Fig. 22.1
Bilateral standing X-ray of a 65-year-old female patient. Mild to moderate radiological findings of osteoarthritis were recognized
A 65-year-old female patient approached the outpatient clinic with symptoms of knee pain. After physical examination and X-ray studies, the physician prescribed non-load-bearing exercise and a dietary supplement containing glucosamine and chondroitin sulfate. The patient had to take the supplement for 3 months. At her control visit, when she reported partial relief of her pain symptoms, an intra-articular hyaluronan injection was proposed. While under treatment with exercise, glucosamine and chondroitin sulfate supplement, and intra-articular injections, the patient was followed up for 6 years until her joint replacement surgery was undertaken.
Oral glucosamine is a dietary supplement used to relieve pain in osteoarthritis (OA). Prescribed as a medicine in some European countries, controlled clinical trials indicate that this disease-modifying supplement is partially effective in the knee and hand but not in all types of OA [2]. More important is the patient’s age, the disease severity, and the phenotype of the patient. Obese and severely osteoarthritic patients benefit less from this supplement. Oral glucosamine is not advised in diabetic patients although insulin resistance or endothelial dysfunction does not occur in healthy individuals [3]. Patients on warfarin (Coumadin) also should not use oral glucosamine. It should be noted that an updated intervention review [4] failed to show any benefit of glucosamine for pain, function, and stiffness scales though one formulation had better results than placebo. Another study found no evidence that oral herbal therapies improve joint structure and prevent joint space narrowing [5].
An algorithm recommended for the management of knee OA was recently defined [6]. Prescription of glucosamine sulfate and/or chondroitin sulfate with or without paracetamol is recommended at step one as background treatment according to this protocol. Intra-articular hyaluronan injection is advised at step two in persistently symptomatic patients in the same protocol. According to the recent OA Research Society International (OARSI) guidelines [7], the symptomatic efficacy of this type of supplementation is uncertain. American Academy of Orthopaedic Surgeons (AAOS) guidelines concluded that these treatments do not provide evidence-based outcomes [8].
Intra-articular hyaluronan injection, which is also defined as viscosupplementation, decreases pain and improves function in knee OA [9].
Structure, Function, and Repair
The nineteenth century humanist, poet, essayist, and journalist Walter Whitman observed the way a joint of a finger is more complex than the most intricate machinery. Most orthopedic surgeons will concur with this observation. Artificial joints of today can partially fulfill requirements of the joints for a determined duration.
Joint cartilage is a highly specialized connective tissue tolerating broad range of motion between long bones. It generates a very low frictional surface and presents lubrication properties for millions of cycles through decades. This macroscopically shiny, light blue or opal tissue at young ages has distinctive structural features. Its thickness, cellular volume, extracellular matrix, and mechanical properties may vary between species, at different joints of the same kind and at different locations of the same joint [10]. The thickness of human knee joint cartilage is less than 10 mm. Six to eight times body weight is absorbed by this structure in a jogging human. Such an intensive load is very well distributed and absorbed in such a thin and small surface area. Macroscopically, the surface of joint cartilage is smooth and durable to deformation. Microscopically, this multilayered structure contains (a) one type of cells named chondrocyte, (b) is mostly of extracellular matrix, and (c) lacks blood and lymph vessels and nerve endings (Fig. 22.2) [11]. Chondrocytes are nourished from synovial fluid. They almost always metabolize through the anaerobic pathway and therefore have limited regeneration capacity. Their primary function is to maintain or restore the extracellular matrix. Chondrocyte function deteriorates with aging leading to cartilage degeneration. Thickness of the cartilage decreases and its molecular biologic structure weakens. The weakened microscopic molecular structure leads to abnormal fluid homeostasis depriving mechanical functions. Oriented fibers of joint cartilage act such as a natural sponge. Synovial fluid flows out of the sponge rapidly at initial loading. Micro- and nanopores of the sponge lock over each other when compressive loading continues and increases. A certain amount of synovial fluid is entrapped in the pores of the spongelike structure of the cartilage even under excessive loads allowing it to properly function. Synovial fluid may not be attained in joint cartilage when this fiber structure of the extracellular matrix is disrupted, and chondrocytes are primarily responsible for regulating this homeostasis. The number and function of chondrocytes decrease with age leading to osteoarthritis (OA). Joint cartilage and subchondral bone degenerate in OA. Pain, limitation of joint motion, and deformity are its clinical findings. Fifty percent of practitioner visits of 65+-year-old patients are due to OA [12].
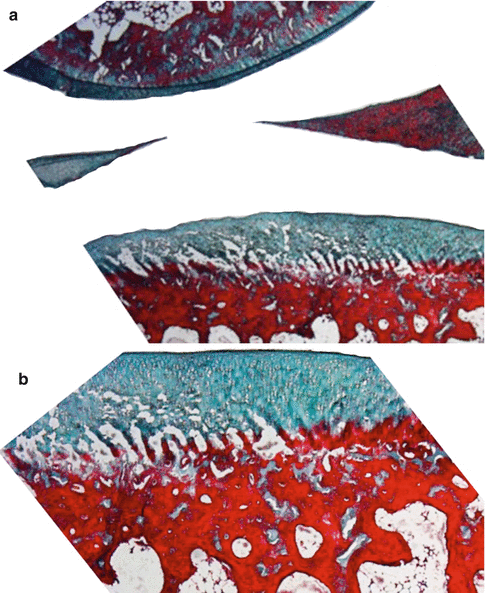
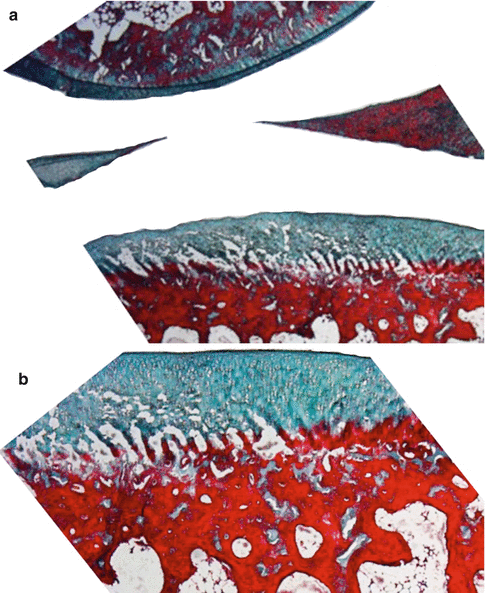
Fig. 22.2
Normal knee joint cartilage and meniscus of rabbit (a) Masson’s trichrome 10× and (b) Masson’s trichrome 40×
Ratio of chondrocyte to extracellular matrix is 1.65 % and 2.6 % at the superficial and middle–deep zones, respectively [13]. The superficial zone is also named as the fibrous or tangential zone. Superficial zone chondrocytes are fibroblast-like flattened cells aligned in the plane of the articular surface. Middle and deep zone chondrocytes function in their lacunae. Molecular mechanism of how chondrocytes control cartilage repair and/or regeneration is less known [14–16]. Regeneration and repair alter with age [17]. Proteolysis, glycation, and calcification are frequent features of aging. Cell density decreases. Abnormal secretory profiles and anabolic responses lead to biomechanical dysfunction and tissue destruction [17].
Eighty percent of wet weight of articular cartilage is of the synovial fluid. Synovial fluid and extracellular matrix determine the phenotype and endurance of joint cartilage. Synovial fluid containment capacity of joint cartilage is dependent on the macromolecule named aggrecan and the electrolyte containment of the synovial fluid. Collagen, proteoglycan, and glycoproteins establish 60 %, 25 %, and 15 % of the dry weight of joint cartilage, respectively. Collagen fibers are aligned longitudinally and are intense under the joint surface at the superficial zone. Resistance to tensional forces is generated by these fibers. In the middle and deep zones, collagen fibers keep chondrocytes in their lacunae. Proteoglycans and glycoproteins determine the synovial fluid containment of the cartilage. Type IX and XI collagens cross-link type II collagen and are responsible of cartilage integrity. Ninety to 95 % of joint cartilage structure is of type II collagen. Half-life of collagen is calculated as 3 months. Type VI collagen is mostly located around chondrocytes. Type X collagen is largely located near calcified and hypertrophic cartilage zones. Type X collagen is assumed to function at the calcification process of joint cartilage (Fig. 22.3).
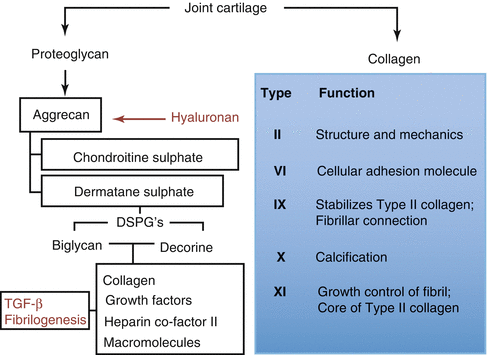
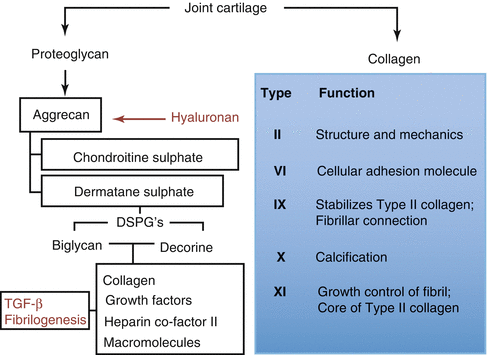
Fig. 22.3
Proteoglycan and collagen structure of joint cartilage
Proteoglycan structure is of several glycosaminoglycan chains attached to a central protein chain. Glycosaminoglycan chains are of repeating disaccharides that contain amino glucose. Each disaccharide unit contains a negatively charged carboxyl or sulfate ring. Hyaluronic acid, chondroitin sulfate, keratan sulfate, and dermatan sulfate are major glycosaminoglycans. Keratan sulfate to chondroitin sulfate ratio increases with age as chondroitin sulfate degrades between globular domains two and three [18]. The big molecules of the 100 mg/ml dense proteoglycan are named as aggrecan. Decorin, biglycan, and fibromodulin are smaller proteoglycans. Type IX collagen is named as a glycosylated protein due to its glycosaminoglycan content (Fig. 22.4).
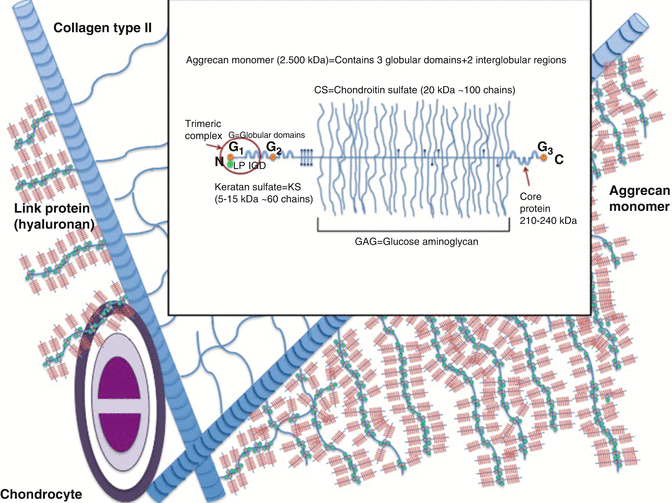
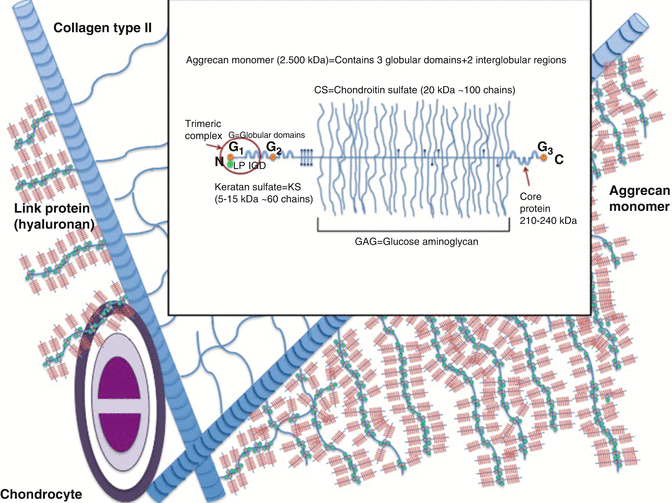
Fig. 22.4
Structure of aggrecan
Keratan and chondroitin sulfates construct aggrecan structure [19]. Aggrecans fill 90 % of joint cartilages’ interfibrillar space. Decorin, biglycan, and fibromodulin contain one, two, and several sulfate chains, respectively. These fill up the remaining interfibrillar space from the aggrecan. Aggrecan is attached to hyaluronic acid by non-covalent bindings. Aggrecan and hyaluronic acid are further attached to each other by connecting proteins. Aggrecan augments all proteoglycans, glycosaminoglycans, and collagen. Hyaluronan that expands from 100 to 10,000 nm establishes the central skeleton allowing about 300 aggrecan attachment (Fig. 22.4). Small proteoglycans that do not attach to other proteins maintain chondrocyte homeostasis. Fibromodulin and decorin stabilize type II collagen and biglycan attaches to type VI collagen. Small proteoglycans furthermore regulate repair by attaching to transforming growth factor (TGF)-β. They also control degeneration triggering enzymes. Proteoglycans and connecting proteins are synthesized from the endoplasmic reticulum, whereas hyaluronan is assumed to be synthesized from the cell membrane.
Core protein of the aggrecan contains a single genetic code. Its three-dimensional structure is of three globular proteins and two other extensions. This structure is different in other species. The aggrecan monomer connects to the central hyaluronic acid skeleton through its N terminal. G1, G2, and G3 globular connection points separate this core protein to E1 and E2 sections. Aggrecan terminates at the G3 globular connection that is also named as the C terminal. The E1 and E2 sections are about 21 nm and 260 nm, respectively. Most of keratan sulfate and all of chondroitin sulfate are attached to the core protein E2 section. Globular connection points are of folding immunoglobulins and disulfide double loop repeating chains. They present CD44 staining and are immunogenic [20]. The three-dimensional G1 domain that is at the N terminal, which connects aggrecan to the central hyaluronic acid skeleton, is a complex structure [21]. G1 domain is stable for heat and proteinases. A disintegrin and metalloproteinase with thrombospondin motifs (ADAMTS) may catabolize this naturally stable trimeric complex and cause degeneration [22, 23]. ADAMTS are potential therapeutic targets for OA. Syndecans that fix ADAMTS to the cell membrane disintegrate by the inflammatory molecule interleukin-1 and lead to cartilage breakdown at synovial inflammation [24]. The G3 globular connection that is of ten cysteine molecules is defined in only 10–50 % of joint cartilage samples. The function of G3 globular connection is not clearly defined. G3 globular connection is assumed to regulate matrix organization, synthesis, intracellular translocation, and glycosylation.
Carbohydrate distribution of cartilage proteoglycans varies between chondroitin sulfate, keratan sulfate, and O-linked and N-linked oligosaccharides. Dimensions, quantity, pathway of sulfatization, and electrical charges of these proteoglycans change with age. Non-collagenous proteins and glycoproteins are oligosaccharides and monosaccharides. Ancorin CII and cartilage oligomeric matrix protein (COMP) connects chondrocytes to the extracellular matrix. COMP is specific to joint cartilage and increases in serum and synovial fluid with degradation [25]. Load-bearing exercise increases serum COMP levels [26]. Functions of non-collagenous proteins fibronectin and tenascin are still searched.
Joint Cartilage Layers
Superficial, intermediate, radiated, and calcified interconnecting zones of joint cartilage are defined (Fig. 22.5). Layers have different functions. Phenotype and metabolism of chondrocytes of these layers are different than each other. Each group of chondrocytes of a specific layer synthesizes different molecules. The thin fibril containing surface layer named as lamina splendens is constructed of tiny polysaccharides and contains almost no chondrocytes. Fibrocyte-like chondrocytes are aligned parallel to the joint surface just below the lamina splendens. The collagen content is high and the proteoglycan content is low of this layer. Lamina splendens controls synovial fluid in- and outflow. Penetration of toxic molecules and immune reactive cells of the synovium to the deeper cartilage layers is prevented by this lamina. Fibronectin and synovial fluid content of this layer is higher than the other layers. This layer is durable in shear force. Injury at the surface layer triggers inflammation and initiates immune response. Mechanical durability changes and deterioration or degeneration occurs after injury.
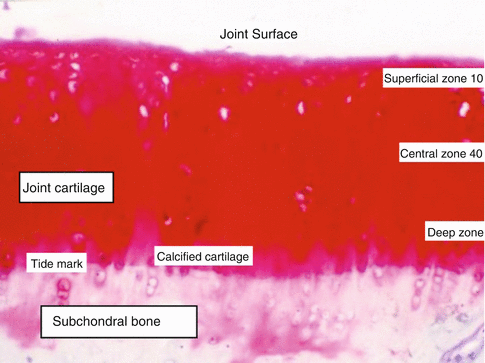
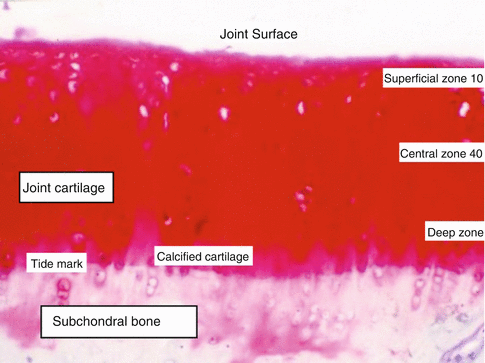
Fig. 22.5
Layers of joint cartilage
Endoplasmic reticulum and Golgi organ complex of the intermediate layer chondrocytes are better developed than the surface layer chondrocytes. Chondrocytes of the intermediate layer are spherical, and the collagen fibers are thicker than the surface layer. Some of these thick collagen fibers may reach the tidemark that separates subchondral bone and joint cartilage. Synovial fluid and collagen content of this layer is lower and the proteoglycan ratio is higher. Orientation of chondrocytes is perpendicular to the surface and their phenotype converts into oval. The intermediate layer due to the orientation of its fibers absorbs shear stress generated at joint movement.
The lower calcified cartilage layer is thin and separates joint cartilage from the subchondral bone. Chondrocyte content of this layer is lower compared to other layers. Active molecule synthesizing organelles of chondrocytes of this layer is less developed indicating lower molecular activity. Some chondrocytes of this layer are isolated in calcified lacunae. This layer regulates blood and other molecule penetration into the cartilage.
Extracellular Matrix and Chondrocyte Interaction
Homeostasis of the extracellular matrix structure and function is regulated and maintained by chondrocytes. Nutrients brought to the chondrocytes by the synovial fluid trespass the extracellular matrix. Some regulatory molecules, cytokines, and growth factors are stored in the extracellular matrix. Chondrocytes on the other hand synthesize the macromolecules of the extracellular matrix lifelong. Chondrocytes maintain the integrity of the extracellular matrix although less is known on the anabolic and catabolic metabolic pathways of maintenance. Inflammatory regulator interleukin-1 (Il-1) triggers extracellular matrix metaloproteases and increases catabolic macromolecules. Il-1 also affects transcription of extracellular matrix macromolecules. Insulin-like growth factor (IGF) and transforming growth factor beta (TGF-β) cytokines reduce catabolic activity by stimulating chondrocyte growth and extracellular matrix synthesis. Autocrine and paracrine cytokines act by binding chondrocyte membrane receptors. Catabolic cascade of the extracellular matrix is complex. Various cytokines including Il-1, stromelysin, aggrecanase, plasmin, and collagenase are involved in this cascade. Prostaglandin, TGF-β, tumor necrosis factor (TNF), metalloproteinase inhibitors, and tissue plasminogen stimulator and inhibitor turn the cascade on and off. Inhibition of Il-1 and TNF is investigated for treatment. Research on Il-6, Il-15, Il-17, Il-18, Il-21, leukemia inhibitory factor, and Il-8 chemokine is still ongoing [27].
Autocrine Regulation of Joint Cartilage
Chondrocytes are mainly isolated in their lacunae and autocrine molecules act less on these cells. Mechanical loads on the extracellular matrix improve autocrine stimulation. Effects of bone morphogenic protein (BMP), epidermal growth factor (EGF), platelet-derived growth factor (PDGF), parathyroid hormone-related protein (PTHrP), fibroblast growth factor (FGF), TGF-β, and IGF on chondrocytes are evaluated under mechanical loading. These hematopoietic cytokines are regenerative in most tissues; however, their molecular action mechanism in avascular joint cartilage is a research area. TNF-α, interleukins, and colony-stimulating factor (CSF) like cytokine levels increase after cartilage trauma and degenerative joint diseases.
IGF-1, TGF-β family, BMPs, and FGS family are most important regulatory autocrine growth factors.
(a)
IGF: IGF-1 is released from the cartilage with systematic growth factor secretion. This is an anabolizing factor. IGF-2 is also isolated from joint cartilage and has similar anabolic effects. IFG-1 stimulates proteoglycan synthesis and chondrocyte proliferation. Metalloproteinase production is triggered by IGF-1. Metalloproteinases catabolize proteoglycans. Type II collagen synthesis is also stimulated by this mediator. Endochondral ossification of the physis is regulated by IGF-1. Growth hormone in acromegalia acts through this factor. Mitogenic activity of EGF and PDGF on joint cartilage is secondary to IGF-1.
(b)
TGF-β: The TGF family has five isoforms. These polypeptides convert into their active form when they are released into the extracellular matrix. Temperature and pH of the environment determine TGF activity. TGF-β stimulates the DNA of chondrocytes and proteoglycan synthesis. TGF-β prevents extracellular matrix degeneration through its anti-catabolic activity. Proteoglycan synthesis increased, and inflammatory synovitis developed after intra-articular injection of TGF-β. TGF-β is accepted as a joint cartilage stimulant; however, it has adverse effects on synovial tissue. Effects of intra-articular PDGF injection have little effects on joint cartilage and synovium.
(c)
FGF: Fibroblast growth factor (FGF) is another important signaling molecule of the joint cartilage. FGF-2 connects to heparin-like molecules frequently. FGF-2 is mitogenic to chondrocytes and synergistic with IGF-1. Opposite to IGF-1 and TGF-β, proteoglycan synthesis is suppressed with this molecule. It stimulates chondral growth when injected into the joint space of young rats. TGF-β secretion is stimulated by FGF. This molecule has a homing effect on mesenchymal stem cells at defect sites and stimulates cartilage restoration.
(d)
BMP: Bone morphogenic proteins are a subgroup of the TGF-β family. They stimulate chondrogenesis of mesenchymal stem cells. All stages of endochondral ossification are observed when BMP is injected ectopically. BMP-1 is proteolytic on the extracellular matrix of joint cartilage. BMPs 2, 3, and 4 stimulate proteoglycan synthesis of joint cartilage. BMP-6 is secreted from hypertrophic chondrocytes [28].
Biomechanics of Joint Cartilage
Joint cartilage is almost always under static and dynamic loads. Physiologic loads on joint cartilage can rise up to 15–20 MPa while climbing stairs. Such load on a very short duration applies 1–3 % compression to the joint cartilage. Static loads up to 5–30 min affect 35–45 % of joint cartilage. Structural integrity of the extracellular matrix defines resistance to compression, tension, and sheer forces. Chondrocytes regulate integrity of synthesis and organization of proteoglycans, collagen, glycoproteins, and other molecules precisely to protect extracellular matrix durability. Up to 0.5 and 1 MPa compression, 0.25 MPa sheer and 10–50 MPa tension forces maintain joint cartilage homeostasis. Collagen fibers resist sheer and tension forces, while aggrecan constituted of glycosaminoglycan molecules resists to compression and synovial fluid flow into the tissue. Electrostatic and osmotic swelling is aggrecan related and determines 50 % of compressive load balance.
Mechanical loads on joint cartilage are important for the metabolic activity, cellular integrity, and synthesis of prostaglandin and nitric oxide like inflammatory mediator turnover in joint cartilage [29]. Immobilization on the other hand decreases extracellular matrix production and causes softening of the tissue [30]. Aggrecan content increases at the site where load increases. Excessive exercise causes cartilage destruction. Acute and/or chronic injury or excessive compression destructs joint cartilage. In vitro experiments revealed that static compression decreased extracellular matrix production reversibly. Intermittent compression and/or hydrostatic pressure on the other hand increase core protein synthesis of aggrecan and all other joint cartilage related proteins. Mechanical forces at around the microenvironment of chondrocyte stimulate production and/or destruction of the nearby extracellular matrix macromolecules. Chondrocytes react to mechanical stimulation. Newly synthesized proteoglycan, collagen, and other molecules’ quality and functionality are affected by mechanical stimulation in line with the extracellular matrix. Extracellular matrix that cannot resist to mechanical loads will be injured.
Resistance to synovial fluid flow determines resistance to pressure under loads. Behavior of chondrocytes under physiologic loads and how they are stimulated to maintain tissue integrity is a field of research. Chondrocytes function in an osmotic and mechanically active microenvironment. Chondrocyte metabolism is exerted by diffusion, and physiologic mechanical stimulation assists anabolism and catabolism. Chondrocytes respond directly to environmental changes. Physiologic loads differentiate cell into chondrocytes from embryogenesis to adulthood. Immobilization on the other hand causes severe proteoglycan lose and ends up with structural deformity [30].
Mechanical loads that affect joint cartilage are quite complex. Compressive loads on one site generate tension and sheer stresses at another site of joint cartilage. Hydrostatic pressure alterations also change chondrocyte behavior. Research on chondrocyte signaling molecules at combined mechanic and hydrostatic loading may elaborate new information. Physiologic increase in hydrostatic pressure leads to the increase of anabolism of chondrocytes in a few seconds. Static or dynamic loading of joint cartilage increases complexity. Transport of nutrients and cytokines is limited under static loads. Intercellular ionic state also changes with static loads. Sodium concentration increases and pH decreases outside the chondrocyte under static axial load. This change adversely affects extracellular matrix production. Combined increase of extracellular potassium and pH on the other hand increases extracellular matrix synthesis. Dynamic loading generates action potential at tissues. Flow in ions under dynamic loads leads to electric charges. Such charges influence chondrocyte metabolism. Chondrocytes are sensitive to shape and function optimal as long as they can preserve their oval phenotypes. This finding is an indirect proof of the presence of mechanoreceptors in these cells. Mechanoreceptors, which may be of protein, can be located on the membrane or cytoplasm of chondrocytes. The function of these mechanoreceptors should be the conversion of mechanical information into biomechanical function.
The upper most layer of joint cartilage decreases friction and allows lubrication. The friction coefficient of this layer of joint cartilage is between 0.02 and 0.04 [31]. Lubrication is attained by four main mechanisms. These are (a) boundary, (b) hydrostatic, (c) hydrodynamic, and (d) elastohydrodynamic mechanisms. All mechanisms are active at the same time during joint cartilage lubrication. Atomic force microscopic assessment [32] revealed sensitivity of 800 nm to 2 mm thick lamina splendens toward protease-containing enzymes.
Role of Synovial Fluid, Proteoglycans, and Collagen at Solution Exchange
Fluid flow along the extracellular matrix is essential for joint cartilage function. Regulatory factors, cytokines, and nutrients reach cells by this flow. Regulatory factors function in a dose and time-dependent manner. They are synthesized in micro- or even nano-quantities, act in less than minutes, and disappear after conducting their function. Concentration of the fluid directly defines extracellular molecule transfer. Ions of the cartilage matrix are primary determinants of osmotic pressure. This pressure preserves fluid of joint cartilage under mechanical loads.
From the mechanical point of view, it is assumed that the intra- and extra-fibrillar areas form two different compartments [33]. Intrafibrillar fluid flow is slower than extra-fibrillar fluid flow. Smaller molecules are transferred in the intrafibrillar area. The extra-fibrillar area holds more fluid due to its proteoglycan content. Loss of proteoglycans in pathologic conditions decreases fluid preservation, and adaptation to loads is adversely affected. Collagen is highly selective to the calcium ion. In an experimental study [33], the calcium content of intrafibrillar calcium concentration increased up to 7.1 in proteoglycan depleted bovine cartilage. Concentrations of sodium, chloride, and bicarbonate change with pressure. For example, extra-fibrillar fluid content increases from 0.2 to 0.6 mEq/g with the increase in pressure. Likewise, sodium and calcium percentage increases from 1.7 to 3.2 and 6.4 to 23.9, respectively, whereas chloride and bicarbonate percentage and pH decrease from 0.7 to 0.5, 0.5 to 0.4, and 7.2 to 6.8, respectively. The fibers of the interfibrillar area cover or leave spaces in between each other (Fig. 22.6). The gap between fibers is less than 0.6 nm. The gap area is about 20 % of all the surface area of joint cartilage. Serum albumin and polyethylene glycol of 6.000–20.00 Da molecular weight contain the intrafibrillar space. Fluid content of joint cartilage decreases when pressure is applied. It is assumed that large channels open under long-lasting static loads in order to retake the lost fluid. The diffusion constant between joint cartilage and synovial fluid is 0.45 that changes due to anionic, cationic, and neutral conditions. Dissolution of ions compared to free fluid is slower. Diffusion space in the cartilage is narrow. Molecules of the synovial fluid are almost the same size as of the gaps of joint cartilage. Interaction between these groups is constant. All these factors are causes of slow synovial fluid and ionic exchange in joint cartilage. Smaller molecule decrease of diffusion constant is geometrically presented [33]. Cyclic loading slows down transportation of small molecules but improves speed of flow of larger molecules such as growth factors, hormones, and enzymes. Synovial fluid flow is important for joint cartilage nutrition. Decrease of motion leads to accumulation of molecules such as lactate and carbon dioxide and may increase tissue degradation.
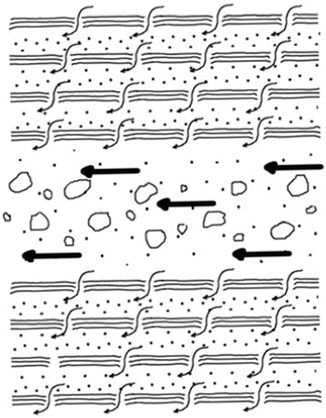
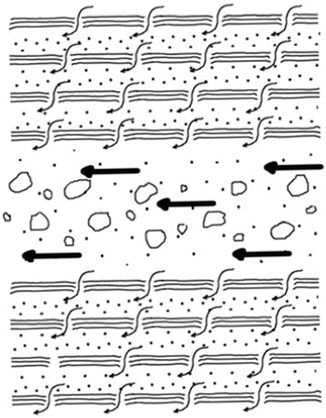
Fig. 22.6
Interfibrillar synovial fluid flow
Geometry of the glycosaminoglycan matrix affects anisotropic hydrolytic permeability of joint cartilage [34]. Intense fibrillar glycosaminoglycan structure is a resistance barrier toward fluid flow. If the glycosaminoglycan matrix had been irregular, then hydrolytic permeability would have been isotropic. Under mechanical loads however, hydrolytic permeability is anisotropic, and geometry is assumed to play an important role in synovial fluid flow [34]. Glycosaminoglycan fiber geometry and direction determine synovial fluid flow and tissue deformation. Important mechanical properties of joint cartilage such as hardness and lubrication capacity are determined by this geometry [35].
Proteoglycan and glycosaminoglycan structures of joint cartilage present biphasic indentation [36]. The Poisson ratio of joint cartilage is between 0.13 and 0.45. Previously, this ratio was measured in laboratory studies as 0.40 and 0.49. This ratio defines how joint cartilage can function under frictional forces throughout decades. Patellar notch joint cartilage thickness permeability is several times higher than that of other locations [36].
Effects of Static and Dynamic Loading on Matrix Metabolism
Collagen fibers resist against tensional forces, whereas the tough and intense structure of proteoglycans allows them to electrostatically bind to glycosaminoglycans and increase strength toward compression [37]. Continuous production and degradation of proteoglycans, collagen, and other matrix molecules preserves mechanical properties of joint cartilage. Density and structure are controlled by molecular biological and cellular harmony under physical conditions.
Loads on the joint and motion may exacerbate complex metabolic activity. Proteoglycan synthesis increases to some extend with increased dynamic load. Static or impact loads however injure joint cartilage. Rupture of the anterior cruciate ligament deteriorates matrix metabolism and may cause osteoarthritis [38]. Physiological loads may lead to structural adaptation when skeletal muscles of the knee joint are trained. Structural adaptation due to mechanical stimulation is difficult to monitor in vivo. Indirect methods include measuring joint cartilage metabolites and growth factors of the serum or synovial joint.
< div class='tao-gold-member'>
Only gold members can continue reading. Log In or Register a > to continue
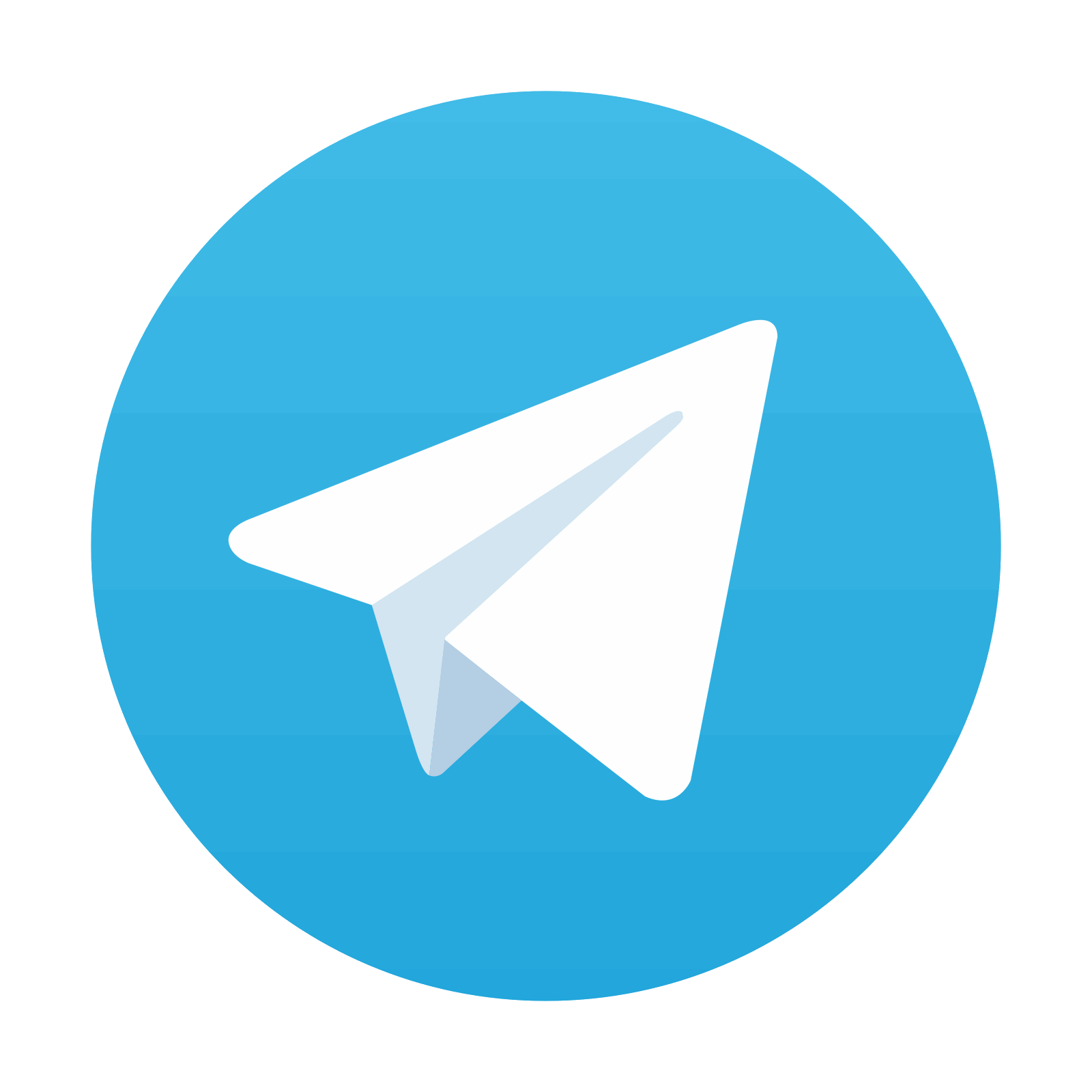
Stay updated, free articles. Join our Telegram channel

Full access? Get Clinical Tree
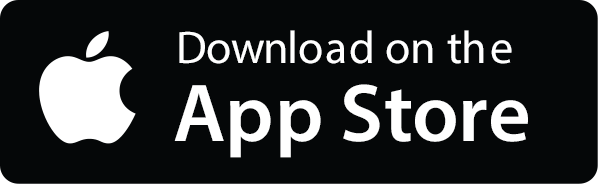
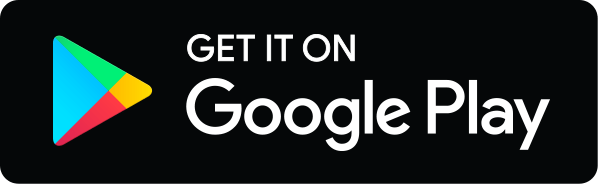