FIGURE 47-1. Grade 3 instability with valgus stress due to MCL deficiency.
Radiographic analysis allows further assessment of the problematic TKA. At a minimum, a weight-bearing AP, lateral, and sunrise views are required. On the AP radiograph, the condition of the medial and lateral epicondyles should be noted as osteolysis and/or avulsion fractures may be evident resulting in varus or valgus instability in the unconstrained TKA. In addition, an attempt should be made to determine the type of prosthesis present and the amount of constraint in the current design. Lastly, evaluation for implant wear, loosening, and available bone stock is mandatory to allow one to assess the reconstructive options. In some instances, long-standing hip to ankle films may be necessary to help elucidate the limb alignment and extraarticular deformity. Also, fluoroscopically directed views, placing the x-ray beam tangential to the fixation interface, are useful in establishing implant fixation. Stress radiographs may be helpful to document instability preoperatively (Fig. 47-2). Finally, limited CT scans offer a perhaps more accurate assessment of component rotational alignment, bone loss around an implant, and the integrity of bone at the origin and insertion of collateral ligaments.
CLASSIFICATION
Stability of the knee following TKA is afforded by a combination of factors related to the host, implant, and surgical technique. Host factors primarily relate to the integrity of the bony architecture and soft tissues, in particular the collateral ligaments, capsule, and surrounding musculature. Implant factors relate largely to the inherent constraint and to a lesser extent articular conformity. Technical factors involve overall coronal, sagittal, and rotational alignment, component sizing, and soft tissue balancing in flexion and extension.
Early total knee implant designs utilized fixed hinges providing rigid constraint to the knee. Stability was almost entirely dependent upon the implant and relegating little if any functional role to the surrounding soft tissues. These early designs including the Walldius, Shiers, and GUEPAR hinged prostheses suffered high failure rates due to loosening, infection, and mechanical failure.5,6 One reason for the high loosening rates associated with these designs relates to the high stresses placed at the bone-cement or bone-implant interface.
FIGURE 47-2. Stress radiograph demonstrating varus instability.
To minimize these high loosening rates, total knee designs evolved into implants with less constraint utilizing surrounding soft tissues for stability. Improvements in loosening rates were evident, but a relatively small yet significant number of these developed instability which became a new challenge. These less constrained designs required careful attention to soft tissue balancing of both the flexion and extension gaps. If these gaps are not adequately balanced, or the soft tissue restraints are compromised, the following types of instability patterns may result.
Coronal Plane Instability Instability in the coronal plane is primarily due to imbalance or deficiency of the collateral ligaments. The superficial MCL is the primary restraint to valgus stress. A well-aligned TKA with the mechanical axis in the center of the knee usually requires 5 to 7 degrees of anatomic femoral-tibial valgus. If the MCL is attenuated or absent and this is not addressed at the time of arthroplasty, the knee is doomed to fail secondary to valgus instability (Fig. 47-3).
FIGURE 47-3. Complete disruption of the MCL with gross valgus instability.
The LCL is the primary constraint to varus. Assuming a well-aligned knee in 5 to 7 degrees of anatomic valgus, some degree of laxity in the LCL is usually tolerated especially if the secondary constraints such as the popliteus, iliotibial band, biceps femoris, and posterolateral capsule are preserved. These two factors probably account for the relative lower incidence of varus instability patterns. However, complete or near-complete absence of varus restraints combined with an overall varus alignment portends functional varus instability.
Sagittal Plane Instability Instability in the anteroposterior direction is usually due to a loose flexion gap. As described earlier, the primary restraints to posterior instability are the PCL, the extensor mechanism, and any inherent implant constraints. Perhaps the first most successful resurfacing total knee prosthesis was the total condylar prosthesis developed by Insall in 1973. The design featured a cobalt-chromium alloy femoral component and an all-polyethylene tibial component with biconcave tibial plateaus and an intercondylar eminence designed to limit side-to-side sliding motions.7 It was designed for cruciate ligament excision and had a reported survivorship of 94% at 15 years.8 The tendency for this prosthesis to sublux posteriorly in flexion with less than perfect balancing combined with the limited posterior femoral rollback was associated with limited range of motion and led to modifications that included a cam and post mechanism (PS TKA) that effectively substituted for the PCL. This design feature allows for posterior femoral rollback in flexion and limited the problem of posterior subluxation. It still requires careful technique to balance the flexion gap and relies upon integrity of the cam and post mechanism to provide for these technical improvements. A loose flexion gap in a PS TKA can cause posterior dislocation via two mechanisms.9,10 The first mechanism involves laxity of the LCL. As the knee is flexed and a varus stress applied as in the figure-four position, the tibial post disengages from the femoral box and the tibia subluxes posteriorly. The second mechanism involves hyperflexion of the knee in the presence of a loose flexion gap, resulting in posterior dislocation, in which the tibial spine can lock behind the femoral cam.
Since modern total knee designs require resection of the anterior cruciate ligament, anterior stability is primarily achieved through component geometry, articular conformity, and soft tissue restraints such as the hamstrings and iliotibial band. Once again this problem usually arises in the setting of a loose flexion gap (Fig. 47-4).
FIGURE 47-4. Posterior tibial translation resulting in dislocation. This PS TKA had severe flexion-extension space mismatch.
The posterior cruciate retaining TKA evolved during this same period. Where the PS design incorporates a cam within the femoral component and post on the tibial component to limit posterior subluxation and improve posterior femoral rollback, the PCR designs rely upon the native PCL to accomplish this function. Preserving the PCL provides posterior restraint and allows posterior femoral rollback to occur as the knee is flexed. With this design, intraoperative injury to the PCL or excessive PCL recession as is sometimes necessary to balance the knee in flexion may compromise the normal or intended function of the ligament lending itself to potential early posterior flexion instability.4 Late flexion instability may also occur from chronic attritional rupture or degeneration of the PCL.3
Global Instability Global instability is due to laxity in both flexion and extension. This is a manifestation of a poorly balanced knee, marked component malalignment, polyethylene wear, or component loosening.10
PROSTHETIC SELECTION
Several characteristics of the implant system are important to consider prior to selection of the prosthesis to be utilized in the revision. First, revision TKA is technically demanding; therefore, surgeon familiarity with the implants and instruments are essential. Knowledge of the modular stems and augment options available, levels of constraint, geometry and kinematics of the femoral and tibial components, and tibial baseplate locking mechanism are all important considerations. For instance, in most revision TKA cases, bone loss after component removal necessitates the use of stems. In many circumstances, adequate fixation may be obtained with cement or cementless fixation.11,12 Most implant systems offer varying degrees of constraint for their revision total knee systems ranging from cruciate retaining, PS, constrained condylar, to hinge designs. The level of constraint necessary is best determined intraoperatively after component removal and an assessment of bone loss, ligament deficiency, and gap balance have been determined.13,14
Nonconstrained Revision TKA Implants
Cruciate Retaining Revision Total Knee Designs. Posterior cruciate retaining TKA prostheses have a very limited role in the revision TKA setting. These prostheses require an intact and functional PCL which is rarely present in a failed TKA. Furthermore, PCR TKA designs require careful balancing of the PCL in flexion which is often difficult in a revision TKA. While it is possible to use a PCR TKA in conditions such as a revision of a failed unicondylar knee arthroplasty, or occasionally in a PCR TKA, we have found that the PCL is frequently degenerative, or bone loss is too great involving the femoral and tibial attachments rendering the ligament incompetent. We have found more predictable results with use of the PS designs in the revision setting. Concerns of flexion instability and less reliable posterior femoral rollback limiting range of motion are serious concerns with these designs in the revision setting.
Posterior Stabilized Designs. Posterior cruciate–substituting designs tend to be the more commonly used implants in revision TKA. The advantages of these implants include the easier correction of deformity,15 enhanced stability in flexion, and increased motion secondary to forced posterior femoral rollback.16,17
Not all PS designs are the same. For instance, some PS designs have the cam and post mechanism engage at 70 degrees of flexion, thus the stabilizing feature during low flexion activities such as walking has no influence. Other prostheses engage as early as 30 degrees of flexion and thus will have earlier cam and post interaction and posterior femoral rollback occurring at earlier degrees of flexion. These early cam and post engagements may theoretically enhance quadriceps function due to an increased quadriceps lever arm but may have implications related to postwear.
PS designs also vary according to the amount of bone removed from the intercondylar box. This is particularly important to recognize during revision surgery when the surgeon either knowingly, due to severe distal femoral bone loss, or unknowingly inadequately restores the joint line. Resection of bone for the intercondylar box may increase the risk of condylar fracture due to the proximity of the intercondylar bone resection to the medial cortex of the medial femoral condyle14 (Fig. 47-5). While the implant provides stability in flexion due to the cam and post interaction, significant increases in the flexion gap will predispose the knee to tibiofemoral dislocation in flexion. This implant provides no constraint to varus and valgus stresses and in the setting of collateral ligament deficiency requires ligament repair, reconstruction, or augmentation, the results of which have been highly variable.18–21
Constrained Implants. The use of constrained implants (constrained condylar vs. hinged TKA) are often required in one of these clinical scenarios. The first is when the flexion and extension gaps are unable to be adequately balanced. The second indication is when substantial laxity or complete absence of the collateral ligaments exists, and repair or reconstruction is not possible or unable to properly stabilize the knee. Finally, these options are absolutely essential if on preoperative clinical exam, functional loss of the LCL or MCL is apparent and severe bone loss is present.
Constraint refers to a restriction of rotational or translational movement which can be grouped into two large categories: linked and unlinked designs.13 Unlinked constrained designs typically utilize an enlarged tibial polyethylene post that interlocks with the intercondylar box of the femoral component which provides resistance to motion when posterior or varus and valgus translational forces are applied (Fig. 47-6). The cam and post mechanism of an unlinked constrained implant also provides resistance to internal and external rotational forces.14 Standard PS designs do not provide this level of restriction to motion. This type of constraint is offered by implants typically termed constrained condylar knee (CCK) prostheses (Fig. 47-7) or varus-valgus constrained prostheses. Each manufacturer’s implants will vary with regard to the degree of translational and rotational constraint provided as well as the “jump distance” which translates to the threshold height of separation between the tibial post and femoral cam above which dislocation of the tibial spine from femoral cam can occur (dislocation height) (Table 47.1). Unlinked constrained devices offer a clear advantage in the additional mechanical stability they provide to the knee. This is useful in two clinical scenarios. First, when collateral ligament laxity exists and is irreparable or nonreconstructable, and second, when substantial flexion and extension imbalance exists and is inadequately stabilized with traditional balancing techniques. The disadvantages of the increased constraint, however, must not be trivialized. Increases in the articular constraint result in increased contact stresses which are transferred to the fixation interfaces. This is clinically relevant as this mechanism is postulated to result in and increased incidence of implant loosening over time.
FIGURE 47-5. Diagram showing reduced medial versus lateral bone mass present at the proximal margin of the intercondylar bone resection required for implantation of a posterior cruciate–substituting total knee prosthesis. (From Hass BD, Dennis DA. Implant selection in revision total knee arthroplasty. In: Callahagn JJ, Rosenberg AG, Rubash HE, et al., eds. The Adult Knee. Philadelphia, PA: Lippincott Williams & Wilkins; 2003, with permission.)
FIGURE 47-6. Left: A constrained condylar polyethylene insert with its enlarged tibial post providing varus/valgus constraint. Right: A PS polyethylene insert that provides no resistance to varus/valgus motion. (Redrawn from Krackow KA. Managemnet of medial collateral ligament loss repair and augmentation. In: Lotke P, Garino J, eds. Revision Total Knee Arthroplasty. Philadelphia, PA: Lippincott-Raven; 1999;chap 15.)
FIGURE 47-7. AP x-ray of a CCK with press-fit stems.
Linked constrained prostheses offer the ultimate constraint. These types of implants refer to designs that join the femoral and tibial components to each other usually with an interlocking pin placed through the femoral condyle and tibial spine. Most older linked designs permitted motion in the sagittal plane alone. Modern hinge designs typically allow rotation to occur at the tibial base plate and polyethylene interface allowing a reduction in contact stresses and hopefully decreasing loosening rates. These designs are termed rotating-hinge prostheses. In the authors’ experience, the need for these designs is exceedingly rare. Indications for their use include severe instability associated with extensor mechanism allograft reconstruction, total nonreconstructable MCL deficiency, massive bone loss secondary to tumor resection, or comminuted supracondylar femur fracture not amenable to fixation.
SURGICAL TECHNIQUE
Following removal of the failed implant, the quality of the bone and the supporting ligaments need to be assessed. Sometimes, instability is a result of bone loss (pseudolaxity). In these cases, the soft tissue envelope is intact and by augmenting the prosthesis and dealing with the bone loss, the soft tissue sleeve comes under tension and stability is restored. Other times, instability is a result of ligamentous deficiency and this will need to be addressed with ligament reconstruction or, more commonly, a constrained implant. The following surgical technique will explain how to manage both scenarios.
The revision begins with reconstruction of the tibia. This is done because the tibial surface affects both extension and flexion gaps, whereas the distal femur affects only the extension space and the posterior femur affects the flexion space. The goal is to create a flat tibial surface perpendicular to the mechanical axis of the tibia. This should be done with minimal removal of host bone. Bone defects are identified but must not be eliminated simply by excessive resection of host bone.
If bone loss is present, it is helpful to classify the defect. Defects may be classified according to size, location, and depth of the defect, and the presence or absence of an intact peripheral rim of bone for placement of a prosthesis or containment of bone graft. The Anderson Orthopedic Research Institute (AORI) Bone Defect Classification system15 is useful as it describes the defects on the femur and tibia separately and is based on the quantity of bone remaining from the metaphysis after component removal (see Chapter 46). It does not, however, distinguish between contained and uncontained defects that are important when considering the use of cement fill or particulate graft which are more easily impacted into these types of defects. For contained defects, it seems most appropriate to use cancellous graft.16 For uncontained defects, loss of the cortical rim implies lack of supportive bone to provide a stable platform to the prosthesis; therefore, modular augments are preferred. When peripheral bone loss that is too large for augments exists, structural allografts may be more appropriate.17 A more comprehensive discussion of bone defects and their management is addressed elsewhere in this book (see Chapters 47 and 48).
TABLE 47.1 Posterior Stabilized and Constrained Condylar Knee Prostheses
Data obtained from manufacturer.
aAt 90 degrees of flexion.
bSize 7.
Adapted from Griffin WL, Fehring TK, Valadie AL. Revision of the unstable total knee arthroplasty. In: Engh GA, Rorabeck CH, eds. Revision Total Knee Arthroplasty.
Baltimore, MD: Williams & Wilkins, 1997:340–355;chap 18.
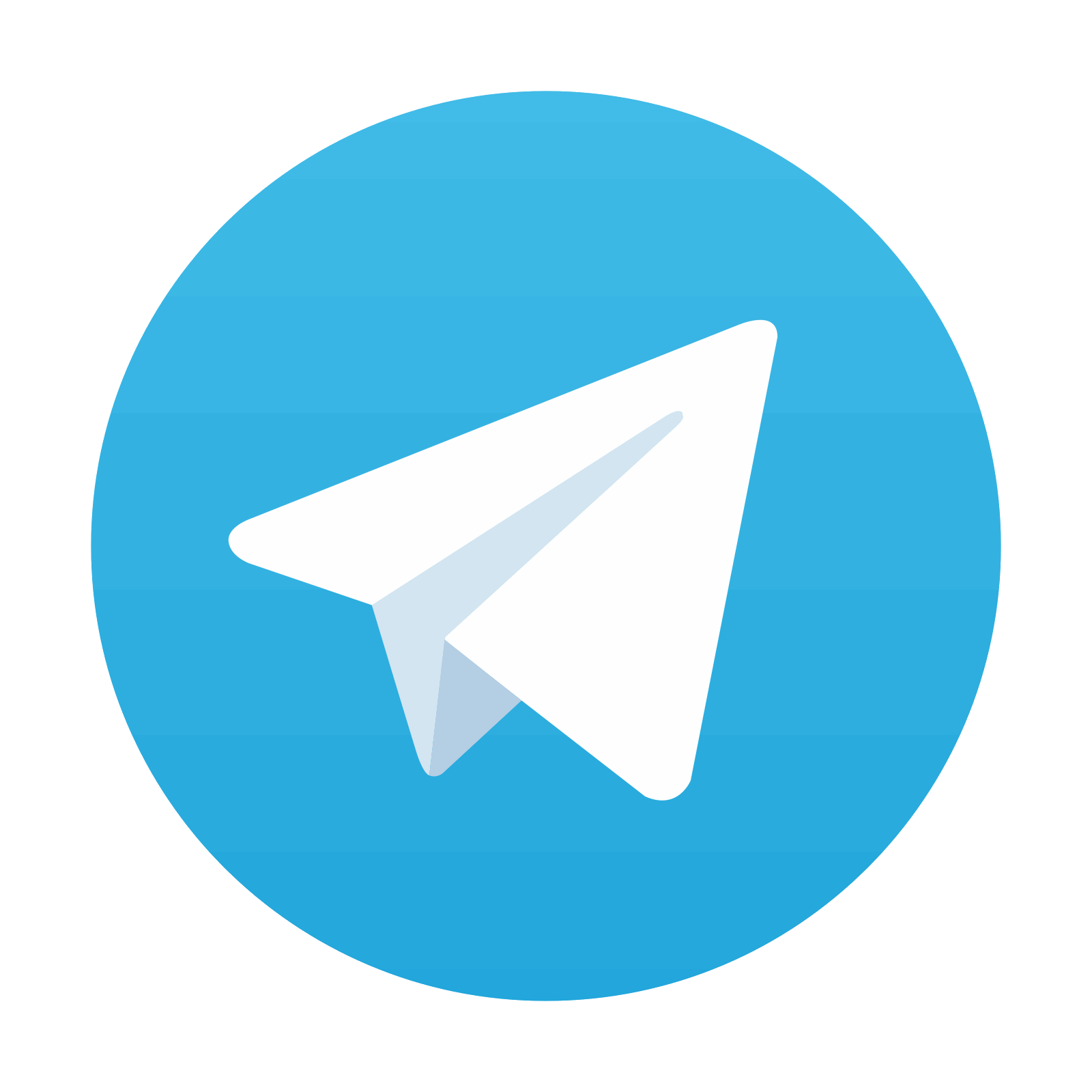
Stay updated, free articles. Join our Telegram channel

Full access? Get Clinical Tree
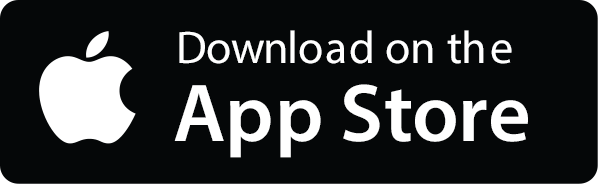
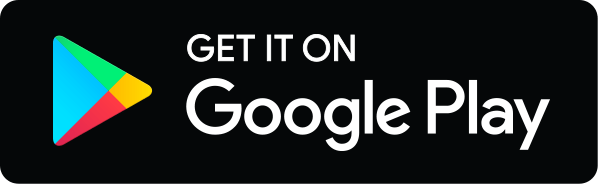