Fig. 11.1
Picture showing the Motion Monitor with the transmitter and semicircular board, which can be placed in the sagittal plane and scapular plane, functioning as a reference for the patients to follow during the measurements [13]
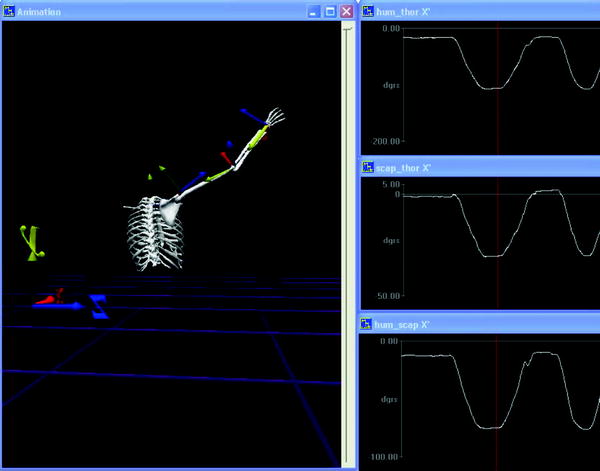
Fig. 11.2
Motion Monitor image of one of the patients, with the real-time visualization of the virtually constructed skeleton with the x-,y-, and z-axis of all the joints, together with the TH, ST, and GH motion in the scapular plane. This image is a still of a video, where the red line in the right-sided picture is moving from left to right during elevation of the arm, showing the contribution of all 3 elements (TH, ST, and GH) at every moment. J Shoulder Elbow Surg 2014 (Epub ahead of print)
Recently, this system has been used in several studies on shoulder arthroplasty, describing the motion pattern of patients with hemiarthroplasties [12], as well as patients with a total shoulder arthroplasty (TSA) and reverse shoulder arthroplasty (RSA) [13–16], providing better insight into the motion patterns of shoulders with these types of prosthesis.
The Kinematic Difference Between TSA and RSA
Both RSA and TSA effectively decrease pain and improve clinical outcome [17–25]. However, the indications for these two different procedures and the biomechanical properties of the two prostheses vary greatly, which likely will influence the kinematics of the shoulder girdle. A recent comparison of RSA and TSA patients by Puskas et al. [26] indeed demonstrated a better postoperative active ROM in TSA patients, but those ROM measurements were performed using a digital goniometer on a video-recorded physical examination, thus only measuring the TH motion. Using the “Flock of Birds” with Motion Monitor software, Bergmann et al. [14] show a better passive than active ROM in their group of RSA patients, in both TH and GH motion with similar differences in ROM. Using the same measurement method, comparable findings were measured by Veeger et al. [12] using anatomical shoulder replacements consisting of half the patients receiving hemiarthroplasty. By measuring both active and passive ROM and comparing primary RSA and TSA patients, it might be possible to find an explanation for the differences between the two types of prostheses.
We recently compared these two types of arthroplasty, using the “Flock of Birds”. Sixteen patients (10 women and 6 men) with a reverse shoulder prosthesis (Tornier®; Edina, MN, USA) (3 bilaterally, total of 19 shoulders) with cuff tear arthropathy as an indication, operated through a deltopectoral approach, were measured. The average time between surgery and measurement was 22 ± 10 months (range 6–41 months) and the mean age of the patients was 69 ± 8 years (58–84 years). The motion pattern of this group was compared to that of 17 consecutive patients (13 women and 4 men) with a total shoulder prosthesis (Aequalis, Tornier®; Edina, MN, USA) (3 bilaterally, total of 20 shoulders) with primary osteoarthritis as the indication. The average time between surgery and measurement for this group was 33 ± 18 months (12–87) with a mean age of 72 ± 10 years (53–87 years) and these patients were also operated through a deltopectoral approach. This comparative study demonstrated, like the study by Puskas et al. [26], that TSA patients have a significantly better postoperative active ROM than RSA patients (* in Table 11.1), 16° more TH motion and 8° more ST motion in the sagittal plane (p = 0.01 and p = 0.018, respectively) and 17° more TH motion and 15° more GH motion (p = 0.016 and p = 0.01) in the scapular plane. However, for the passive ROM, only a difference in internal rotation of 18° in favor of the RSA cases (p = 0.049) (* in Table 11.1) was demonstrated. Considering the differences in passive and active ROM within the types of prostheses, RSA had a significantly larger passive than active TH and GH ROM in both planes as well as a larger passive external rotation. TSA only demonstrated a larger passive than active TH and GH ROM in the sagittal plane and larger passive external and internal rotations, but no significant difference in active versus passive TH and GH motion in the scapular plane (Table 11.1). The differences between passive and active ROM for RSA in both planes (sagittal and scapular), and the difference only in the sagittal plane for TSA suggest that both prostheses do not mimic normal shoulder motion. However, the absence of a difference between the active and passive motion in the scapular plane in the TSA patients suggests that the TSAs act more anatomically in the so-called zero position [27] and can better utilize the possible GH motion of the prosthesis in this plane, compared to the RSA patients (Table 11.1). Although the prostheses behaved differently, the GH to ST ratios, in contrast, demonstrated similar patterns for both TSA as RSA without significant differences between the ratios (Table 11.2).
Table 11.1
The maximal active and passive joint angles (degrees) for the 19 RSA and 20 TSA shoulders during the elevation and rotation tasks
Plane of movement | Shoulder motion | Movement | RSA | Paired t-test (active vs. passive RSA) | TSA | Paired t-test (active vs. passive TSA) | General linear model | ||||
---|---|---|---|---|---|---|---|---|---|---|---|
Active ROM | Passive ROM | Active ROM | Passive ROM | Mode | Group | ![]() | |||||
Sagittal | Thoracohumeral | Forward flexion | 93 ± 21 (44 to 127) | 113 ± 16 (85 to 154) | <0.001 | 109 ± 18 (59 to 131)* | 117 ± 16 (69 to 135) | <0.001 | <0.001 | 0.062 | 0.001 |
Glenohumeral | 71 ± 18 (41 to 108) | 87 ± 16 (62 to 116) | <0.001 | 80 ± 17 (34 to 102) | 87 ± 17 (42 to 113) | <0.001 | <0.001 | 0.388 | 0.003 | ||
Scapulothoracic | 30 ± 11 (1 to 43) | 32 ± 10 (3 to 43) | 0.124 | 38 ± 11 (23 to 67)* | 38 ± 11 (19 to 62) | 0.848 | 0.139 | 0.03 | 0.197 | ||
Scapular | Thoracohumeral | Abduction | 93 ± 22 (41 to 139) | 108 ± 21 (62 to 152) | <0.001 | 110 ± 19 (67 to 141)* | 112 ± 17 (71 to 135) | 0.2 | <0.001 | 0.097 | <0.001 |
Glenohumeral | 64 ± 15 (45 to 95) | 76 ± 18 (55 to 111) | <0.001 | 79 ± 19 (34 to 99)* | 79 ± 18 (37 to 106) | 0.982 | <0.001 | 0.118 | <0.001 | ||
Scapulothoracic | 33 ± 15 (0 to 52) | 36 ± 14 (0 to 55) | 0.096 | 39 ± 13 (16 to 67) | 37 ± 11 (22 to 61) | 0.18 | 0.769 | 0.399 | 0.032 | ||
Scapular, arm 90° abduction | External rotation | Rotation | −31 ± 25 (5 to −93) | −47 ± 32 (34 to −94) | 0.01 | −42 ± 12 (−23 to −60) | −49 ± 16 (−21 to −80) | 0.018 | <0.001 | 0.379 | 0.108 |
Internal rotation | Rotation | 21 ± 24 (−35 to 60) | 26 ± 37 (−54 to 90) | 0.501 | 19 ± 18 (−11 to 58) | 8 ± 17 (−20 to 40)* | 0.003 | 0.428 | 0.146 | 0.043 |
Table 11.2
Ratio GH/ST for the 19 RSA and 20 TSA shoulders for the active and passive elevation tasks in both planes
Plane of movement | Movement | RSA | TSA | One-way ANOVA RSA versus TSA |
---|---|---|---|---|
Sagittal | Active | 1.34 ± 0.3:1 | 1.41 ± 0.3:1 | 0.437 |
Passive | 1.32 ± 0.2:1 | 1.38 ± 0.2:1 | 0.423 | |
Scapular | Active | 1.49 ± 0.3:1 | 1.44 ± 0.3:1 | 0.694 |
Passive | 1.45 ± 0.3:1 | 1.48 ± 0.3:1 | 0.761 |
The Influence of Revision Surgery
When the reverse prosthesis is used in revision surgery, the improvement of function is inferior, compared to primary placed prosthesis, with a mean active elevation of approximately 70° [28] and it is also associated with a higher rate of complications [22]. This improvement in active elevation mostly occurs in the TH plane. For a better understanding of the influence of the revision surgery on the motion pattern in the different planes (GH or ST), we compared 19 primary RSA’s with 16 cases which were used in revision surgery after a failed primary placed hemi- or total shoulder prosthesis with a non-functional rotator cuff [13]. This comparison demonstrated that in the sagittal plane there was no significant difference between the primary and revision patients (93 ± 21° vs. 78 ± 36°, respectively, p = 0.157) for active elevation and in the scapular plane primary patients tended to reach a higher active abduction than the revision patients (93 ± 22° vs. 73 ± 34°, respectively, p = 0.052). However, considering the two different parts (GH and ST) of the elevation of the arm, the mean GH motion differed significantly between the primary and revision patients, in both the sagittal (71 ± 18° vs. 53 ± 26°, respectively, p = 0.025) and scapular plane (64 ± 15° vs. 46 ± 24°, p = 0.013). Therefore, primary patients had both higher forward flexion and abduction than revision patients in the GH joint. This difference was not seen in the mean scapulothoracic motion (Table 11.3). For external rotation, patients with a primary prosthesis had a significantly higher active external rotation than revision patients (−31 ± 25° vs. −13 ± 16°, p = 0.015). A possible explanation for this difference in motion between primary placed prostheses and revision cases could be adhesions or scar tissue around the glenohumeral joint from previous procedures. No axillary nerve palsies were observed in the patient groups, but the remaining quality of the deltoid muscle may have been inferior after previous operations; also the strength and function of the remaining rotator cuff muscles might have been better in primary cases.
Table 11.3
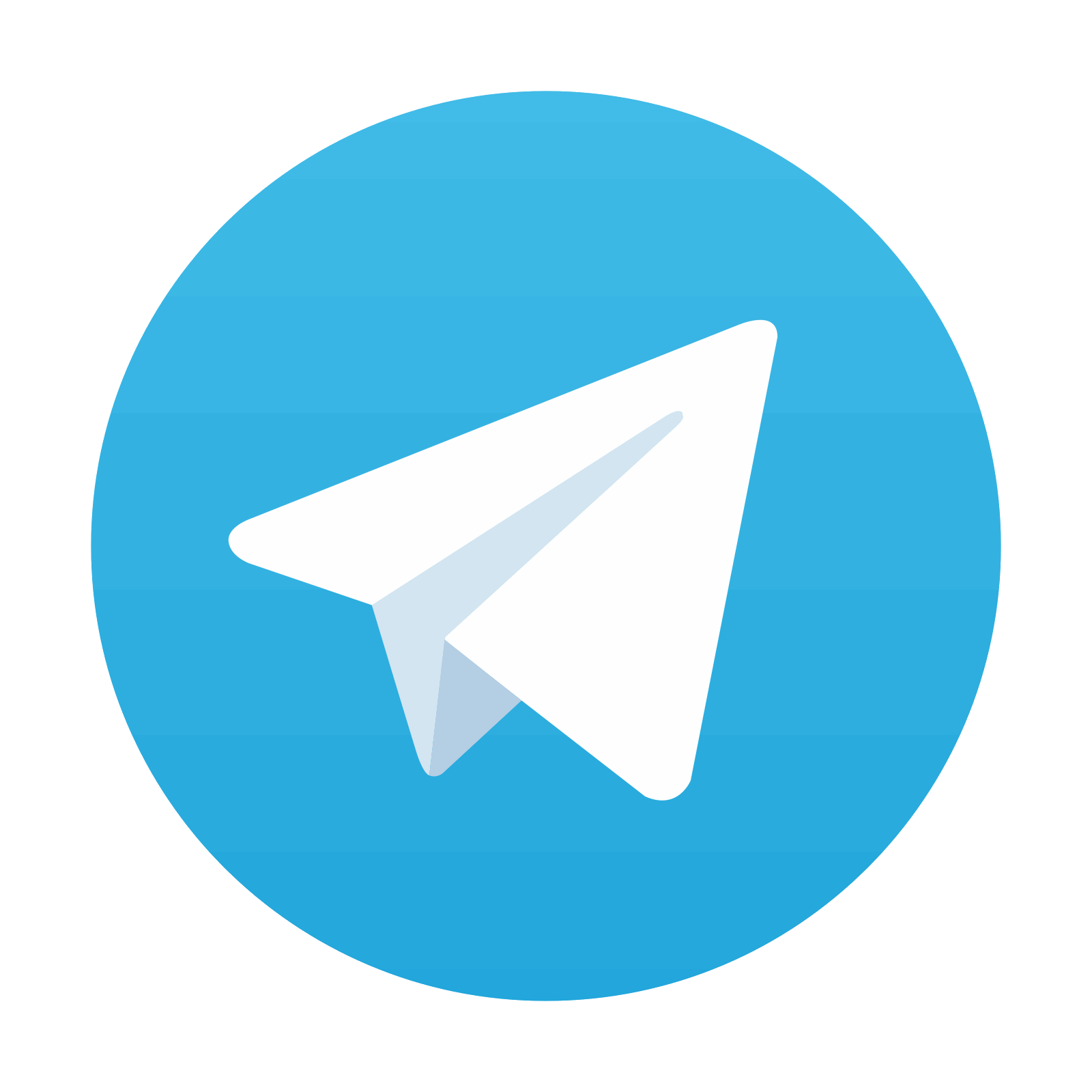
Maximal active joint angles (degrees) for 35 shoulders during the elevation and rotation tasks [13]
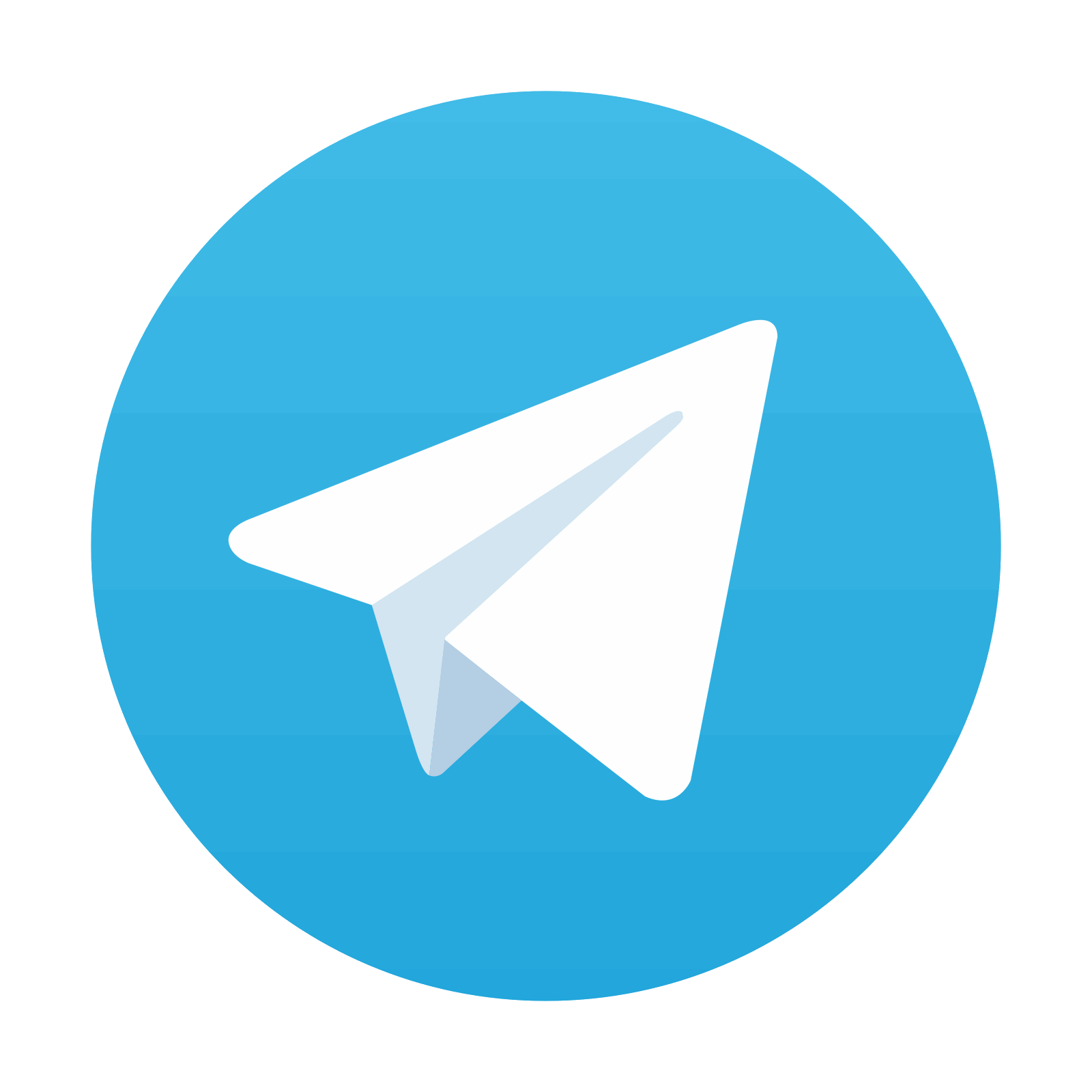
Stay updated, free articles. Join our Telegram channel

Full access? Get Clinical Tree
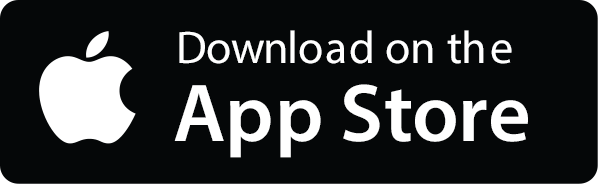
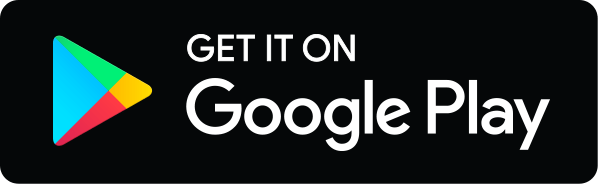
