15 INFECTION AND INFECTION CONTROL
Rapid evacuation and transport to trauma centers and sophisticated medical technologies facilitate successful resuscitation and life support after trauma, thereby saving more lives of critically injured patients. Survivors of traumatic events experience compromise of innate host defense mechanisms, increasing their risk for infection.1 It is a medical paradox that the invasive therapies needed to resuscitate and sustain critical trauma patients increase the risk of life-threatening infections, a predominant complication of severely traumatized patients.
Trauma patients have a predisposition to infection, owing to disruption of major protective barriers. In a recent study of 1277 trauma patients, 45.4% had nosocomial infections.2 Of the nosocomial infections, 22% were respiratory infections (including pneumonia, sinusitis, and tracheobronchitis), 22% were intraabdominal/gastrointestinal infections, 21% were genitourinary infections, 20% were bacteremias, and 10% were wound/skin infections.2 Nosocomial infections are a significant complication in trauma patients and are associated with prolonged length of stay, higher health care costs, and increased mortality rates.3 These infections can also lead to the development of severe sepsis and septic shock.2,4 In 2004, The Joint Commission recognized that nosocomial infections are a frequent safety issue for hospitalized patients and issued new patient safety goals aimed at reducing the risk of nosocomial infections.5,6 Early diagnosis and treatment of infection are essential to improve outcome and decrease length of stay and mortality rates of trauma patients.
HOST DEFENSE MECHANISMS
EXTERNAL DEFENSE MECHANISMS
Skin
The major mechanical barrier to invading pathogens is the skin. The skin’s protective ability is enhanced by its natural acidic pH, which inhibits growth of some organisms. Also, sebum, the oily secretion of the sebaceous glands, contains long-chain fatty acids that act as a germicide. Antimicrobial peptides, which are small-molecular-weight proteins secreted by epithelial cells, are toxic to some bacteria, fungi, and viruses.7 Normal skin flora include coagulase-positive and coagulase-negative staphylococci, streptococci, and diptheroids, which act as a potential reservoir of invading organisms.
Elderly patients have the added burden of the age-related changes that affect the skin’s normal function.8 Their skin has less oil secretion, blood flow, innervation, and subcutaneous fat,9 which increases the incidence of skin breakdown and wound infection.10 Similarly, obese patients are also at higher risk for skin breakdown because of impaired mobility and decreased tissue perfusion.
Respiratory Tract
The respiratory tract has a complex system of host defense mechanisms. Mucociliated epithelium cleans and protects airway passages to prevent microbial overgrowth. Coughing, sneezing, and deep breathing provide mechanical clearing of the airways. Lung surfactant proteins appear to promote phagocytosis.11
Pathogens can gain access to lower airways during intubation, by inhalation, or from bloodstream infections, aspiration of gastric contents, or spread from nearby areas.12 The major loss of defenses in trauma patients occurs with placement of an artificial airway. With this intevention, all natural defense mechanisms are circumvented, and microorganisms from the environment can be afforded unobstructed entry into the lungs. Contamination of airway circuits can allow rapid replication of pathogens that are aerosolized into the lungs. Endotracheal tubes allow pooling of oropharyngeal secretions, which contain a variety of pathogens that can pass the inflated cuff and move into distal airways.12
Elderly patients are further compromised by age-related physiologic changes. A gradual decline in many immune functions, including cell-mediated and humoral aspects of acquired immunity, contributes to an increased risk of pneumonia.13 Decreased alveolar elasticity, reduced intercostal muscle and diaphragm tone, and diminished vital capacity hinder the elderly patient’s ability to cough and expectorate sputum, increasing the risk of respiratory tract infection.14
Nurses can promote respiratory tract defense mechanisms by frequently repositioning unconscious and immobile patients and, in awake patients, encouraging the use of incentive spirometry, coughing, and deep breathing to facilitate removal of pulmonary secretions. Chest physiotherapy is vital to critically ill patients, especially those with new pulmonary infiltrates, fever, leukocytosis, or purulent sputum. In a study of critically ill ventilated patients, Joshi et al15 demonstrated the benefits of vigorous chest percussion. Within 8 hours after chest physical therapy, 80% of patients had partial or complete resolution of pulmonary infiltrates. Another important maneuver that increases coughing and deep breathing to promote aveoli opening and secretion removal is getting patients moved out of bed to a chair and assisting with ambulation as soon as their condition permits. Specific interventions to prevent pneumonia are described later in the chapter.
Gastrointestinal Tract
Heavily colonized by a variety of bacteria, the gastrointestinal tract is a major reservoir of microorganisms that can cause nosocomial infection. An intact mucosal epithelium, the acidic gastric barrier, and continuous peristaltic evacuation throughout the tract constitute the external defenses in the gastrointestinal tract. Lytic enzymes in saliva, secretory antibody immunoglobulin A (IgA), lysozyme, and phagocytic cells work to destroy ingested bacteria. Normally the stomach, duodenum, and jejunum are colonized with low concentrations of mouth flora. However, the trauma patient soon loses this protection because normal gut flora is disrupted by injury, ischemia, decreased gut motility, pH changes, and fasting. Decreased visceral perfusion, seen for example in patients in shock, decreases gut barrier function and raises the potential for “translocation” of bacteria out of the gastrointestinal tract.16
Drug therapy may have a negative impact on gastrointestinal tract defense mechanisms. Antacids and H2-blocking agents used to prevent or treat mucosal bleeding elevate the normal gastric pH, eliminating the acidic barrier. Gastric pH greater than 4 facilitates bacterial overgrowth.17 Muscle relaxants, sedatives, and analgesic agents reduce gastric motility. Last, antibiotics change the composition of the normal gut flora.18
INTERNAL DEFENSE MECHANISMS
This aspect of the immune system defends against infectious microbes that breach external defenses and helps eliminate foreign substances. This innate resistance is designed to limit tissue injury and prevent infection by environmental organisms.11 The human body is designed with various physical, mechanical, and biochemical barriers that protect it from environmental substances and microbial pathogens. When the external barriers are compromised, the body’s inflammatory response is activated by internal defense mechanisms (the immune system) to provide protection, prevent infection, and promote healing.11
Injury to vascularized tissue prompts the initiation of a characteristic inflammatory response with clinical manifestations that include redness, heat, swelling, and pain. Redness and warmth result from the increased blood flow to the affected area. Local blood vessels in the microcirculation dilate, and vascular permeability increases, allowing fluid to leak from the vessels. Leukocytes and plasma enter the tissues as the result of increased vascular permeability brought on by chemical reactions of histamine, bradykinin, leukotrienes, substance P, and prostaglandins.19 White blood cells (WBCs) migrate from inside the blood vessels to the site of injury. These effects are visible within seconds.
The inflammatory response initiated to prevent infection includes activation of three plasma protein systems: complement, clotting, and kinin systems.11 The complement system has a dual role in directly destroying pathogens and activating other inflammatory response systems. The clotting system uses a group of plasma proteins to form a fibrinous meshwork at the site of injury or inflammation. This meshwork prevents spread of infection by containing the microorganisms and foreign bodies. It also controls bleeding and creates a framework for tissue repair. The kinin system activates inflammatory cells and works in conjunction with the coagulation system to trap pathogens. Bradykinins, along with prostaglandins, induce pain, produce smooth muscle contraction, increase vascular permeability, and enhance leukocyte chemotaxis.11
Activation of these proteins recruits granulocytes and specialized cells, including neutrophils, eosinophils, basophils, and mast cells to the site of inflammation or infection. Mast cells are found in large numbers in skin and lining the gastrointestinal and respiratory tracts. They are activated and begin synthesizing inflammatory mediators (e.g., histamine, bradykinin, leukotrienes, substance P, and prostaglandins), which increase vascular permeability, activate platelets, and enhance adhesion of leukocytes to endothelial cells. These cells provide an early response to microbes even before infection. The leukocyte response is initiated within 6 to 12 hours after injury, when polymorphonucleocytes (neutrophils) infiltrate the area.11 Their role is to ingest or phagocytize bacteria, dead cells, and cellular debris. The number of circulating neutrophils increases, usually accompanied by an increase in the ratio of immature cells (bands, metamyelocytes, myelocytes) to mature neutrophils-known as the “left shift.” Neutrophils, also called polymorphonuclear cells (PMNs), are granulocyte cells that serve as the predominant phagocytes of early inflammation.11 They are incapable of division and sensitive to acidic environments. As inflammation persists, capillary congestion at the site leads to tissue anoxia, anerobic metabolism, and decreased pH of the exudate, which is lethal to neutrophils. They have a short life span and degrade to become a component of purulent exudates (pus), which is composed of dead and dying leukocytes, blood, plasma, fibrin, cellular debris, and living and dead microorganisms.11 It is not uncommon to have a very high WBC count (20,000-40,000 cells/mm3) immediately after severe traumatic injury because of the inflammatory response.
Other WBC lines that are induced by “protein” messengers are monocytes and macrophages. They are similar to neutrophils in characteristics and functions, but they have a longer life span. Produced in the bone marrow as monocytes, they circulate in the bloodstream, becoming macrophages when they infiltrate the tissues.20 Macrophages and lymphocytes may arrive at the site within 24 hours of injury but are often slow to respond, arriving 3 to 7 days later.11 They can survive in acidic environments and therefore provide long-term defense against infectious agents. They have bactericidal activity against most microorganisms. Macrophages also stimulate other inflammatory factors and initiate the healing process.11
Fever/Thermoregulation as Part of the Immune Sytem
Fever (defined as core temperature >100.4° F [38° C])21 results from any disturbance in the hypothalamic thermoregulatory activity that leads to an increase of the thermal set point. Fever can be an infectious or a noninfectious inflammatory response. The infectious fever response is initiated when exogenous pyrogens, such as lipopolysaccharide complexes in the cell wall of gram-negative bacteria, invade the body. Endogenous pyrogens, fever-causing cytokines, are released by neutrophils and macrophages in response to exposure to bacterial endotoxins or to antigen-antibody complexes. Endogenous pyrogens include interleukin-1 (IL-1), interleukin-6 (IL-6), tumor necrosis factor (TNF), and interferon. These pyrogens act on the preoptic nucleus of the hypothalamus to change the body’s thermostat.22 The hypothalamus and the brainstem signal increased heat production and conservation to raise the body temperature. Peripheral vascular vasoconstriction causes blood to shunt to the body’s core, thereby creating a new temperature level.22
A febrile response may have beneficial effects by killing temperature-sensitive microbes. Fever also causes decreased levels of iron, zinc, and copper, which are needed for bacterial replication. In addition, elevated body temperature changes metabolism to lipolysis and proteolysis, thereby depriving bacteria of glucose sources. Fever can also increase neutrophil mobility, which increases their killing capability by enhancing their phagocytic capability.22 Because of the beneficial effects of fever, antipyrogenic medications should be used judiciously. Antipyretics can negate the protective fever response and may increase the mortality rate.21
Fever may also be harmful by increasing the effects of endotoxins from gram-negative bacteria.11 Also, pyrexia exacerbates ischemic neuronal damage and physiologic dysfunction after acute brain injury.23 To avoid increasing the cerebral metabolic rate, which can increase the risk for intracranial hypertension and brain ischemia, hyperthermia is typically aggressively treated in patients with acute severe brain injury. Hypermetabolism caused by fever may not be well tolerated by trauma patients in need of nutrients for healing.
Acquired Immunity
When a microbe bypasses external defenses and the initial inflammatory cascade system is primed, additional mechanisms are triggered. By this adaptive or acquired immunity, the human body is able to develop powerful specific immunity against invading bacteria, viruses, and toxins. This is often thought of as the humoral or antibody response. The innate and adaptive immune systems are linked. Stimulation of the innate immune system, as described previously, initiates the adaptive immune response, which is slower acting, specific, and very long lived.24 When challenged by substances identified by the body as “foreign,” the adaptive immune system responds with lymphocytes, the WBCs that make antibodies. Foreign antigens include microbial pathogens (bacteria, fungus, virus, or parasites), noninfectious environmental agents (pollen, food, venom), and clinical mediators (drugs, vaccines, transfusions, transplants). Repeated exposure causes the adaptive immunity to “remember” the invader and increase the number of lymphocyte-produced specific antibodies.24 When activated, lymphocytes migrate through lymphoid tissues and become either B lymphocytes, responsible for humoral immunity, or T lymphocytes, responsible for cell-mediated immunity. Although B lymphocytes make antibodies, T lymphocytes are involved in complex cell-mediated responses that provide resistance to infection.25 The human body produces millions of T and B lymphocytes, each capable of recognizing one specific foreign antigen. B cells secrete specialized antibodies that attack antigens. For example, antibodies bind to extracellular microbes and toxins, facilitating elimination of bacteria and viruses. T cells undergo differentiation with maturation and develop into subgroups. Some stimulate leukocytes, whereas others become cytotoxic cells that directly attack and kill antigens. This immunity provides defense against intracellular viruses and bacteria that can live within phagocytes inaccessible to circulating antibodies, thereby eliminating reservoirs of infection.
The body’s response to the threat of injury or infection, though usually protective, can be inadequate or excessive and uncontrolled. Understanding how tissue damage can be contained, how infection can be prevented and detected, and how best to treat injuries and promote healing enables nurses to provide optimal patient care.11
INFECTION
In an attempt to categorize and study the protean manifestations of the body’s response to invasion, various definitions are used. Experts from a number of North American and European intensive care societies have agreed on consistent terminology and definitions for the response to insults that may trigger inflammation, namely, infection.26 Infection is defined as the pathologic process that occurs when microorganisms invade a normally sterile body fluid, tissue, or cavitiy.26 The definitions of systemic inflammatory response syndrome (SIRS), sepsis, severe sepsis, septic shock, and multiple organ dysfunction syndrome (MODS) are presented later in this chapter.
INFLAMMATORY RESPONSE
Inflammation is the normal response of living tissue to injury or infection.27 This complex process mediated by the internal immune system is described earlier in this chapter (see Internal Defense Mechanisms). Inflammation is a nonspecific response27 to tissue damage that can be caused by direct trauma or induced by a variety of mechanical, chemical, and biologic stimuli. In addition to tissue trauma, inflammation can be induced by the presence of foreign objects such as bullets, stones, sutures, catheters, and orthopedic hardware; exposure to toxic agents such as extremes of temperature, ionizing radiation, and chemicals; and the presence of biologic agents such as microorganisms, bacterial toxins, antigen-antibody complexes, and devitalized and necrotic tissue. The more extensive the tissue destruction, the greater the inflammatory response.28 When the inflammatory response results from tissue necrosis, the progression of systemic inflammation is rapid and blood cultures often have negative results.
Systemic Inflammatory Response Syndrome
SIRS is a complex overall inflammatory response affecting multiple organs with or without infection. It can be precipitated by insults such as trauma, infection, pancreatitis, or surgery. It is seen in almost 70% of patients in critical care and is also common to trauma patients.2 SIRS is a massive response of intrinsic mediators and proinflammatory cytokines indicated by the presence of at least two of the following clinical criteria: fever (temperature ≥38° C) or hypothermia (temperature ≤36° C), tachycardia (≥90 beats/min), tachypnea (≥20 breaths/min) or partial pressure of carbon dioxide in arterial blood (PaCO2) <32 mm Hg, leukocytosis (≥12,000 cells/μL) or leukopenia (≤4000 cells/μL), or a left shift in the immaturation of granulocytes (bands) >10%.29
A SIRS diagnosis does not confirm presence of infection or sepsis but is associated with adverse outcomes.2 In trauma patients, the SIRS score on admission is an independent predictor of nosocomial infection and mortality. A SIRS score >2 continues to be predictive of nosocomial infection through 21 days after injury.2 SIRS scores have poor specificity, and studies are being done to explore a combination of other biomarkers (e.g., CRP, procalcitonin [PCT]) that can provide a reliable early indicator of infection.
Sepsis
In the United States, sepsis accounts for more than 210,000 deaths each year. It is the leading cause of death among critically ill patients.30 Sepsis has been denoted as a malignant intravascular inflammation because it is uncontrolled, unregulated, and self-sustaining. Extension of the normal local inflammatory response to infection into normal tissue is termed sepsis, an autodestructive process.31 Sepsis is a complex, systemic inflammatory response to infection, with or without positive blood cultures. In addition to infection, at least two of the SIRS criteria must be present to meet the definition of sepsis.26 Sepsis is a proinflammatory response and, because it activates the coagulation system, thus altering the procoagulation-anticoagulation balance, it is also a procoagulant response. The activated coagulation system depletes plasmin and antithrombin III and activates protein C, leading to hemorrhage when anticoagulation factors are depleted. Activation of the coagulation cascade and the resultant coagulopathy produce diffuse microthrombi. As microthrombi obstruct flow through capillaries, the tissues become unable to to receive and extract oxygen. Arterial vascular tone decreases and endothelial permeability increases, causing a redistribution of intravascular fluid volume, which fosters progression to severe sepsis. Acute respiratory alkalosis, resulting from stimulation of ventilation, may be seen in early sepsis; however, as deterioration progresses to septic shock, lactic acidosis develops.32
Severe sepsis is defined as sepsis with organ dysfunction, hypotension, or hypoperfusion.26,33 It is a leading cause of death in noncoronary intensive care units (ICUs).34 In the United States, patients with severe sepsis have a mortality rate of 28% to 50%.35 Annually, 200,000 patients in the United States die of severe sepsis.36 With an average individual cost of care of $22,000, health care for sepsis accounted for expeditures of $16.7 billion in the United States in 2000.34 It is anticipated that these figures will rise as the population ages, the number of immunocompromised patients increases, more antibiotic-resistant organisms emerge, the use of invasive procedures and monitoring devices expands, and the awareness of severe sepsis criteria increases.21
Organ dysfunction in severe sepsis results from hypotension and tissue hypoperfusion. Hypotension is defined as mean arterial blood pressure (MABP) <60 mm Hg, systolic blood pressure (SBP) <90 mm Hg, or a decrease in SBP of >40 mm Hg from baseline without other causes.26 Hypoperfusion leads to tissue hypoxia and ischemia. Clinical signs of tissue hypoperfusion may include oliguria, lactic acidosis, or acute alterations in mental status.26
Septic Shock.
Septic shock is sepsis accompanied by hypotension and tissue hypoperfusion, despite adequate fluid resuscitation, with other systemic abnormalities, which may include lactic acidosis, oliguria, and acute mental status changes.26,29 Infection from a variety of bacteria, fungi, and, rarely, viruses can cause septic shock. These pathogens or exotoxins produced by bacteria interact with the host immune cells to incite a massive inflammatory response. Noncytokine and cytokine mediators (e.g., IL-1, TNF-α, IL-6) are released, activating systemic inflammation and release of mediators (e.g., thromboxane A2, prostaglandins, and nitric oxide), which causes vasodilation and endothelial damage. Cytokines also activate the coagulation pathway, creating capillary microthrombi.37 The inflammatory and procoagulant responses initiated by bacterial toxins result in capillary leakage, inadequate circulatory volume, circulatory collapse, and potentially profound hypotension despite adequate fluid resuscitation. This leads to impaired tissue perfusion and ischemia. As perfusion of nonvital tissues, and eventually vital organs, decreases and the body shifts to anaerobic cellular metabolism, lactate production increases, leading to metabolic acidosis. To compensate for hypoxia and acidosis, respirations become rapid and shallow. Uncorrected, this respiratory insufficiency can lead to acute respiratory failure. If the shock state is not corrected quickly, the patient’s survival is jeopardized. The mortality rate from septic shock is 50% to 60%.34
As sepsis progresses, organ system function becomes compromised. MODS occurs when organ function is altered and homeostasis cannot be maintained without intervention. The first systems affected are usually the cardiovascular and pulmonary systems, followed by hepatic, gastrointestinal, and renal systems, and, finally, the brain.21 As one or more organ systems fail, the mortality rate increases; with failure of three or more systems, the mortality rate reaches 70%.38 Organ system failure is closely linked to a hypercoagulable state.
Assessment for Sepsis/Septic Shock.
Vital signs, particulary changing trends in these measures, should be noted.32 Increasing heart and respiratory rates and elevated or reduced body temperature could meet the criteria for SIRS. In early septic shock, the increase in cardiac output offsets low systemic vascular resistance; therefore, systolic blood pressure is maintained. Over time, as circulating blood volume is further depleted and compensatory mechanisms are exhausted, blood pressure will decline. Assessment of WBC count (either leukocytosis or leukopenia) and differential is also important, although leukocytosis is neither sensitive nor specific for infection. As a result of inadequate tissue perfusion, organ dysfunction affecting one or more organs can become apparent. It is important to monitor for signs of altered tissue perfusion, including altered mentation, systemic hypotension, decreased urinary output, prolonged capillary refill time, hypoxemia, respiratory compromise requiring ventilation, and respiratory alkalosis progressing to metabolic acidosis.26 Earliest signs can include increased apprehension, confusion, and decreased sensorium (in a sedated or brain-injured patient, these findings may not be evident).26,39 Signs of coagulation imbalance, such as decreased platelet count, abnormal coagulation studies, increased bleeding at venipuncture sites, and petechiae, may also indicate sepsis.26,39 If two or more of the SIRS criteria are met, infection is deemed present or suspected, the patient is hypotensive or has evidence of tissue hypoperfusion, and one or more organs is dysfunctional, severe sepsis is suggested.34
Investigations continue in attempting to identify a biologic marker that would provide early, accurate detection of sepsis with good specificity and sensitivity. As mentioned earlier, the host response in sepsis is modulated by a cascade of proinflammatory cytokines or mediators (e.g., TNF, IL-1, IL-6, and interleukin-8 [IL-8]) followed by immunomodulators that down-regulate the response (e.g., anti-inflammatory mediators include interleukin-4 and interleukin-10). Markers of interest include IL-6 and IL-8, which may correlate with patient outcome. High IL-6 levels are associated with death in septic patients. However, the cytokines are not specific and are also induced by various noninfective stimuli, including surgery, viral infections, and autoimmune disorders.40 There is great clinical interest in using these markers for predicting patients most at risk for sepsis.
Currently available markers include PCT, CRP, and the erythrocyte sedimentation rate (ESR). PCT is a propeptide produced in the thyroid gland. Usually at low systemic levels (<0.1 ng/ml), the PCT concentration rises dramatically in sepsis to more than several hundred nanograms per milliliter. PCT levels seem to reflect increasing severity of the systemic inflammatory response.2 Another early marker is CRP, a liver-produced protein released after inflammation or tissue damage. It has both proinflammatory and anti-inflammatory actions and is frequently used to detect the presence and severity of infection or sepsis. However, multiple-trauma patients and elective surgery patients may show an increase of PCT, CRP, and other biomolecules, indicating inflammation during the early postoperative or posttraumatic period independent of the diagnosis of sepsis or infection.41–43
Recent investigations have identified a new potential sepsis biomarker, sTREM-1. This soluble triggering receptor expressed on myeloid cells has a sensitivity of 96% and a specificity of 89% in determining the presence of infection.44 Other biomarkers being studied for their potential prognostic ability include endotoxin (a component of gram-negative cell walls), brain natriuretic peptide (a myocardial dysfunction indicator), and endogenous protein C.45 Much research is under way to determine the accuracy of these biomarkers in identifying and guiding the treatment of sepsis.
Management of Sepsis and Septic Shock.
In 2003, critical care and infectious disease experts convened at an international conference to develop evidenced-based guidelines for the management of sepsis and to launch an initiative to encourage use of these interventions to improve patient outcomes from sepsis—the Surviving Sepsis Campaign. Key recommendations focus on the need for early syndrome recognition. New monitoring and therapy developments place great importance on the early identification and aggressive management of the septic patient. Meta-analysis of sepsis resuscitation trials showed that early interventions before organ dysfunction provide better outcomes.45 Rivers et al46 showed that the timeliness of treatment significantly improves the mortality rate when hemodynamic optimization is achieved within the first few hours after disease presentation. Patients with septic shock have a significantly greater risk of death when antibiotics are started more than 1 hour after initial presentation or diagnosis of septic shock.47 These and other recommendations for managing sepsis are included in the established guidelines. The guidelines continue to evolve, and the reader is referred to the latest published recommendations for up-to-date suggestions for the management of sepsis.
The key recommendations of the evidence-based guidelines for management of sepsis and septic shock are highlighted in Box 15-1. Many instituations have developed treatment algorithms on the basis of these evidence-based recommendations. An example of an algorithm is found in Figure 15-1.
BOX 15-1 Guideline Recommendations for Management of Sepsis and Septic Shock
< div class='tao-gold-member'>
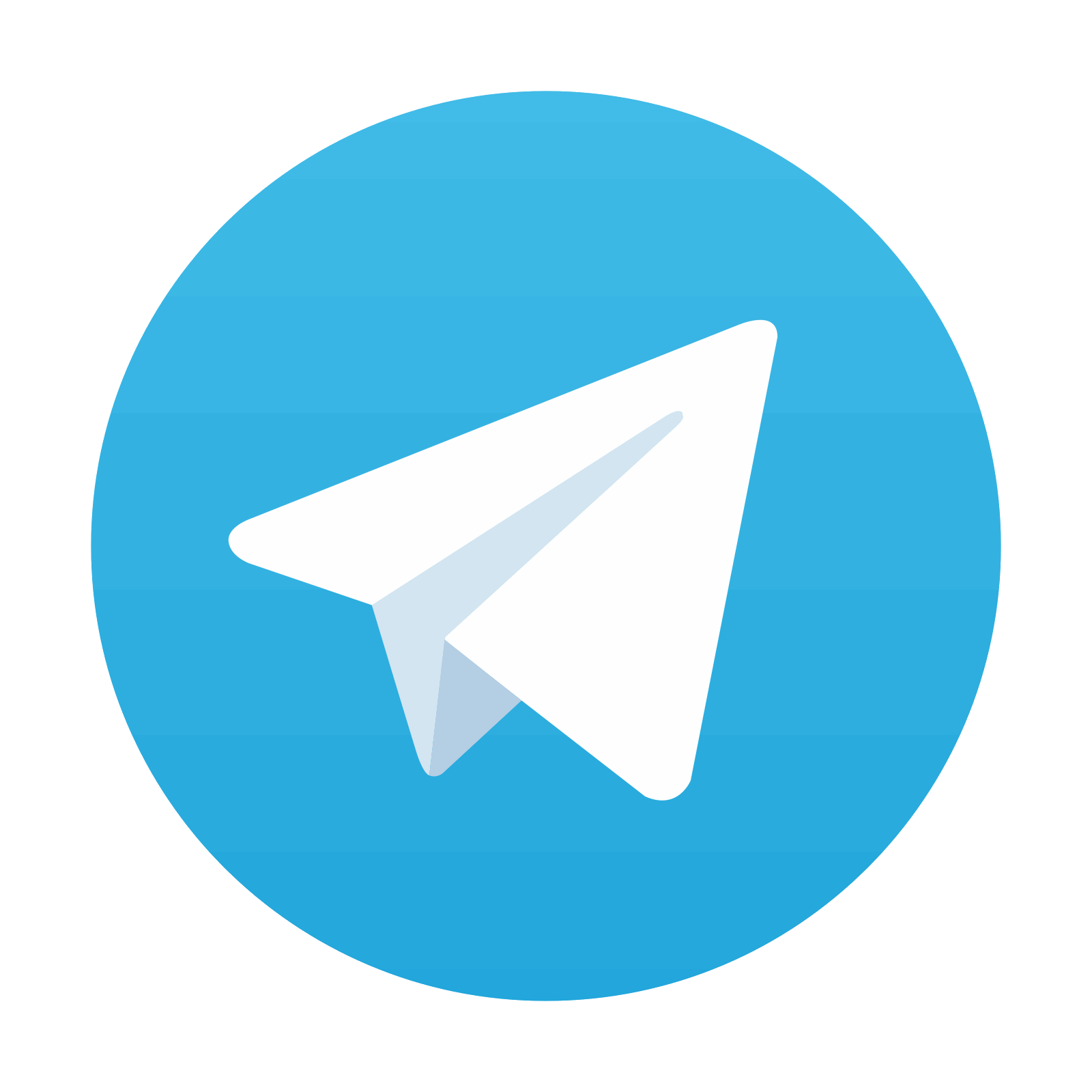
Stay updated, free articles. Join our Telegram channel

Full access? Get Clinical Tree
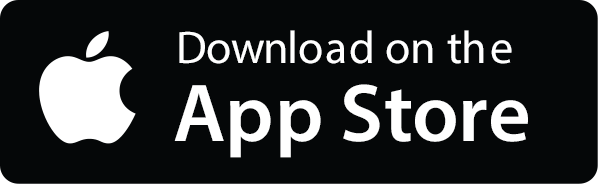
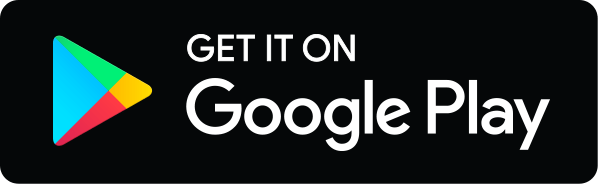
