In the last decade, advances in ultrasound technology, specifically the development of high-frequency linear transducers, have made ultrasound an indispensable tool for imaging the musculoskeletal system. Using ultrasound to identify target structures for botulinum neurotoxin injection is a natural extension of the applications of musculoskeletal ultrasound. The goals of incorporating ultrasound with neurotoxin injections are to improve the accuracy of needle placement, avoid undesired structures, minimize volume overload during injection, and reduce the risk of the procedure. This article provides an update on the neurotoxins and their expanded use in clinical practice, and a detailed review of the use of ultrasound for the varied indications and applications for neurotoxin injection.
In the last decade ultrasound has become an indispensable tool for imaging the musculoskeletal system as well as for guiding various medical procedures. Advances in ultrasound technology, specifically the development of high-frequency linear transducers, have made this possible. High-frequency transducers have revolutionized imaging of musculoskeletal (MSK) structures of interest including muscle, tendons, joints, nerves, and other soft tissues. Using ultrasound to identify target structures for botulinum neurotoxin injection is a natural extension of the applications of MSK ultrasound. The goals of incorporating ultrasound with neurotoxin injections are to improve the accuracy of needle placement, avoid undesired structures, minimize volume overload during injection, and reduce the risk of the procedure. The purpose of this article is to provide an update on the neurotoxins and their expanded use in clinical practice, and a detailed review of the use of ultrasound for the varied indications and applications for neurotoxin injection.
The botulinum toxins: past, present, and future
Toxin Structure
Clostridium botulinum bacteria are gram-positive anaerobic rods that produce a family of 7 serologically distinguishable exotoxins: A, B, C1, D, E, F, and G. Only botulinum toxins (BoNTs) type A (BoNT-A) and type B (BoNT-B) are available for clinical use. All of the BoNTs produce temporary presynaptic blockade of cholinergic nerve terminals by blocking the release of acetylcholine (ACh) at neuromuscular junctions (NMJ) and neuroglandular junctions (NGJ).
The BoNTs are synthesized as a single 150-kDa chain. For bioactivity this single chain must be “nicked” or cleaved into a bichain molecule composed of a 100-kDa heavy chain (HC) and a 50-kDa light chain (LC) linked by a disulfide bond. Only some species of C botulinum contain the proteases required to nick/activate the toxin. Nonproteolytic bacteria such as those producing serotype E require trypsin to activate the toxin. The percentage of toxin B that is recovered nicked from culture is lower than that of BoNT A, which is 95% nicked. This factor is one of several which may account for the observed potency and potential immunogenic differences between and within the serotypes of BoNT.
The bichain BoNTs are folded into a complex 3-dimensional (3-D) globular shape. The shape of the individual toxins influences acceptor binding at the NMJ and proteins involved in ACh release. The size of the total complex of the different commercially available products varies from 500 to 900 kDa because of the presence of accessory molecules, including hemagglutinin (HA) and nonhemagglutinin (NH) proteins, associated with the toxin. The HA and NH proteins act to stabilize and shield the toxin. Individual BoNT products vary significantly in the manufacturing process, the strain of C botulinum toxin used for culture, and the amount of accessory protein. These differences are specified in product information provided by each manufacturer. For internalization and bioactivity, BoNTs must remain in the linked bichain form (HC-LC linked). If the LC-HC bond is cleaved before injection, the LC cannot cross the presynaptic membrane and the toxin is rendered inactive.
Bioactivity and Pharmacology
The intracellular production, transport, and release of ACh vesicles is a complex process involving a suite of SNARE proteins (Soluble N -ethylmaleimide-sensitive factor Attachment Protein Receptors). Normally, when an action potential reaches the NMJ or NGJ it induces synaptic vesicles to dock/fuse with the presynaptic membrane and release their contents of ACh into the synaptic cleft. BoNTs do not affect the synthesis, production, or intracellular packaging or transport of ACh in cholinergic nerve terminals. Rather, the toxins exert their effect by blocking synaptic vesicles from docking or binding at the NMJ, thereby preventing the release of ACh. The process of BoNT acceptor-mediated binding and vesicle blocking is a multistep process and includes:
Step 1: Binding. The C-terminus of the BoNT HC binds avidly to serotype=specific binding sites on presynaptic cholinergic nerve terminals called active zones. The N-terminus of the HC contributes to internalization of the toxin (see later discussion). Evidence suggests this process is dependent on Ca 2+ binding and the involvement of synaptotagmin, one of the SNARE proteins.
Step 2: Internalization of BoNT. BoNT is endocytosed forming an enclosed endosome formed by the lipid bilayer of the cell membrane.
Step 3: Translocation of the LC. For activity the LC must exit the endosome moving into the cytosol to reach its SNARE target. Once endocytosed the HC is cleaved from the LC by a mechanism that has not yet been discovered. The nitrogen or N-terminus of the HC acts as a transmembrane chaperone for the LC by creating a conducting channel or membrane pore in the endosome. The conducting channel created by the HC allows the LC to translocate the lipid bilayer of the endosome and move into the cytosol. Because of its size and 3-D globular shape, the LC must unfold before exiting through this membrane pore. This unfolding process is facilitated by a pH differential existing between the cytosol and endosome. Typically, a lower pH increases the helical structure of proteins and a higher pH facilitates unfolding. Unlike most proteins, a lower pH facilitates unfolding of the BoNT LC. The pH of 5 inside the endosome is lower than the neutral pH of the surrounding cytosol (pH 7). This lower pH allows the LC, contained in the endosome, to unfold to exit into the cytosol. Once free in the cytosol, with its higher pH, the LC refolds back into its original 3-D globular shape/structure.
Each serotype of botulinum toxin works by enzymatic cleavage of one or more of the SNARE proteins. The 3-D shape/conformation of each LC serotype is specific for corresponding sites/proteins in the SNARE complex ( Fig. 1 ). The LCs of each of the 7 botulinum serotypes cleaves a distinct peptide bond on one or more of the SNARE proteins. No 2 serotypes act at the same peptide bond. Botulinum toxins A, C, and E cleave the 25-kDa synaptosomal-associated protein SNAP-25; types B, D, F, and G cleave a synaptobrevin vesicle-associated membrane protein (VAMP).
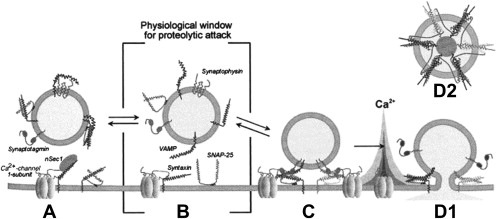
In recent years, evidence has shown that all synaptic neurotransmitter release, including nociceptive neuropeptides, is regulated by this same vesicular release mechanism involving SNARE proteins forming a membrane pore to allow release of a vesicle contents ( Fig. 2 ). This mechanism provides a theoretical basis for the observed effects of BoNTs on pain. The antinociceptive effects of the BoNTs may be produced by blocking SNARE proteins. This block may reduce the release of nociceptive neuropeptides and neurotransmitters involved in pain signaling, chronic inflammatory pain response, and central sensitization of pain. The neuropeptides and neurotransmitters involved in nociception include substance P, glutamate, calcitonin gene-related peptide, and others.
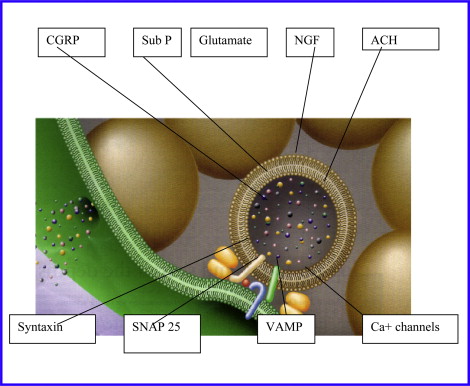
Clinical Effects
Once the SNARE proteins are cleaved, the ACh-containing vesicles cannot dock with or be released from presynaptic membrane, thereby blocking muscle activation or gland stimulation. A corresponding decrease in muscle activity or glandular secretion occurs following blocking. Initial effects are generally noted within 48 to 72 hours with peak effect of the toxin at 4 weeks. The effects of BoNTs are temporary and begin wearing off as the process of reinnervation occurs, reestablishing a functional NMJ/NGJ. The clinical effects gradually begin wearing off as the NMJ/NGJ is reestablished. Effects typically last 3 months but may last much longer in some patients and/or conditions. Retreatment is determined by recurrence of clinical symptoms or problems, but is not recommended before 12 weeks.
Immunogenicity
The BoNTs, like all foreign proteins, can stimulate an immune response and the formation of neutralizing antibodies. The incidence of neutralizing antibodies has become less frequent in recent decades with changes in injection patterns and toxins. The problem of secondary nonresponse (defined as an absent or markedly decreased response in a patient with prior good clinical response) may be caused by issues other than antibody formation. These issues include contractures, change in pattern, and evolution of the disorder. Many patients have been treated successfully for well over a decade with no evidence of secondary nonresponse or antibody formation. When true nonresponse is considered likely, a clinical test for nonresponse rather than antibody titers is most commonly performed. The most commonly performed clinical test is the Frontalis test, which involves injecting a small quantity of BoNT in the frontalis muscle and observing the clinical response/nonresponse (ability to elevate forehead). An alternative is measurement of the compound motor action potential (CMAP) of the abductor digiti minimi pre and post toxin injection. To minimize immune system stimulation repeat BoNT treatment is performed no more frequently than at 12-week intervals. Booster dosing or dosing at intervals shorter than 12 weeks should be avoided. Although there is no specific evidence to support it, most experienced clinicians also recommend avoiding immunizations within 2 weeks of BoNT injections.
Dosing
The BoNTs are biologic agents, and dosing must be customized for the patient’s clinical problem, severity of the tone issues, size of the muscle, and the treatment goals. This customization involves both the art and science of patient evaluation, target identification, and dose selection. Using the minimal effective dose is recommended to avoid immune stimulation and adverse events such as local or regional weakness. The BoNT’s are dosed in bioactive units rather than in weight or volume of toxin. These units of potency are specific for each individual toxin manufacturer; this is true both within and between the different serotypes of toxins that are clinically available. There are no accepted conversion tables when comparing individual products.
Adverse Events
There have been reports of hypersensitivity and anaphylactic reactions to BoNT. History of these events is a contraindication to additional treatment with BoNTs. Reported adverse events include dry mouth and dysphagia, primarily seen in patients with cervical dystonia. Excess weakness in the injected muscle is generally a dosing issue. Weakness may be seen in muscles adjacent to the target or, less commonly, more distant from the site of injection. There have been some case reports suggesting spread of the toxin to sites distant from that injected. Symptoms have included asthenia, diplopia, blurred vision, ptosis, urinal incontinence dysphagia, exerostomia, and breathing problems (see the Food and Drug Administration [FDA] label update).
2010 Toxin Update
Worldwide, the number of botulinum toxin preparations has expanded to include several A serotypes and one B serotype ( Table 1 ). The inherent differences between and even within serotypes requires clinicians have a thorough knowledge of toxin biopharmacology. The differences in potency and dosing between and within serotypes are likely due to manufacturing differences, intracellular targets, and the accessory or accompanying molecules/proteins. Because the BoNTs are biologicals, not drugs, the product from each manufacturer is unique. From a clinical standpoint this mandates dosing that is specific or unique to the individual products. Despite being used in clinical practice, there are no accepted or recommended conversion factors when moving from one toxin product to another. When switching between or within serotypes it is recommended that clinicians return to the recommended starting dose for the new product.
Botulinum Toxin A | Botulinum Toxin B |
---|---|
Botox (onabotulinum toxin A), Allergan, USA | Myobloc/Neurobloc (rimabotulinum toxin B), Solstice, USA |
Dysport (abobotulinum toxin A), IPSEN, UK | |
BTXA (LIBP, China) | |
Puretox (Mentor, USA, Europe, Canada) | |
Reloxin (Ipsen/Medicis, UK/France) |
FDA Updates
In 2009, to reduce the potential for confusion between the different toxin products, the FDA issued unique generic names for each of the 3 botulinum toxin products available in the United States (see Table 1 ). Clinicians and researchers should become familiar with these generic names.
In 2009 the FDA also issued a boxed warning for all BoNT products used in the United States. This warning includes information on possible adverse events and side effects of toxin therapy, including spread of the toxin with distant effects, weakness, swallowing problems, and death. The deaths reported in association with BoNT therapy were in compromised or medically fragile children with cerebral palsy and preexisting swallowing problems. In its investigation, the FDA did not determine a causal link between toxin therapy and the patient deaths. The FDA has mandated that a Risk Evaluation and Mitigation Strategy (REMS) be performed at each treatment session. It is mandated that physicians provide information about the risk of treatment to all patients at each treatment session.
Clinical Applications of the Botulinum Toxins
From the initial approval of botulinum toxin A (oculinum toxin) by the FDA in 1989 for ocular muscle disorders (strabismus and blepharospasm), there has been a seemingly ever-expanding list of approved indications, clinically accepted and proposed uses for the neurotoxins.
A recent Medline search for “Botulinum toxin injection” revealed over 4000 citations in peer-reviewed publications since the mid 1980s. Most physicians are familiar with the approved indications for the botulinum toxins (see Table 1 ). Many clinicians are also familiar with some of the off-label but accepted clinical uses of the botulinum toxins, including the dystonias, muscle overactivity in upper motor neuron syndromes/neurologic disorders, sialorrhea, headache, and muscle spasm ( Table 2 ). Clinicians may be less familiar with many of the novel off-label applications for botulinum toxins, which include treatment of disorders affecting a wide variety of body systems and conditions ( Tables 3 and 4 ).
Approved Indications for BoNT A, Botox (Onabotulinum Toxin A) | Approved Indications for BoNT A, Dysport (Abobotulinum Toxin A) | Approved Indications for BoNT B, Myobloc (Rimobotulinum Toxin B) |
---|---|---|
Strabismus Blepherospam Cervical dystonia Hemifacial spasm Hyperhidrosis Glabellar lines Spasticity, flexor muscles upper extremity | Cervical dystonia | Cervical dystonia |
Body System | Conditions |
---|---|
Dystonia | Primary: focal, task specific, oromandibular, lingual, voice disorders/dystonia, palatal tremor, genetic Secondary: Parkinson’s, tardive dyskinesia |
Gastrointestinal | Achalasia, rectal fissures |
Genitourinary/Gastrointestinal | Overactive bladder, urge incontinence, bladder pain/spasm, interstitial cystitis, sphincter dyssenergy, bladder outlet obstruction |
Musculoskeletal | Myofascial pain |
Neurologic | Muscle overactivity/spasticity/upper motor neuron syndrome, neurogenic thoracic outlet syndrome, facial paralysis, palatal tremor |
Pain | Headache, myofascial pain, muscle spasm |
Neurosecretory | Sialorrhea, Frey syndrome (gustatory sweating) |
Surgical | Postoperative pain/muscle spasm in cerebral palsy, chemoprotection of tendon repairs, limb lengthening |
Body System | Conditions |
---|---|
Dermatologic | Dyshidrosis, epidermolysis bullosa simplex (EBS), Weber-Cockayne type (EBS-WC), multiple eccrine hidrocystomas, hidradenitis suppurativa, hypertrophic scars, multiple cutaneous piloleiomyomas psoriasis |
Gastrointestinal | Intractable constipation, morbid obesity, neurogenic dysphagia, diabetic gastroparesis |
Genitourinary/Gastrointestinal | Benign prostatic hypertrophy, premature ejaculation, dysparenuria, pelvic floor pain, vaginismus |
Head and neck | First bite syndrome, idiopathic rhinitis, bruxism, temporomandibular junction, trismus |
Musculoskeletal | Club foot deformity, lateral epicondylitis,, thoracic outlet syndrome, piriformis syndrome |
Neurologic | Muscle imbalance from obstetric brachial plexus palsy, Schwartz-Jampel syndrome |
Ocular disorders: | Acquired disruption of motor fusion (intractable diplopia) |
Pain | Osteoarthritis |
Surgical | Postoperative pain/muscle spasm, muscle tightness following hip arthroplasty |
Vascular | Thoracic outlet syndrome, Reynaud syndrome |
Physicians who provide BoNT treatment must have an extensive knowledge of anatomy, physiology, and biopharmacology. Additional requirements include clinical skills in problem solving, setting realistic goals, determining the correct target for injection, and dose and dilution of toxin. A prerequisite is the skill needed to isolate the target structure during the procedure. This last step, accurate injection technique, is critical for effective treatment as well as minimizing risk and potential adverse events. Techniques used for muscle localization include anatomic guidance/palpation, electromyography (EMG), electrical stimulation (E-stim), and ultrasound. Each of these techniques has well-documented advantages and disadvantages ( Table 5 ). Techniques other than ultrasound have limited value when muscles are not the target for BoNT injection.
Technique | Advantages | Disadvantages |
---|---|---|
Anatomic/Palpation | Ease of application, no technology required | Limited by experience/training, cannot palpate deep or small structures |
EMG | Widely available, clinician experience, target active area in target muscle | Cocontraction of adjacent muscles may be falsely attributed to a target muscle leading to inaccurate injection, patients may not be able to activate muscle, cannot be used for nonmuscle target |
E-stim | May be more precise than EMG Widely available Inexpensive technology | Cannot be used for nonmuscle targets, painful, requires sedation in most children |
Ultrasound | Precise targeting of structures, More quickly isolates target, smaller needles, can be used for nonmuscle targets, visualize injection/volume of injectate, avoid injection of nontargeted structures | Cost Clinician experience/training Access to equipment |
Ultrasound Applications for BoNT Injection
Ultrasound has been used for many years for procedural guidance. Ultrasound with E-stim is considered the preferred technique for nerve localization for anesthetic or neurolytic nerve blocks. The first reported use of ultrasound to guide BoNT injection was in 1996 in the treatment of achalasia. As physicians have gained experience with ultrasound, it has gained increasing acceptance as one of the preferred, if not the preferred tool for procedural guidance for BoNT therapy. Although each localization technique has its advantages and limitations, clinicians increasingly recognize the benefits ultrasound provides over other techniques. One of the advantages of ultrasound over other localization techniques is its ability to visualize the target of interest and to avoid inadvertent injection of vessels, nerves, or other nontargeted structures. A group of physicians who met recently to discuss a multicenter BoNT trial concluded that ultrasound or ultrasound with E-stim constitute the gold standard for muscle or other target structure localization for BoNT therapy (Katharine Alter, MD, personal communication, 2009).
Ultrasound Technology for BoNT Injection
Although there are several modes of ultrasound available, B mode is used for live procedural guidance. This mode provides direct visualization of the target structure, the needle, and the toxin entering the target. Under direct visualization the needle can be adjusted to avoid nerves, vessels, and tendons, and to redirect the needle (with a single needle stick) to multiple sites within a target. Ultrasound allows the physician to observe the effect of the injection volume in a given target and to redirect the injection to avoid overloading the muscle or target, which could potentially reduce the risk of spread of the toxin outside the target structures. Ultrasound does not require the active participation or cooperation of the patient. Therefore, is has clear advantages in patients with limited motor control or involuntary movements, and in patients who cannot or will not follow directions or cannot cooperate. Identification of muscles and other targets using ultrasound is based on pattern recognition and identifying structures such as bones and vessels adjacent to the target.
Transducers
Because of the size and location of the various target structures, more than one transducer may be required. A 12-5 transducer will allow imaging of most muscles and structures in smaller patients. A 3-5 transducer may be needed for deeper targets or muscles, and large or obese patients. Visualization of superficial or small structures may require the use of a stand-off pad. Adjustment of machine parameters (depth, gain, and time:gain compensation) may be needed to optimize image quality.
Needles
Although etched or large needles are more easily visualized, they are rarely needed or used for BoNT injection. Most clinicians typically use standard hypodermic needles of 25- to 27-gauge. The length of the needle needed can be estimated by a preliminary scan of the target structure when its depth can be ascertained. Some physicians combine EMG or E-stim with ultrasound for some injections. In patients with dystonia, the use of EMG and ultrasound may help target the most active area of the muscle. There is no evidence that proves this technique is more effective than ultrasound alone.
Technique
At the time of the procedure a preliminary scan is performed to identify the structure, its depth, and the presence of neurovascular or other structures to be avoided, thus allowing the clinician to determine the most direct and safest path for needle insertion. The target structure(s) are most often identified in both the transverse and longitudinal orientation with a preliminary scan. This scan allows one to select the optimal approach for the procedure while visualizing the needle tip and structures of interest. Some structures are better viewed in one plane than another. For example, the parotid gland is best viewed in its transverse plane, whereas the submandibular gland is best viewed with the transducer placed along the longitudinal axis of the gland.
Transducers have a long axis and short axis. A needle may be inserted through the transverse (short axis) or longitudinal (long) axis of the transducer. For most procedural guidance, the longitudinal view is preferred, as the entire length of the needle will be visualized (see the article on interventional procedures elsewhere in this issue). In BoNT therapy the short-axis approach is more often chosen, as this frequently allows a more direct approach to the target structure. The needle is inserted at an angle under the ultrasound beam. Care must be taken to keep the needle within the field of view of the transducer; this applies to either the short- or long-axis approach.
Moving the needle up and down or vibrating the needle by hand, without advancing, will help localize the tip of the needle. Moving up and down while advancing to the target also creates movement in the surrounding tissues, which helps localize the needle site and its distance from the target. The transducer must be angled and adjusted throughout the procedure to maintain a good view of the muscle/target and needle. The injection is performed once the target is localized. The amount of injectate at one site can be adjusted based on direct visualization. The skin should be cleaned using the usual technique. A sterile field with sterile gel packets and condom catheters should be used for joint injections. Sterile gel and condom catheters are less frequently used for muscle and other targets.
Ultrasound-Guided BoNT Injections for Specific Conditions
Ultrasound for procedural guidance for BoNT injection has been reported for a wide variety of target structures, most commonly muscle, but increasingly in salivary glands and other structures. This section comprises a review of reported uses of ultrasound for BoNT injections in specific conditions and structures. Many of these applications of BoNT are off label.
Upper motor neuron syndromes
Muscle overactivity is a frequent clinical problem in upper motor neuron syndromes (UMNS) such as stroke, brain injury, cerebral palsy, and multiple sclerosis. Tone disorders include velocity-dependent spasticity and secondary dystonia, which is characterized by sustained postures that have a twisting quality. BoNT therapy (off label in the United States except for flexor muscles in the upper extremity on label in Europe and other countries) is frequently initiated to reduce unwanted muscle activity so as to improve range of motion and passive or active function, and to reduce pain.
Commonly encountered patterns in UMNS include a flexed elbow or wrist in the upper extremity, knee/hip flexion, thigh adduction, and equinus in the lower extremity. Even the forearm muscles where complex overlapping architecture obscures muscle identification can be accurately targeted with ultrasound guidance. Ultrasound is also useful in isolating deep muscles or muscles that have limited surface anatomy and are therefore difficult to isolate with palpation or even EMG/E-stim. Examples of muscles easily targeted with ultrasound include flexor digitorum superficialis, flexor carpi radialis, pronator teres in the upper extremity, and the piriformis, iliopsoas, or tibialis posterior in the lower extremity ( Figs. 3–12 ). To date, the majority of the studies and case reports in the use of ultrasound in UMNS are in children with cerebral palsy.
