(3)
Labrum is University of Pittsburgh Medical School, Arner is Orthopedic Residency UPMC, University of Pittsburgh Medical Center, 3200 South Water Street, Pittsburgh, PA 15203, USA
Take-Home Message
The normal acetabulum is anteverted and caudally oriented.
The iliofemoral, pubofemoral, and ischiofemoral ligaments surround the hip joint and place limitations on hip movement.
The ascending cervical branches of the medial and lateral femoral circumflex arteries are the primary blood supply of the femoral head and neck.
Acetabular and labral blood supply is derived mainly from the superior gluteal, inferior gluteal, and obturator arteries.
Introduction
Prototypical ball-and-socket joint provides a wide range of motion that is surpassed only by the shoulder. The femoral head and the acetabulum comprise the osseous elements of the hip.
The femoral head closely approximates a sphere and is entirely covered by hyaline cartilage, except for a central depression termed the fovea capitis, which serves as the femoral attachment site for the ligamentum teres.
The acetabulum is often described as horseshoe shaped and is the result of fusion between the ischium, ilium, and pubis bones. The anterior wall of the acetabulum is thin and provides only minimal support, whereas the acetabular roof and posterior wall are composed of much denser bone. The acetabulum is deficient inferiorly, giving rise to the acetabular notch—which is bridged by the transverse ligament.
Due to the variation in wall thickness, the orientation of the acetabulum does not lie in standard anatomical planes; rather, the normal acetabulum faces forward with slight anteversion and also faces downward with caudal orientation.
Ligaments
Three strong ligaments span the hip joint and integrate with surrounding connective tissue to help form the hip capsule.
Iliofemoral ligament (Y ligament of Bigelow)—Located anteriorly and prevents hyperextension at the hip
Pubofemoral ligament—Blends with the lower fibers of the iliofemoral ligament and limits excessive abduction
Ischiofemoral ligament—Lies posteriorly and also resists hyperextension
Taken together, the restrictions placed on the hip joint by these three ligaments result in a capsule that is taught with extension and external rotation and relaxed with flexion and internal rotation.
Acetabular Labrum
Several important intra-articular structures are present within the hip capsule, including the labrum and ligamentum teres.
The labrum is a fibrocartilaginous structure attached to the edges of the acetabulum. Inferiorly, the labrum blends with the transverse ligament at the margins of the acetabular notch.
The function of the labrum continues to be investigated, although it is commonly held that it serves to
Stabilize the hip by deepening the socket
Act as a seal to maintain joint fluid within the intra-articular space
Contain the femoral head during acetabular formation
Evidence suggests that the labrum does not participate in direct load transmission.
Ligamentum Teres Femoris
Originates in the fovea capitis.
Courses along the inferior aspect of the hip joint where it attaches distally and medially to the transverse ligament and to several surrounding structures, including the central nonarticular acetabular surface.
Forms the pulvinar, in conjunction with the small fat pad within the acetabular fossa.
Long felt to be of no real importance in the adult hip; however, there is increasing evidence that it plays a role in hip stability.
Blood Supply of the Femoral Head and Neck
Branches of the medial and lateral femoral circumflex arteries form the primary blood supply to the femoral head and neck. These arteries may originate as branches from either the profunda femoris or femoral arteries.
The medial femoral circumflex artery courses posteriorly between the iliopsoas and pectineus muscles before giving rise to medial, posterior, and lateral ascending cervical branches.
The lateral femoral circumflex artery runs anterior to the iliopsoas and gives rise to an anterior ascending cervical branch.
Together, these four ascending branches form the extracapsular arterial ring; of the four, the lateral branch is responsible for providing most of the blood supply to the femoral head.
The ascending cervical arteries proceed to traverse the capsule along its attachment at the base of the femoral neck. Within the capsule, they form a subsynovial anastomotic ring on the surface of the femoral neck at the margin of articular cartilage. This is known as the subsynovial intra-articular arterial ring.
A second and much smaller source of blood to the femoral head is the artery of the ligamentum teres or foveal artery. This artery arises from the acetabular branch of the obturator artery, which itself is a branch of the external iliac artery. However, this artery is said to be often occluded in adults.
Acetabular Blood Supply
Acetabular blood supply comes primarily from branches of the superior gluteal, inferior gluteal, and obturator arteries.
Superior gluteal artery—Deep branches supply the superior rim of the acetabulum
Inferior gluteal artery—Reaches the gluteal region by coursing through the lower part of the greater sciatic foramen. Once outside the pelvis, its branches travel inferiorly to supply the posteroinferior regions of the acetabulum
Obturator artery—Several branches contribute to acetabular blood supply. Of these, the acetabular branch is most significant, which emerges from the acetabular notch beneath the transverse ligament and supplies the acetabular fossa
Labral Blood Supply
Blood supply of the acetabular labrum has received increased attention as diagnosis and treatment of labral pathology continues to evolve.
As with the acetabular osseous tissue, the labrum derives its blood supply from branches of the superior gluteal, inferior gluteal, and obturator arteries.
Labral vascularity differs by region and can be divided into four quadrants, as demonstrated in Fig. 1.
Fig. 1
Four quadrants of labral vascularity. Zones IA and IB receive a peripheral vascular contribution largely derived from the capsule, whereas zones IIA and IIB are relatively avascular (Adapted from Kelly et al. Vascularity of the hip labrum: a cadaveric investigation. Arthroscopy. 2005;21:3–11)
Zones IA and IB receive a peripheral vascular contribution largely derived from the capsule, whereas zones IIA and IIB are relatively avascular.
Thus, the majority of the vessels present within the labrum come from the capsular periphery and show minimal penetration into the central articular-sided region.
Bibliography
1.
Bardakos NV, Villar RN. The ligamentum teres of the adult hip. J Bone Joint Surg Br. 2009;91:8–15.
2.
Blankenbaker DG, De Smet AA, Keene JS, Del Rio AM. Imaging appearance of the normal and partially torn ligamentum teres on hip MR arthrography. AJR Am J Roentgenol. 2012;199(5):1093–8.
3.
Chung SM. The arterial supply of the developing proximal end of the human femur. J Bone Joint Surg Am. 1976;58:961–70.
4.
Ferguson SJ, Bryant JT, Ganz R, Ito K. The acetabular labrum seal: a poroelastic finite element model. Clin Biomech. 2000;15:463–8.
5.
Itokazu M, Takahashi K, Matsunaga T, Hayakawa D, Emura S, Isono H, Shoumura S. A study of the arterial supply of the human acetabulum using a corrosion casting method. Clin Anat. 1997;10(2):77–81.
6.
Kelly BT, Shapiro GS, Digiovanni CW, Buly RL, Potter HG, Hannafin JA. Vascularity of the hip labrum: a cadaveric investigation. Arthroscopy. 2005;21:3–11.
7.
Kim Y-H. Acetabular dysplasia and osteoarthritis developed by an eversion of the acetabular labrum. Clin Orthop. 1987;215:289–93.
8.
Konrath GA, Hamel AJ, Olson SA, et al. The role of the acetabular labrum and the transverse acetabular ligament in load transmission in the hip. J Bone Joint Surg Am. 1998;80:1781.
9.
Rao J, Zhou YX, Villar RN. Injury to the ligamentum teres: mechanism, findings and results of treatment. Clin Sports Med. 2001;20:791–9.
10.
Takechi H, Nagashima H, Ito S. Intra-articular pressure of the hip joint outside and inside the limbus. Nihon Seikeigeka Gakkai Zasshi. 1982;56(6):529–36.
2 Biomechanics of the Hip Joint
Joseph Labrum4
(4)
Labrum is University of Pittsburgh Medical School, Arner is Orthopedic Residency UPMC, University of Pittsburgh Medical Center, 3200 South Water Street, Pittsburgh, PA 15203, USA
Take-Home Message
Analysis of biomechanics using free body diagrams is performed assuming a one-legged stance and static conditions.
The force exerted by the abductor musculature must be approx. 2.5 times greater than that of the gravitational force to keep the pelvis level.
In equilibrium, the joint reaction force is approx. 2.7 times body weight.
Ways of decreasing the joint reaction force include decreasing body weight or altering the lever arms of the abductors or gravitational force.
The joint reaction force reaches three to four times body weight while walking and seven to eight times while running in-line.
Hip Joint Characteristics
Principal link between the axial skeleton and lower extremity, responsible for transferring weight between the two
Central role in generation and transmission of forces
Exceptional amount of inherent osseous stability due to anatomy of the femoroacetabular interface
Motion is almost entirely in rotational plane with no detectable amount of translation; predictable motion allows for simplifications necessary to analyze biomechanics
Simplifications for Analysis
It should be noted that biomechanics of the hip are not influenced solely by local anatomy; as the trunk and lower limb are connected through the hip, remote structures in these areas can have considerable impact on hip biomechanics.
However, it is customary to simplify the analysis by excluding the contribution of remote structures.
Additional assumptions must also be made: although the femoral head is slightly aspherical and, therefore, does not have a single center of rotation throughout hip motion, the analysis presented here will assume a perfectly round femoral head.
Free Body Diagram
Analysis using free body diagrams of the hip joint have traditionally been performed assuming a one-legged stance under static conditions and evaluating forces that occur in the frontal plane.
While this scenario is somewhat artificial, it presents a powerful illustration of the impact that even subtle changes in body position or hip anatomy can have on hip biomechanics.
Given the simplifications above, there are three main forces acting on the hip to consider (Fig. 2).
Fig. 2
Free body diagram of forces acting on the hip during a one-legged stance under static conditions. W gravitational force, A force of abductor musculature, R joint reaction force, a lever arm of gravitational force, b lever arm of abductor musculature
The gravitational force, W—calculated as the weight of the body minus the weight of the ipsilateral lower limb.
This gravitational force acts on a lever arm, a, that is the distance between the body’s center of gravity and the center of the femoral head.
Under conditions of equilibrium, the center of gravity is situated in the midline of the trunk, just anterior to the sacrum.
The force exerted by the abductor musculature, A—acts on a lever arm, b, that extends from the lateral aspect of the greater trochanter to the center of the femoral head.
The joint reaction force, R—the compressive force experienced between the femoral head and the acetabulum.
In order to hold the pelvis level during a one-legged stance, the abductor musculature must exert a moment that is equal to that of the gravitational pull. Likewise, it must exert a moment that is greater than the gravitational pull if the pelvis is to be tilted to the same side while walking.
However, since the length of the lever arms for the two forces differs, forces W and A will not be equal when the pelvis is held level; instead, force A must be approximately 2.5 greater than force W since that is the approximate ratio of lever arm a to lever arm b.
Calculation of the Joint Reaction Force
Under static conditions, the sum of the force vectors W, A, and R must equal to zero in order to keep the pelvis level.
Thus, R can be calculated as the sum of the vectors W and A (Fig. 3).
Fig. 3
Sum of force vectors acting on the hip. In order to maintain the pelvis level, the sum of vectors A and W must equal the magnitude of vector R
In equilibrium, R is calculated to be 2.7 times greater than body weight and is directed 69° from the horizontal during a single leg stance.
The joint reaction force can be determined if the abductor force is known, according to the equation
A is calculable if the weight and lever arms a and b are known. This equation approximates the weight of one lower limb as 1/6 total body weight.
Reduction of Joint Reaction Force
The equation above also illustrates the potential routes of decreasing the joint reaction force:
Decreasing total body weight
Decreasing the lever arm of the gravitational force
Increasing the lever arm of the abductor musculature
Any of these changes allow for a decrease in the force exerted by the abductor musculature and thus decrease the joint reaction force.
The classic Trendelenburg lurch provides an example of this principle. During such a gait, the individual’s weight is shifted over the standing leg, bringing the center of gravity closer to the center of hip rotation and thus decreasing the lever arm of the gravitational force.
The result is a decrease in the amount of force generated by the abductor musculature necessary to stabilize the pelvis and consequent decrease in joint reactive force.
Cane Use
Use of a cane can also help reduce the load experienced across the hip joint.
When used in the contralateral hand, the cane also allows for a reduction in abductor force by creating an upward directed force that helps offset the gravitational pull from the patient’s weight.
The resultant decrease in load across the hip can be substantial, with some researchers reporting up to a 42 % decrease in abductor muscle activity when near-maximal effort with a cane is used.
Correlates to a reduction in hip joint reaction force from 3.4 times body weight to 2.2 times body weight
Motion and Dynamic Loading
Examining the forces about the hip under static conditions, while useful, is not entirely practical.
The effects of motion and dynamic loading conditions are likely more relevant when considering the athlete. Researchers have calculated estimated loads across the hip joint under walking and running conditions.
Between three and four times body weight during walking, at various points of the gait cycle and at different speeds.
Approximately seven to eight times body weight are transmitted across the hip joint during running.
However, calculations were performed for straight-line movement, and do not include potential forces encountered during cutting, pivoting, and other changes of direction.
In vivo measurements have been made possible in recent years by implanting pressure transducers into prosthetic components.
Bibliography
1.
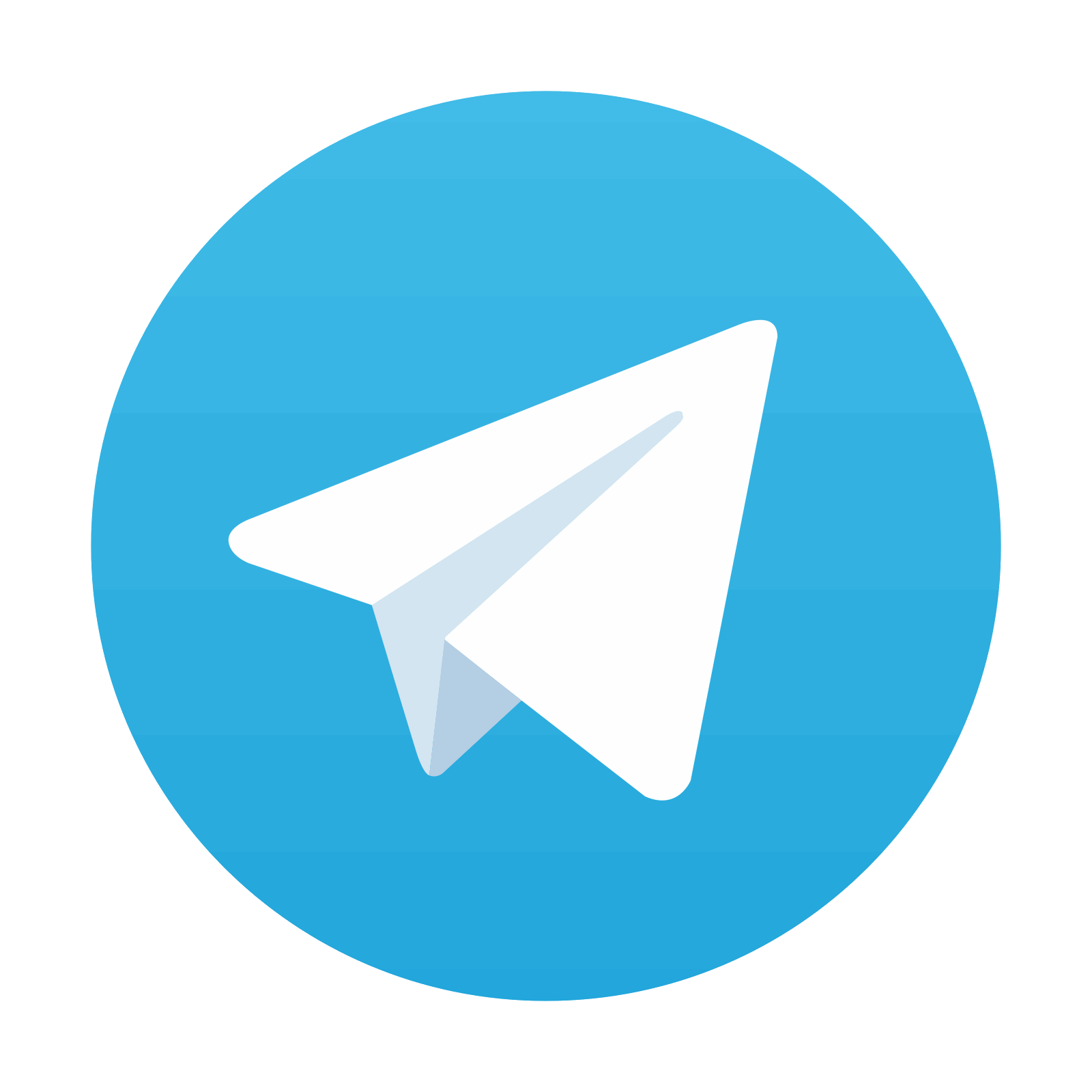
Bergmann G, Deuretzbacher G, Heller M, et al. Hip contact forces and gait patterns from routine activities. J Biomech. 2001;34:859–71.
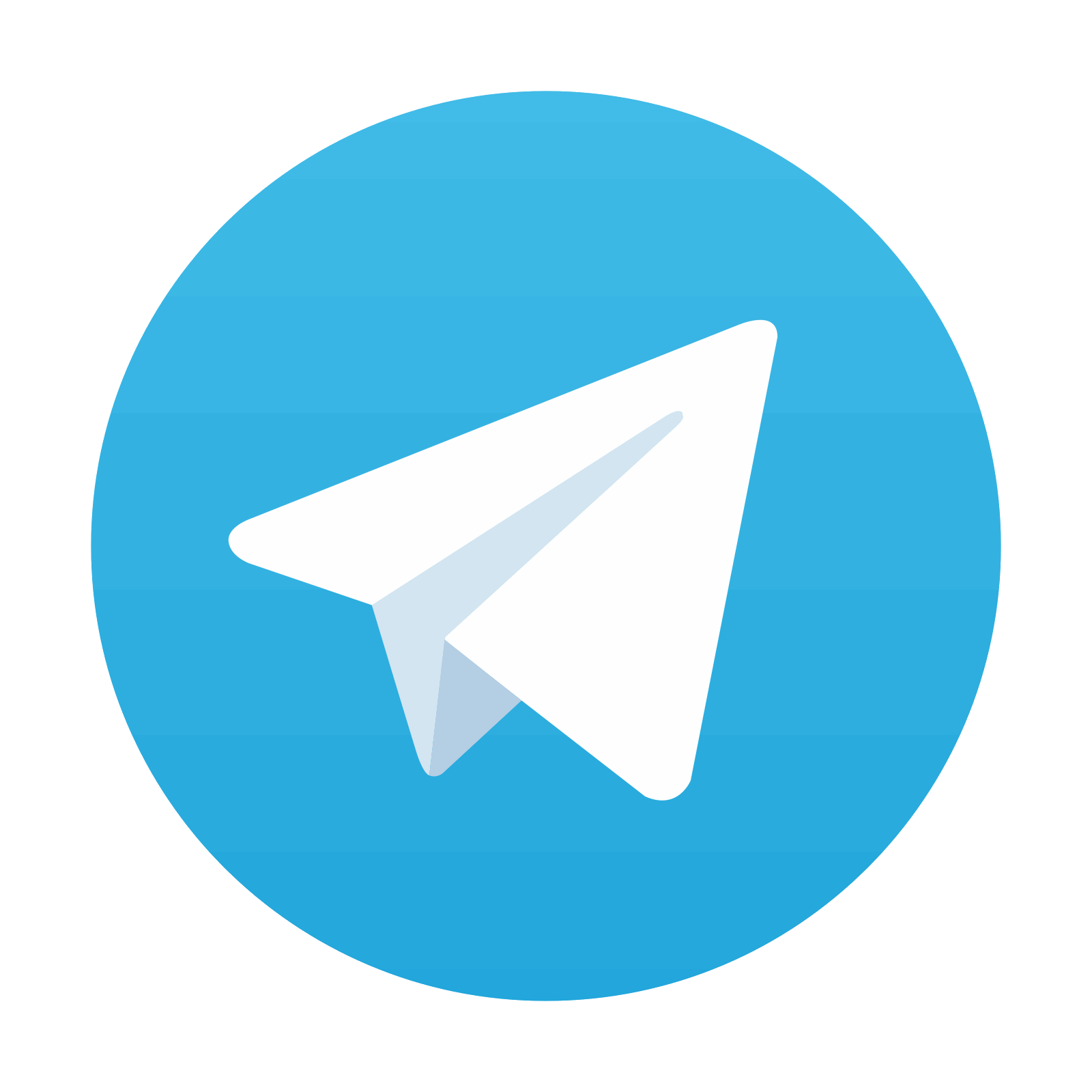
Stay updated, free articles. Join our Telegram channel

Full access? Get Clinical Tree
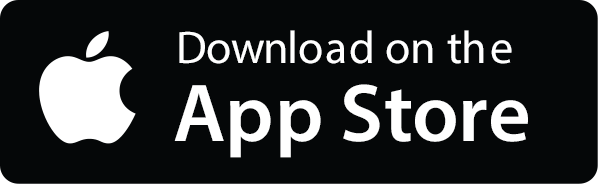
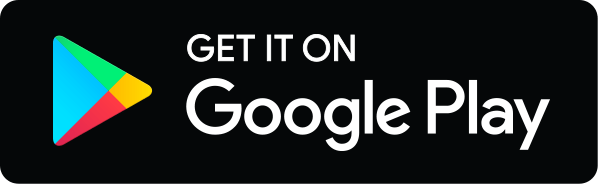
