Chapter 16 In Vivo Mechanics and Vibration of the Knee Joint
Knee joint injuries are one of the most commonly reported musculoskeletal problems. These injuries can occur because of various reasons. In young adults, sports are a major cause of injuries. In older subjects, arthritic degeneration (e.g., rheumatoid arthritis or osteoarthritis) of the knee is a well-known phenomenon and is known to result from a variety of traumatic causes. This leads many patients to undergo total knee arthroplasty (TKA) to correct such conditions. At least 150 TKA designs are available in the market today, with advancements by physicians and engineers that simulate the geometry and behavior of a healthy knee joint.7 The differences in these designs are based on factors such as condylar geometry, bearing mobility, and ligament preservation versus substitution and fixation methods.32
Experimental studies in humans are difficult and often restrictive because of the exclusion of any measuring device that would require invasive techniques. Because cadaveric studies fail to simulate in vivo conditions adequately,22 biomechanical researchers have strived for new and unique methods for indirect measurements. These have been used to analyze the normal knee joint and assess and justify design protocols of TKAs.
Knee Kinematics: Mobile or Fixed, Retaining or Sacrificing?
Determining the kinematics of the knee (normal and implanted) has been investigated using multiple techniques, both in vitro and in vivo. These include invasive cortical pins, roentgen stereophotogrammetric analysis (RSA), skin marker analysis,2 and fluoroscopy-based model reconstruction techniques.
In vivo fluoroscopic studies have shown that the kinematics of the normal knee is determined by the condylar geometry of the articular surfaces. The results of those studies have revealed that the lateral condyle experiences significantly more posterior motion than the medial condyle throughout knee flexion8 (screw-home mechanism).
Component design engineers and surgeons continue to collaborate in an effort to develop TKA systems that reproduce normal knee kinematic patterns. However, fluoroscopic studies of multiple implant designs have shown much less predictable and reproducible knee kinematics after TKA. Numerous kinematic variances from normal knee kinematic patterns have been demonstrated, including paradoxical anterior femoral translation during deep knee flexion, reverse axial rotational patterns, and femoral condylar liftoff.12
Anterior femoral translation during deep knee flexion—femoral component sliding anteriorly during deep flexion rather than posterior femoral rollback—has numerous negative consequences. Anterior femoral translation results in a more anterior axis of flexion, lessening maximum knee flexion. The quadriceps moment arm is decreased, resulting in reduced quadriceps efficiency. Anterior sliding of the femoral component on the tibial polyethylene surface risks accelerated polyethylene wear. Some TKAs have shown the tendency to produce undesirable reverse axial rotation, which risks patellofemoral instability caused by lateralization of the tibial tubercle and an associated increase in the Q angle during deep flexion, as well as lessening maximum knee flexion because of reduced posterior femoral rollback of the lateral femoral condyle.11
In spite of these limitations, TKAs in general have provided good midterm to long-term survivability. However, the question on which type of implant configuration provides the best postsurgical outcome is still a cause for debate. One area of contention is bearing mobility. The mobile-bearing implant was designed to reduce contact stress, thereby reducing wear, and re-create more normal-like knee kinematics. In vitro studies have shown reduced wear with the use of a mobile-bearing implant.16,17 However, kinematics, clinical outcomes, and survivability between fixed- and mobile-bearing implants have produced similar results. A review study conducted by Post and colleagues29 could not find any basis to justify one design over the other. Clinical success and long-term survivorship were found to be mainly dependent on the accuracy with which the components were implanted. It was concluded that the best design is the one with which the surgeon is most comfortable and most able to implant reproducibly. Studies that have compared the performance of the mobile and fixed configurations in the same patient have also concluded that the patient does not demonstrate any difference in terms of range of motion, knee scores, and survivorship.3,21,30,32 Pagnano and associates27 conducted a study on 240 rotating platform TKAs and found that it did not improve patellar tracking. A multicenter study conducted by Wasielewski and coworkers39 on 527 mobile-bearing TKAs found that only 12% of the knees exhibit more than 10 degrees of axial rotation during a deep knee bend (DKB) activity. Also, almost half the knees analyzed experienced less than 3 degrees of axial rotation. They also found that the rotational parameters are comparable with results reported for fixed-bearing TKAs by Dennis and colleagues.11
Another area of contention is whether the posterior cruciate ligament should be retained or substituted. Numerous studies have shown that certain cruciate-retaining (CR) total knee designs exhibit paradoxical femoral sliding, decreasing clinical weight-bearing flexion.8 This paradoxical femoral rollback (PFR) was not seen in posterior-stabilized (PS) TKAs. However, a study comparing PS TKAs with CR TKAs with asymmetrical condyles found that although the CR design did exhibit lesser medial PFR, both designs achieved similar amounts of lateral PFR. Proponents of the CR design have suggested that the PFR seen in PS TKAs is caused by the guided motion provided by the cam-post engaging, which leads to higher rates of implant failure because of cam-post wear. In their discussion of failure of the cam-post mechanism, Bourne and Baré6 found that all cam-post mechanisms are not the same and that substantial differences exist from one implant type to another. They also suggested that the cam-post mechanism does not always engage as designed. However, they did find evidence that the cam-post mechanism may result in increased wear; this was limited to implants with varus-valgus constraints.
We have carried out a study to estimate cam-post engagement during a DKB activity for 10 knees with a fixed-bearing bicruciate stabilizing design and 9 knees with a high-flexion mobile-bearing PS design. In vivo, weight-bearing knee kinematics was determined for the participants while they performed a DKB activity under fluoroscopic surveillance. The three-dimensional kinematics was re-created from the fluoroscopic images using a previously published three-dimensional to two-dimensional registration technique.24 To determine the polyethylene insert location and orientation for the mobile-bearing design, four metal beads were embedded into the polyethylene insert prior to the replacement surgery. Images from full extension to maximum flexion were analyzed at 10-degree intervals. Once the three-dimensional kinematics of all implant components was re-created, the analysis focused on the cam-post mechanism. The distance between the interacting surfaces was monitored throughout the flexion and the predicted contact map was calculated. When the minimum distance between the cam and post surfaces dropped to zero, this was considered to indicate the engagement of the mechanism.
From this analysis, it was found that in the bicruciate-stabilized design (BCS), the cam-post engagement varied among patients, with the average flexion at which engagement occurred being 34.0 degrees (Fig. 16-1). However, there were subjects who had engagement as early as 15.0 to 20.0 degrees and also subjects who had engagement as late as 68 degrees. Most subjects had initial contact with the tibial post medially, with the contact location moving centrally and superiorly with increasing knee flexion. For the mobile-bearing PS design the cam-post interaction was found to be very consistent among subjects (Fig. 16-2), and the stabilizing mechanism engaged in deeper flexion (between 90 and 105 degrees). It was also observed that once the two components engaged, they remained in contact until maximum flexion was achieved (Fig. 16-3). The interesting finding was that unlike the fixed-bearing BCS design, the contact between the cam and post for the mobile-bearing PS design was located centrally on the post at all times when engaged. This is probably because of the mobility of the polyethylene, which is characteristic for the analyzed TKA design. The polyethylene insert rotated axially in accord with the rotating femur. Therefore, the posterior surface of the mobile-bearing post was able to remain parallel to the surface of the femoral cam. As a result, the stresses imposed on the polyethylene insert could be distributed more evenly and over a larger area, which could increase the longevity of this component. Such conditions may conceivably be difficult to achieve for a fixed-bearing TKA, in which the femoral component rotating externally may cause the cam-post mechanism to engage more medially, increasing the edge loading on the polyethylene, as was seen with the BCS design. The results from this study are with accordance with the findings of Bourne and Baré 6 cited earlier, and also suggests that mobile-bearing PS designs may have an advantage when compared with their fixed-bearing counterparts in terms of long-term survivability. Because the mobile insert ensures that the cam and post are parallel to each other, contact between them occurs centrally, hence reducing the chances of asymmetrical loading conditions, as seen in the fixed-bearing design.
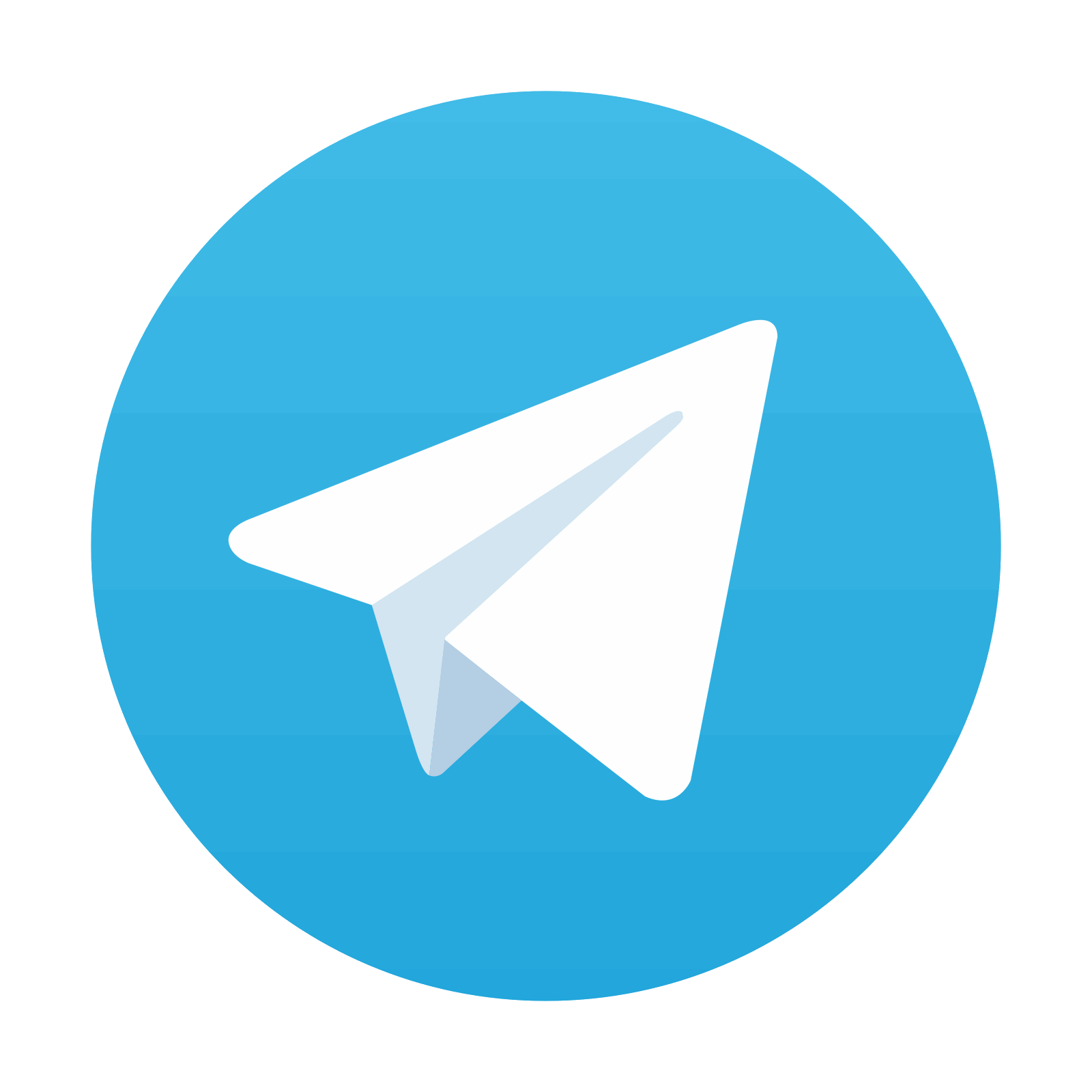
Stay updated, free articles. Join our Telegram channel

Full access? Get Clinical Tree
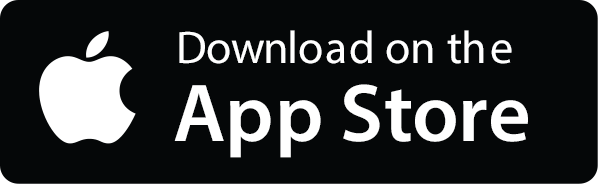
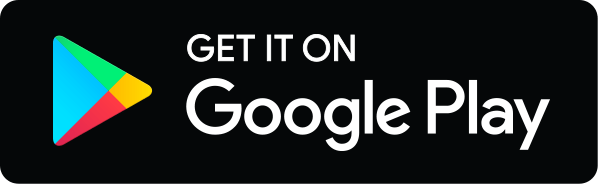