Chapter 19 Knee Wear
Wear and Damage
Components of Wear
Scratching and Pitting
Deformation of tibial inserts by scratching and pitting, which often reflect damage but not wear, can be visually striking, yet represent only superficial surface changes in the polyethylene. This type of damage is almost ubiquitous on clinical retrievals and can result from third-body debris such as cement, residual bone fragments, or loose porous-coating beads. Scratched and pitted surfaces look damaged, but the damage may be only cosmetic (Fig. 19-1).
Scratched and pitted surfaces have been reproduced in laboratory simulations of the knee environment in the absence of material removal. Atwood and colleagues3 have demonstrated reproducible scratching and pitting of polymer pins in an oscillating pin on flat experiment. Of note, the damage and characteristic skipping marks observed in retrieval studies were not only reproduced on the polyethylene pins, but clinically comparable scratching was apparent on the cobalt-chromium-molybdenum (CoCrMo) counterface. This damage occurred when bone cement and bone debris from cutting were introduced into the articulation.
The ability of a softer material to scratch a harder material is documented elsewhere in the tribology literature.32,52 In knees, the result is a scratch running in the direction of the predominant motion. It is well documented that counterface roughness and scratching can have dramatic effects on the wear rates of polymer bearings, depending on the degree of crossing motion encountered by the system.25 Therefore, in vivo scratching of metallic femoral components is important to monitor when evaluating tibial wear.
Fatigue Failure
Fatigue failure occurs in all types of gamma-sterilized tibial inserts, regardless of design, material, or method of fabrication (molded or machined; Fig. 19-2).12 The incidence of fatigue failure increases with time in vivo as oxidation and cycles of use increase. Higher initial oxidation means fewer cycles before failure. Hence, devices that were gamma-sterilized in air failed after shorter in vivo durations if they were first stored on the shelf. Recent findings have shown that even gamma barrier–sterilized inserts eventually oxidize and therefore are subject to fatigue over the longer term (Fig. 19-3).
From a clinical perspective, it is difficult to predict when a device will fail because of fatigue mechanisms. Whereas most industrial fatigue life calculations only need to account for the distribution of stress and cycles over a period of time, the orthopedic engineer needs to be cognizant of both the changes in material properties and the magnitude of stresses as a function of time. It is difficult, if not impossible, to gauge this for an individual patient, and fatigue failure is therefore identified as being more likely to happen once a component reaches a threshold oxidation value.13 Although perhaps an oversimplification of the complex chemical-mechanical system, using such threshold values has helped researchers predict failure for long-duration retrievals.
Retrieved tibial bearings, both gamma air– and gamma barrier–sterilized, show articular surface fatigue failure. This fatigue failure is driven by the oxidation of ultra–high-molecular weight polyethylene (UHMWPE) and the resulting decrease in mechanical properties.7,55 It is important to note that the debris released from these types of failed surfaces is not necessarily biologically active. Analyses of the functional biologic activity of wear debris have demonstrated a much stronger biologic response to debris in the submicrometer size range.27,30 Debris from a device that fails through fatigue failure is orders of magnitude larger, often several millimeters in size.
The mechanism of debris generation has been well characterized in the literature.* It is generally understood that oxidation, and therefore reduction of mechanical properties, happens at a faster rate below the exposed surface of a tibial bearing. This is independent of whether the material is exposed to oxygen on the shelf or to oxidizing species in the body.7,13 In most knee designs, complete conformity is avoided to allow for femoral translation and rotation relative to the tibia. As a result, the nonconforming tibiofemoral contact sets up a stress environment in which the maximum contact stress is developed below the contact surface. Although the maximum subsurface oxidation and subsurface stress are not related to each other, they exist at approximately the same depth. This coincidental colocation allows for a mechanical situation in which cracks can nucleate and propagate to the surface from a depth of about 1 mm. If the cracks propagate immediately to the surface, 1-mm-diameter polyethylene pieces will spall off the device. If the cracks first propagate parallel to the surface before turning toward the articular region, even larger debris will be generated.
New UHMWPE formulations produced with antioxidants, without radiation, or with free radicals eliminated should be more resistant to oxidation and subsequent fatigue damage in vivo.5 Nonetheless, it will be important to monitor new bearing treatments continually at device retrieval to monitor the effects of long-term exposure to in vivo oxidizing species.
Adhesive and Abrasive Wear
Adhesive and abrasive wear is the process whereby small particles of bearing material are generated at the contact surface and become fine debris. This fine-particle generation is driven by abrasion and adhesion rather than by oxidative degradation. Adhesive and abrasive wear is an inevitable result of all intended articulations (Fig. 19-4); however, it can be easily masked by contact fatigue failure whereby large flakes of material are delaminated and removed.
Fine particulate debris from adhesive/abrasive wear historically has been associated with osteolytic response in hip arthroplasty. In hips, where adhesive/abrasive wear predominates, wear rates of 0.1 mm per year are thought to be sufficient to cause osteolysis.20,46,53 The principal mechanism leading to bearing failure in knees historically has been contact fatigue damage on the articular surface.7,22,29,36,60
However, because contact fatigue failure is addressed by efforts to minimize oxidative degradation of the polyethylene, more attention is being focused on the backside wear of knee inserts. It has been recognized that in modular knee bearings, backside wear mechanisms of abrasion and burnishing can produce small debris particles of the size implicated as the cause of osteolysis.44,53,61 Other studies have documented the effect of backside wear on the locking mechanisms for different modular knee systems.10,22,47,51
Adhesive and abrasive wear has been extensively studied over the last 3 decades, and a thorough discussion of the proposed mechanism is beyond the scope of this chapter. Numerous theories and explanations exist for the observed wear behavior; these tend to be scale-dependent,11 stress-dependent,4 or property-dependent.63 The most widely accepted theory of adhesive and abrasive wear is that the stresses induced during multidirectional articulation orient the polyethylene molecules and, through strain hardening, improve wear behavior in one direction.58 If stresses are applied to this oriented material through crossing motion, as observed in the hip and to a lesser extent in the knee, the directionally oriented polymer wears quickly because of what is termed a strain-softening effect.21,59
Cross linking has been known to reduce wear both in vitro and in vivo.34,39,64 It is theorized that the primary mechanism for this phenomenon is a reduction of the plastic deformation of the near-surface polyethylene, as well as the strengthening of intermolecular bonds. For example, Edidin and colleagues21 have shown that cross-linked polyethylene possesses a shallower plastic deformation zone. Muratoglu and associates41 have shown wear resistance to be highly correlated with cross link density, and Wang58 has modeled the phenomenon with similar results.
Adhesive and abrasive wear is also common on the backside of fixed bearing modular knees of many designs and types of locking mechanism (Fig. 19-5). With adhesive and abrasive wear, appearances can be deceiving, and this highlights the importance of the distinction between damage and wear (Fig. 19-6). It is therefore imperative that the researcher quantify material removal, rather than qualify the appearance of a surface.
Quantifying Wear
Retrieval studies have allowed the detailed assessment of all aspects of knee-bearing inserts and provide the opportunity for quantitative measurement of wear, provided that appropriate unworn reference surfaces are present and original dimensions are available. Knee inserts, by nature of their complex geometry, lack the intradevice reference frame provided by the spherical geometry of a hip implant. It has become clear that accurate and reliable wear data would be greatly enhanced by the inclusion of fiducial marks or wires embedded at known locations at manufacture of the bearings. In the absence of such references provided by the manufacturers, deformation in knee inserts is ambiguous. An instructive example of this is the presence of so-called pegs, or extrusions of polyethylene, on the backside surfaces of inserts (Fig. 19-7). They can be interpreted as cold flow of an unsupported area of thin polyethylene, which would not entail loss of material, or they can be interpreted as polyethylene remaining after the surrounding material was worn away. The two interpretations have different implications regarding debris and its clinical impact.
Both damage and wear on the articular (top side) of knee inserts have been documented in numerous studies. Cracking and delamination caused by oxidative fatigue of the polyethylene have been the primary modes of insert failure.7,13,42 It is evident that wear has occurred because material has been delaminated and removed in relatively large pieces. However, accurately quantifying the amount of material lost is challenging. Gravimetric methods are not useful with retrievals because reference weights are not known. Geometry on the top side surfaces of knee inserts is complex, consisting almost entirely of curved surfaces with sweeps and blends between them, usually with no reliable datum surface from which depths can be measured. Surface scanning and coordinate measuring machine metrology have been used with some success,43 but warping of the inserts from extended in vivo duration and from the process of retrieval can far exceed the dimensional changes caused by wear, especially those from adhesive and abrasive wear.
An early wear study on fixed-bearing knees of the Freeman-Swanson and Freeman-Samuelson designs (Protek AG, Münsingen-Bern, Switzerland) looked at 27 retrievals and reported an average wear penetration rate of 0.025 mm/yr.48 A study by Williams and coworkers62 in 1998 quantified wear in unicompartmental bearings using matched, never-implanted bearings as a reference. That study showed a wear rate of 0.55 mm/yr in gamma-sterilized bearings, which were oxidized and therefore underwent contact fatigue failure of the articular surface, compared with 0.09 mm/yr in ethylene oxide (EtO) sterilized bearings, which did not show any contact fatigue on their articular surfaces.
A study presented by Mayor and colleagues38 in 2005 quantified backside wear in a series of fixed-bearing Press-Fit Condylar (PFC, DePuy Orthopaedics, Warsaw, Ind) knees, and addressed the issue of reference dimensions by obtaining manufacturing drawings for all inserts in the study. Estimates of backside wear were made by comparing retrieval dimensions with corresponding design dimensions.
For the total series of PFC inserts, the mean estimated backside wear depth was 0.38 mm and the mean calculated linear wear rate was 0.05 mm/yr. When converted to volumetric wear estimates by incorporating the backside surface area, the mean estimated volume of backside wear of the series was 809 mm3 and the volumetric wear rate was 99 mm3/yr. It is important to recognize that on a knee-bearing insert, a penetration rate that in the context of hip wear would be considered low, acts over a very large area and therefore results in a debris load sufficient to be a contributing factor in osteolysis. An extension of this study was presented in 2009 and confirmed the wear rate in the series out to 220 devices of the same design.15
One consistent observation from the Mayor fixed-bearing knee series38 is that backside wear was at or near 0 at the anterior edge of the inserts and increased toward the posterior edge. In addition, backside wear was generally greater on the medial side of the inserts. The resulting wear pattern is shown to be a wear wedge (Fig. 19-8), which increases in thickness toward the posterior aspect of the insert and, in most cases, increases from the posterior lateral margin toward the posterior medial margin. This wear pattern is consistent with the following: (1) more weight is carried by the medial condyle; and (2) the greatest contact force occurs with the knee in flexion, therefore bearing more posteriorly.
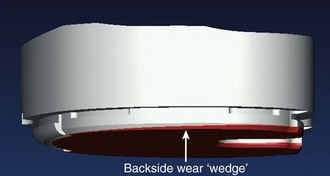
Figure 19-8 A study by Mayor and associates38 quantified backside wear and wear rate in a series of PFC fixed-bearing inserts and found the wear pattern to be a wedge, with little or no wear on the anterior margin, increasing toward the posterior edges. The mean estimated backside wear depth was 0.38 mm and wear rate was 0.05 mm/yr. It is important to recognize that on a knee bearing insert, a penetration rate that would be considered low for a hip acts over a very large area and therefore can result in a debris load sufficient to be a contributing factor in osteolysis.
A notably different wear pattern was observed in a series of NexGen knees retrievals (Zimmer, Warsaw, Ind) as reported by Van Citters and associates.56 In that study, the wear pattern was much more variable than for the PFC series, but the greatest dimensional changes were generally seen in the anterior and medial areas of the inserts. Although the PFC and NexGen knees inserts are similar in their overall plan view shape and peripheral capture mechanism for the insert, the evidence suggests that other factors play the most important roles in backside wear and wear pattern. Wear is a function of motion, load, bearing material properties, and lubrication. Differences in motion pattern and load and their relative timing in a gait cycle or flexion activity can be the determining factors in where and how much backside wear occurs.
Wear of the backside surface of knee inserts in modular fixed-bearings can affect the efficacy of the mechanisms whereby the inserts lock onto the base plates.10,23,47,51 Many modular knee designs use a peripheral capture mechanism that follows the curve of the anterior aspect of the tibial plateau. This locking geometry is not well suited to resist the torque applied to the insert by the femur by rotation under loaded flexion, and neither is a centrally located locking mechanism very effective at resisting tibiofemoral rotation. As backside wear progresses, the tightness of fit of the capture mechanisms is compromised. As a result, the locking geometry becomes less effective and allows more relative motion, which in turn promotes further wear. This progression of motion and wear is evident with even qualitative visual assessment of knees of a variety of designs (see Fig. 19-5). Efforts to devise locking mechanisms that resist torque better and geometries that are not progressively degraded by the observed backside wear patterns would appear to be warranted.
Factors Involved in Knee Wear
Conformity
A notable observation taken from the aforementioned PFC study is that the backside wear has a strong dependence on the articular geometry of the insert. One of the three bearing geometries of the implant series is relatively flat in the anteroposterior (AP) plane and that geometry stands apart, with significantly lower wear penetration and wear rate than the other two more conforming insert designs (Fig. 19-9). One implication of this finding is that torque transmitted to the insert by the femoral component can be a primary driver of backside motion and wear of the insert.
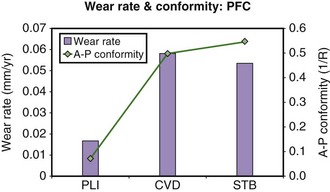
Figure 19-9 The PFC fixed-bearing wear study by Mayor and coworkers38 indicated that backside wear has a strong dependence on the different articular geometries of the inserts. Posterior lipped inserts (PLI) have a relatively flat articular geometry in the AP plane; the curved (CVD) and stabilized (STB) geometries are much higher in AP conformity.
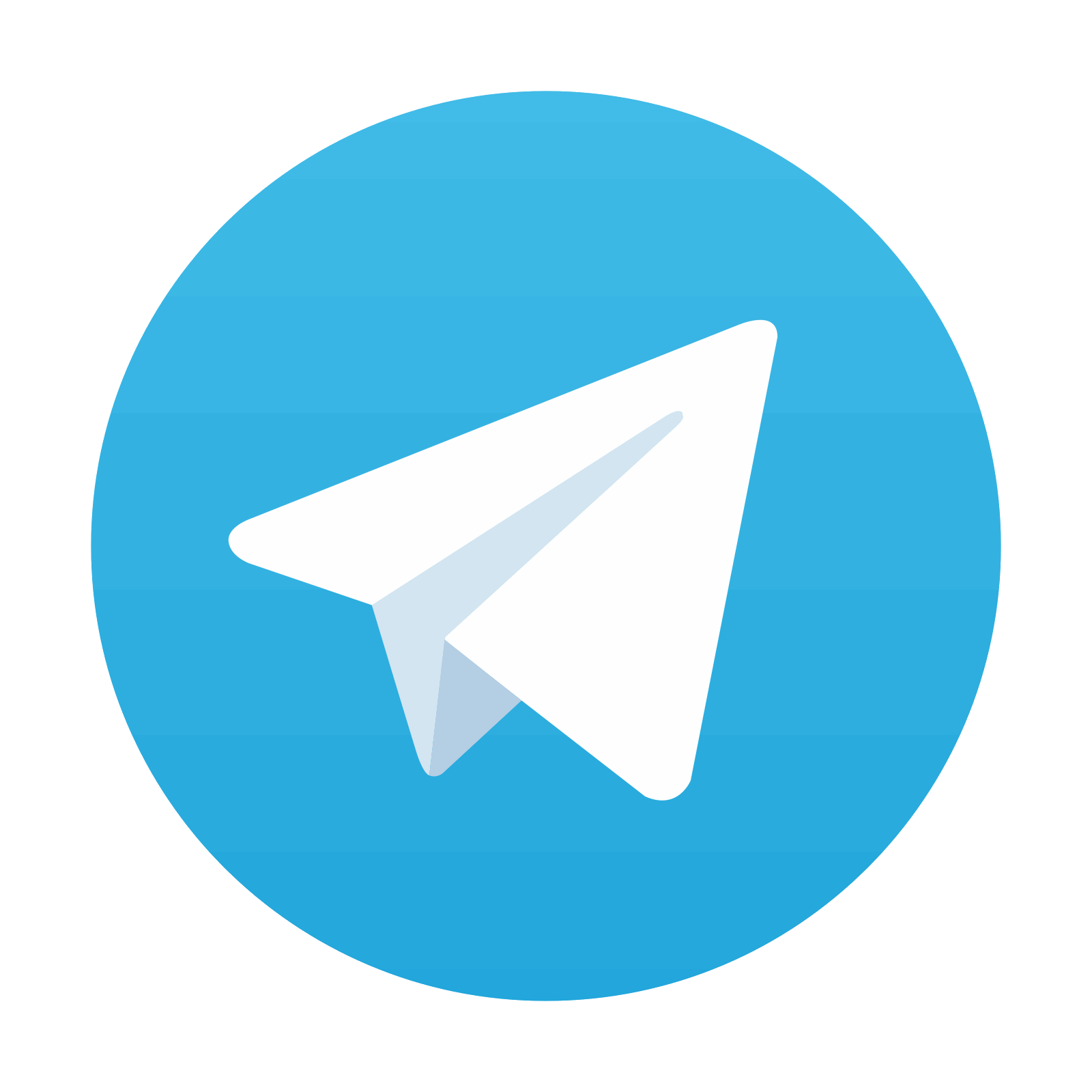
Stay updated, free articles. Join our Telegram channel

Full access? Get Clinical Tree
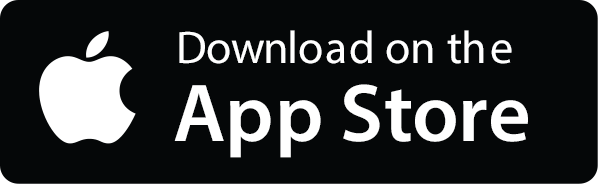
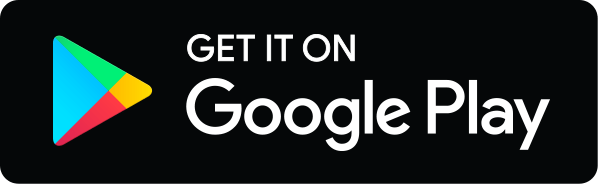