Infection
Hany Bedair and Craig J. Della Valle
Key Points
Introduction
Despite the great success of total hip arthroplasty, deep infection remains one of the most devastating complications. This diagnosis almost inevitably leads to prolonged and complex treatments involving reoperation and ultimately poorer outcomes. The cost to the patient is enormous with regard to the impact on the physical and mental health state. Treatments usually are costly and place significant burden on any healthcare system. In a review of the Healthcare Cost and Utilization Project Nationwide Inpatient Sample, infection was the third most common reason (after instability and loosening) for revision surgery following total hip arthroplasty (THA) and was by far the most common reason for removal of the prosthesis.1 Although prevention, diagnosis, and treatment have improved as surgical technique and patient management have evolved, the risk of developing this feared complication has not been eliminated. The unfortunate state is that as the number of total hip arthroplasties expected to be performed in the coming years significantly increases, so too will the prevalence of deep infection.2
There exist innumerable factors in infinite permutations that may contribute to or directly result in deep infection following THA. To simplify and thus best address this complex clinical scenario, these factors can be organized into three groups: the host, the local wound environment, and the microbiology.3 By investigating and addressing how these entities contribute singularly and interact with one another, a better understanding of the disease process and its treatment will emerge.
Risk Factors and Prevention
The incidence of deep infection following total hip arthroplasty (THA) in the early experience of Charnley was 9% but fortunately was reduced to 1.3% in his next cohort of patients; this was attributed to air cleanliness and body exhaust attire.4,5 Although this dramatic reduction was realized over the course of the initial 10 years of his experience into the late 1960s, currently the acknowledged rate of infection is approximately 1% at large-volume centers.6–10 This relatively small but important improvement in incidence can be attributed to many improvements, including better patient selection, the use of prophylactic antibiotics, and operating room measures.
Improved patient selection has been predicated upon the identification of host risk factors, both systemic and local, predisposing to infection following THA. Although the physiologic stress of surgery11 itself may predispose to infection, multiple factors should be recognized and, if possible, addressed to reduce risk. Malnutrition and advanced age are independent factors known to affect innate immunity and normal immune response by altering both humeral and cell-mediated immunity, thus increasing infection risk.12–15 Systemically immunocompromised patients, because of underlying disease or pharmacologic treatment, are at increased risk for infection. Patients with rheumatoid arthritis and those being treated with steroid therapy are at increased risk for infection.5,13,16,17 Obesity18 increases the odds of infection 4.2 times. Patients with diabetes mellitus17,19-21 appear to experience higher rates of infection compared with nondiabetic patients, with some studies suggesting an astonishingly high rate of nearly 11%22 in matched groups. Improved perioperative glycemic control appears to reduce the risk of infection in thoracic surgery.23 Although definitive prospective studies have not been completed as of yet, suggestions have been put forth that strict glycemic control in the perioperative period may reduce the rate of infection in THA patients.24 Chronic renal failure patients on dialysis25 and those with liver or kidney transplants are at increased risk for infection.26 Patients with malignancy19 and those with human immunodeficiency virus, particularly patients with CD4 counts below 240 cells/mm3,27 are also at increased risk.
Local host factors that appear to increase the risk of infection include previous infection of the native hip that has not been quiescent for longer than 10 years28 and revision hip arthroplasty for any cause of failure.16 Delayed wound healing and persistent wound drainage often lead to infection.15,29
Remote sources of infection, including skin lesions,30,31 dental caries or procedures,32,33 and urinary retention34 with subsequent bladder instrumentation can lead to infection at the site of THA. Systemic predispositions should be optimally minimized and potential local and remote sources of infection should be addressed before the time of surgery.
Administration of an appropriate prophylactic antibiotic is considered the single most important factor in reducing postoperative wound infection.3,7,8,35 The choice of a first-generation cephalosporin is often made on the basis that this agent is bacteriocidal, therapeutic against commonly infecting pathogens, and inexpensive while possessing an appropriate half-life. Antibiotic prophylaxis with cefazolin before and after surgery was demonstrated to reduce the risk of infection after THA compared with placebo from 3.3% to 0.9% in a double-blind controlled study.36 For patients with hypersensitivity to penicillin, clindamycin or vancomycin should be considered as the alternative.3 The preoperative dose of prophylactic antibiotic appears to be most effective in reducing infection when administered within 2 hours preceding the incision,37 and peak bone concentrations are reached within 35 to 40 minutes after intravenous administration.38 A multicenter prospective trial observed that when the preoperative antibiotic was given within 30 minutes before incision, the rate of surgical site incision was 1.6%, compared with 2.4% when the antibiotic was given between 31 and 60 minutes.39 In addition, this trial found that intraoperative re-dosing in cases lasting longer than 4 hours appears to reduce the risk of infection. The postoperative duration of prophylactic treatment is debatable; a prospective double-blind multicenter trial found no difference in treatment for 24 hours versus 3 days.40 Multiple other studies have confirmed that prolonged prophylactic treatment does not appear to be of benefit.41,42
Modifications of the surgical suite environment, including limiting operating room personnel or “traffic”, surgical time, and the use of iodophor-incorporated drapes, have been shown to reduce the risk of infection.43–47 Use of ultraclean air operating rooms, vertical laminar flow, and body exhaust suits has been demonstrated to favorably affect the rate of infection; however, these points have been debated.5,48-51 Gortex gowns may be more appropriate than cotton in preventing dissemination of shed bacteria by the surgical team.52 The use of double gloves and the choice of the optimal antiseptic remain controversial.3 Frequent changing of the suction tip may also decrease the introduction of bacteria into the wound.53 Pulsatile lavage and antibiotic irrigation solutions are effective in reducing the wound bacterial load.54–56
Although rates of bacteremia following oral procedures and diagnostic procedures of the genitourinary and gastrointestinal tracts have been documented,57 and even though bacteremia may lead to bacterial introduction to the hip arthroplasty,58 antibiotic prophylaxis given before these procedures remains controversial.3 The most recent statement by the American Academy of Orthopaedic Surgery recommends “antibiotic prophylaxis for all total joint replacement patients prior to any invasive procedure that may cause bacteremia,” which includes all dental procedures. The previous treatment limitation period of 2 years postoperatively has been removed, and the current recommendation is for prophylactic treatment of all patients, independent of the time elapsed since surgery. The recommended regimen is 2 g of cephalexin, cephradine, or amoxicillin 1 hour before the dental procedure. Those allergic to penicillin should receive 600 mg of clindamycin 1 hour before the procedure.
Microbiology
A thorough understanding of the microbiological environment that leads to infection after total hip arthroplasty is crucial to successful prevention and treatment. The introduction of a biomaterial into the body has been likened to a race to inhabit the surface of the material between host tissue and bacterial pathogens.59 Should the bacteria win this race, the result is often deep infection of the prosthesis. Introduction of bacteria to the prosthetic interface may occur at the time of surgery through direct inoculation, or it may occur at a later time through hematogenous seeding. Certain bacteria may have a predilection for seeding different biomaterials. For example, Staphylococcus aureus seems to preferentially seed metallic implants, and Staphylococcus epidermidis shows a preference toward polymers such as polyethylene and polymethylmethacrylate. Many bacteria form a mucopolysaccharide biofilm that isolates and protects organisms from the host immune response, antibiotic penetration, and even mechanical débridement. Even though approximately 75% of cultures in a single study revealed a single organism,60 retrieval and microbiological analysis have demonstrated that the bacterial glycocalyx may harbor multiple different bacterial species.61 This makes treatment of such patients difficult without removal of the prosthesis because the appropriate antibiotic regimen cannot be determined. Recent investigation into methods of better diagnosis through molecular diagnostic techniques, as well as more effective antibiotic biofilm penetration, has demonstrated that rifampin appears to improve treatment protocols when used synergistically with other antibiotic agents.62,63
In multiple large studies, the most common offending organisms are gram-positive aerobes ranging between 64% and 74% of all infecting organisms following total hip arthroplasty, with the Staphylococcus species (mainly S. epidermidis and S. aureus) representing approximately half of these gram-positive cocci.7,60,64,65 Other identified gram-positive organisms include Enterococcus, Streptococcus viridans, and Streptococcus groups A, B, and G. There may exist a trend toward an increasing prevalence of cultures positive for S. epidermidis and a decrease in gram-negative bacteria.66 Gram-negative organisms known to cause periprosthetic hip infection range from 11% to 14% of all isolated organisms and include Pseudomonas aeruginosa, Enterobacter cloacae, Serratia marcescens, Proteus mirabilis, Escherichia coli, Klebsiella pneumoniae, Acinetobacter species, Moraxella nonliquefaciens, and Salmonella cholerasuis. Other more rare organisms that have been reported include anaerobes, mycobacterium, and fungi.
A trend that has generated extreme concern has been the emergence of antibiotic-resistant bacterial strains. Over a 5-year period, nosocomial infection rates in intensive care unit (ICU) patients had increased by 31% for methicillin-resistant S. aureus and by 55% for vancomycin-resistant Enterococcus.67 Fortunately, these alarming trends have not been observed to this same magnitude in periprosthetic infection of the hip, but they do exist. The rate of methicillin-resistant S. epidermidis in hip replacement patients was observed to be as high as 40% of 30 positive cultures68 and 48% of 56 S. epidermidis cultures.60 In a single study of 35 infected cases following THA, 54% were infected with a resistant organism, and results of treatment for these resistant organisms were far inferior to that given for sensitive organisms, with successful retention of the prosthesis in only 16%.69 In a second study comparing the treatment of methicillin-resistant and methicillin-sensitive S. aureus joint infections, patients with methicillin-resistant organisms had significantly longer hospital stays and higher risks of treatment failure and removal of the prosthesis.70 This further emphasizes the critical need for accurate diagnosis and identification of the infecting species and its sensitivities in providing optimal care for these patients.
Diagnosis
The diagnosis of infection following total hip arthroplasty requires a high level of suspicion and clinical acumen. These findings should be supplemented with serologic testing, radiographic evaluation (conventional and occasionally advanced imaging), and synovial fluid analysis. Diagnosis should be confirmed by cultures and/or histopathology. The diagnosis should be established expeditiously because the treatment algorithm may shift depending on the chronicity of the infection.
A thorough history should identify any risk factors for infection, including immunocompromised states, history of prior hip surgery, or history of infection. Persistent wound drainage postoperatively or superficial wound infection raises concern for persistent deep infection. Any abnormalities in the recovery period, including persistent postoperative or even new-onset deep pain, are causes of concern for infection. A history of fever can indicate infection; however, this finding can be unreliable. Any recent procedures that may have caused bacteremia or remote infection should be investigated.
The range of motion of the hip should be determined and compared with previous examination findings. Guarding, pain with passive motion, pain at rest, and diminishing motion are matters of concern regarding infection. A thorough neurovascular examination, as well as examination of the skin for any remote lesions, should be performed. The wound should be carefully examined for drainage, erythema, fluctuance, or an active or healed sinus tract. Wound drainage should not be cultured because these results are highly inconsistent and may only confuse the clinical picture. Empirical antibiotics for wound drainage should be avoided because they only serve to suppress and delay the definitive diagnosis.
Plain radiographs should be evaluated and compared with prior studies for the presence of rapid or progressive loosening, osteolysis, periosteal or endosteal reaction, and osteopenia. These findings, however, may have other causes. Implant loosening or osteolysis within the first 2 years is particularly suspicious for infection (Fig. 100-1). Plain radiographs can be helpful should any of these findings be observed, but the lack of radiographic changes does not exclude infection.
Figure 100-1 Plain radiograph of a total hip arthroplasty (THA) with a cementless acetabular component and a cemented femoral component demonstrating significant osteolysis and implant loosening of the femoral component at 21 months postoperatively, which is highly suggestive of deep infection.
C-reactive protein (CRP) level and erythrocyte sedimentation rate (ESR) should be the initial screening laboratory tests when infection is suspected. In a recent study of preoperative testing for more than 200 revision THAs, 100% specificity for a hip not to be infected was noted when both CRP and ESR were normal.65 An elevated ESR (>30 mm/hr) was shown to have a sensitivity of 97%, a specificity of 39%, a positive predictive value of 42%, and a negative predictive value of 96%. A CRP value greater than 10 mg/L was shown to have a sensitivity of 94%, a specificity of 71%, a positive predictive value of 59%, and a negative predictive value of 96%. These values are consistent with the findings of previous studies, which additionally showed that when both the ESR and CRP are elevated, the probability of infection was 83%.71
If the ESR and CRP are elevated, or if the clinical suspicion for infection is high based on the history and physical examination aspiraiton of the hip is recommended and the fluid obtained is sent for a synovial fluid white blood cell count, differential and culture.65,72-74 Patients should be off of antibiotics for a minimum of two weeks prior to aspiration to maximize culture yield and accuracy.71,73 Table 100-1 provides recommended diagnostic criteria from several previously reported studies of periprosthetic infection of the hip and knee using ESR, CRP, synovial WBC, and differential. Although these synovial tests alone may provide valuable information, the combination of these test results with ESR and CRP results can greatly improve diagnostic accuracy.65 Bacterial growth on solid media and gross purulence visualized within the joint are generally considered diagnostic for infection,75 although the clinician should be aware that cultures (even on solid media) can be falsely positive, and alternative causes may be proposed for purulent appearing fluid around a prosthetic hip. These include wear of a polyethylene or metal-on-metal bearing surface; thus the results of multiple tests should be analyzed together to allow the most accurate conclusion to be drawn.
Table 100-1
Previously Reported Values for Diagnosis of Periprosthetic Joint Infection
†Units given in these cases are as reported in source literature.
Because serologic markers of inflammation (ESR, CRP), synovial WBC count, and differential are normally elevated in the early postoperative period, these tests may be of debatable utility in the first few weeks after surgery. Recent work by Bedair and associates76 has revealed in a multicenter, multisurgeon study of almost 12,000 primary total knee arthroplasties that these markers of inflammation can be used reliably in the diagnosis of periprosthetic infection of the knee in the early postoperative period (<6 weeks from surgery), but at threshold levels higher than those previously reported. Specifically, the ESR was not found to be useful in the early postoperative period, but the CRP was useful, with an optimal cutoff value of 95 mg/L. The synovial fluid WBC count was nearly a perfect test at diagnosing infection, with a threshold of less than 10,000 WBCs/µL being an excellent “rule-out test,” and a threshold of approximately 28,000 WBCs/µL being an excellent “rule-in” test. The differential was also helpful, with an optimal balance of sensitivity and sensitivity at greater than 90% PMNs. Unpublished data from our institution demonstrate similar utility of these tests in the diagnosis of periprosthetic hip infection in the early postoperative period (Table 100-2).
Table 100-2
Diagnostic Values for Early Postoperative Periprosthetic Joint Infection
CRP, C-reactive protein; ESR, erythrocyte sedimentation rate; PMNs, polymorphonuclear neutrophils; WBC, white blood cell.
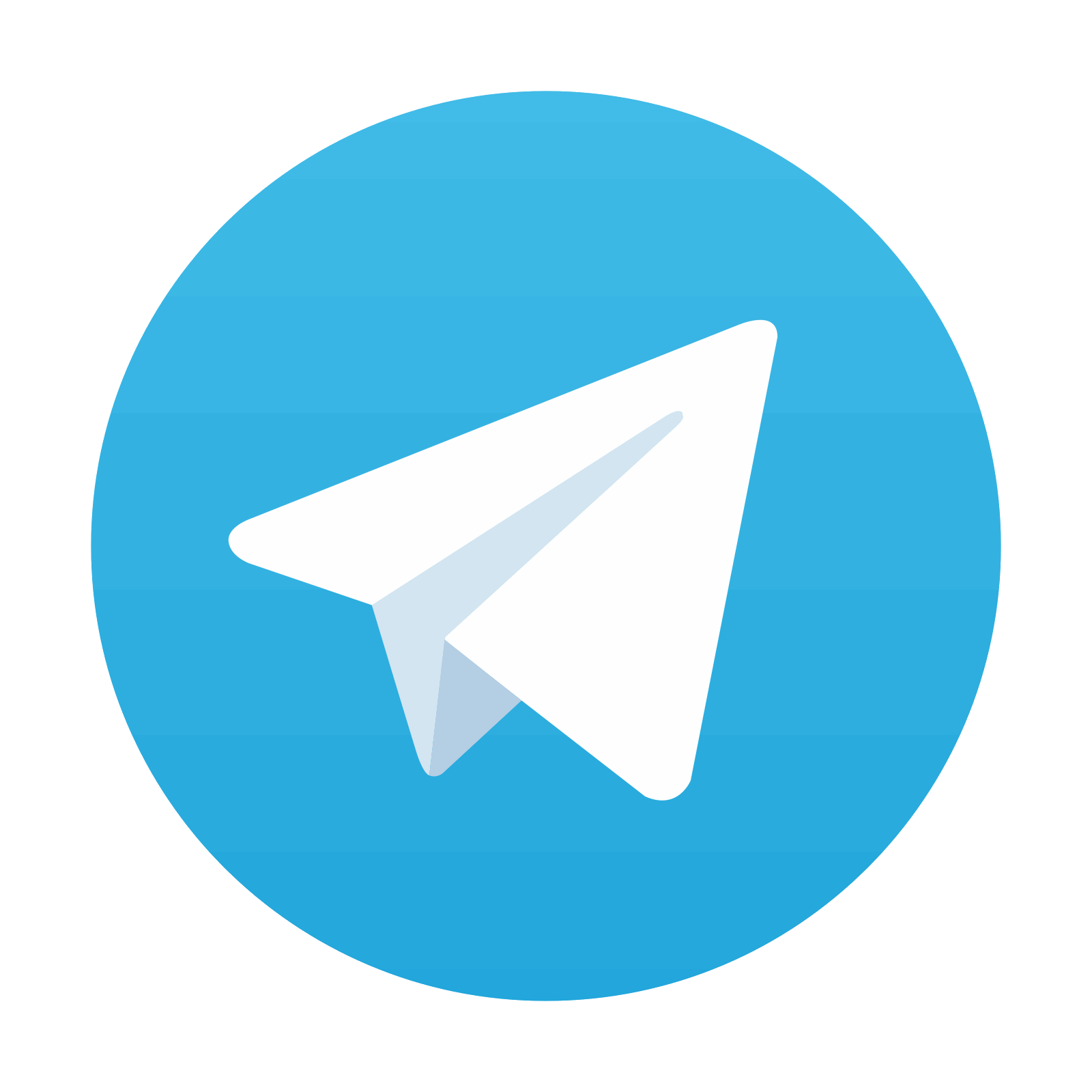
Stay updated, free articles. Join our Telegram channel

Full access? Get Clinical Tree
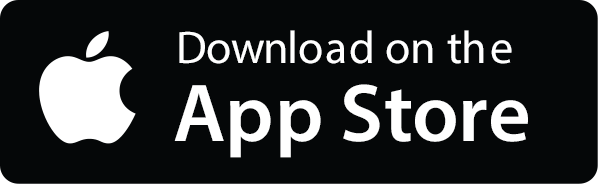
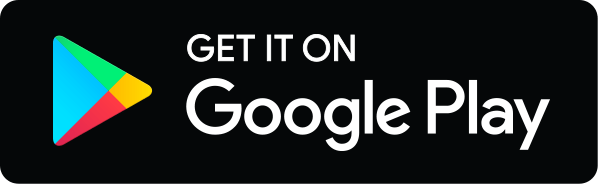