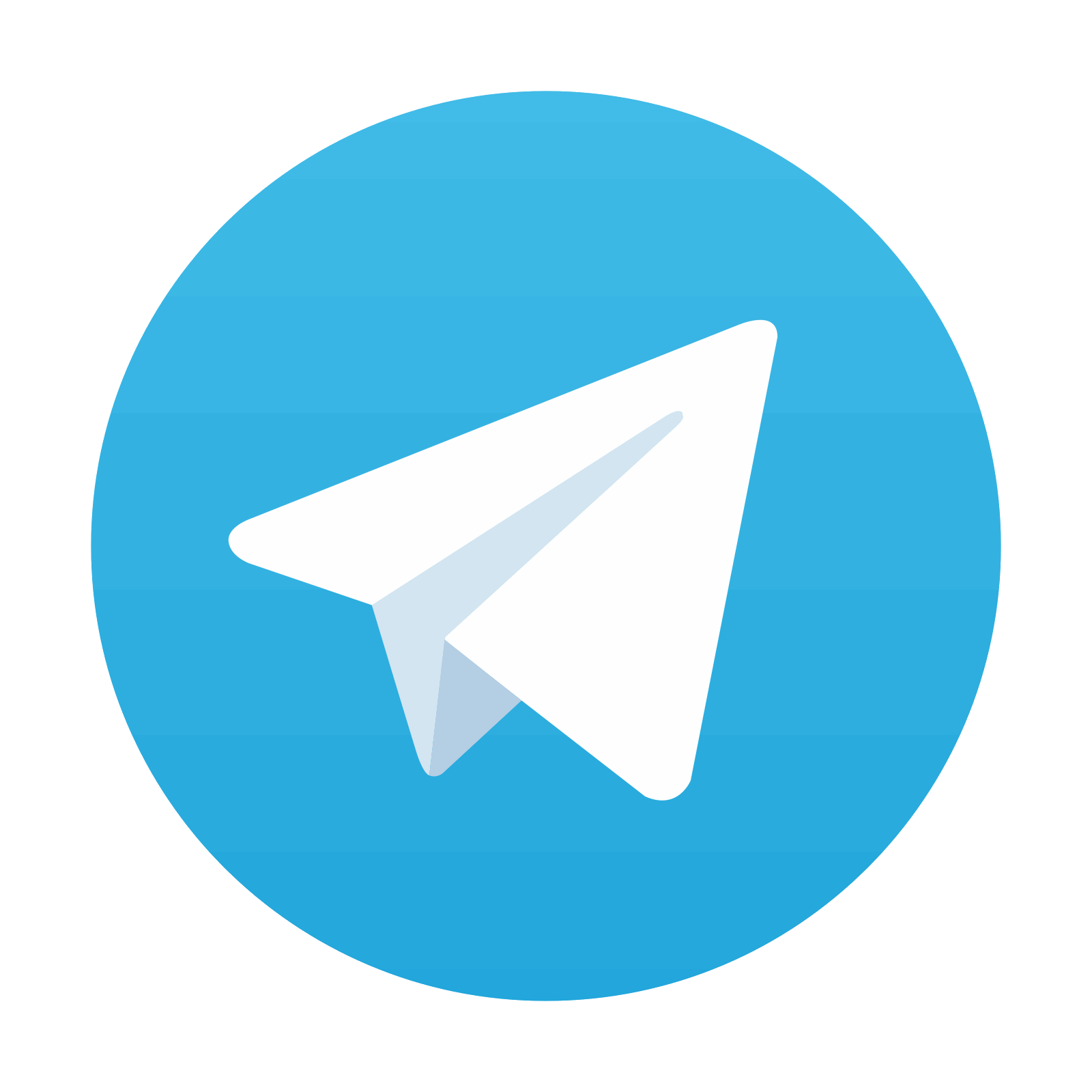
Stay updated, free articles. Join our Telegram channel

Full access? Get Clinical Tree
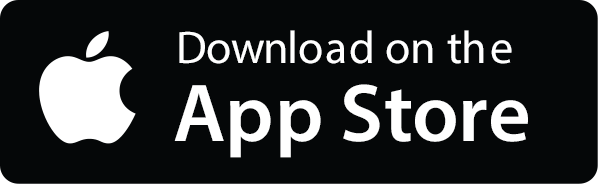
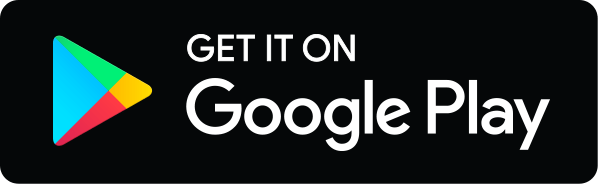