Fig. 21.1
The histologic structure of the growth plate
Reserve Zone
This zone, which is the closest one to the epiphysis, contains chondrocytes, either singly or in pairs. These chondrocytes were recently shown to be effective in columnar and one-directional growth of the cells located deeper in the growth plate [4]. The size of the cells in reserve zone is similar to that of the cells in the proliferation zone. In cell staining studies, cytoplasms show strong positivity for glycogen. Electron microscopy studies have shown those cells to be rich in endoplasmic retinaculum, which is an indicator of protein synthesis [5]. The cells are scarcer compared to other zones and are separated from each other with a wider extracellular matrix. The oxygen density is low [6, 7]. Cartilage cells in the reserve zone pass to the proliferation zone with an unknown trigger mechanism.
Proliferation Zone
Cells in this zone have a smoother structure and settle as columns parallel to the long axis of the bone [5]. Oxygen density and the rate of cell metabolism are higher than in other zones. The basic function of this zone is the proliferation of cells, which is one of the basic mechanisms responsible for the longitudinal growth of the bone. The cell at the top of each column is a single cartilage cell displaying the properties of cartilage proliferation and thus it forms the true germinal layer of the growth plate [8]. Besides cell proliferation in this zone, it is also a zone of intense extracellular matrix synthesis [1]. Extracellular matrix synthesis helps cartilage cells separate from each other in the form of columns. The extracellular matrix is formed of collagens, proteoglycans, and other non-collagen proteins. Although the basic collagen of the growth plate is Type II collagen, Type IX and Type XI collagens are also intensively synthesized. Type IX collagen functions in the interaction of Type II collagen with the other matrix components surrounding. Mutations in Type II collagen are known to be related to skeletal dysplasia such as spondyloepiphyseal dysplasia, Kniest dysplasia, and Stickler syndrome.
Aggrecan is a basic proteoglycan of the cartilage matrix formed with the alignment of multiple keratan sulfate and chondroitin sulfate around a core protein [9]. It plays a key role in resistance of the cartilage against the compressive forces [10]. Decorin and biglycan are other matrix components with a proteoglycan structures. It is thought that decorin functions in the regulation of collagen fibril synthesis and biglycan in mineralization [11]. The most important non-collagen matrix protein is cartilage oligomeric matrix protein (COMP). It functions in binding of calcium to the extracellular matrix. Mutations are known to be related to pseudoachondroplasia and some forms of multiple epiphyseal dysplasias [12].
After a specific time period, either the number of cell divisions comes to an end or with the effect of local growth factors the chondrocytes lose their dividing capacity. The cells are differentiated by increasing their diameters and enter the hypertrophic zone [13].
Hypertrophic Zone
The cells that have been smoothed in the proliferation zone take on a spherical shape in the hypertrophic zone, which is the final zone, and their volume increases approximately fivefold compared to the proliferation zone [5]. Parallel to the increase in internal cell volume, an increase is seen in alkaline phosphatase enzyme activity and in the synthesis of Type X collagen, which is only found in the hypertrophic zone. Although the function of Type X collagen is not fully understood, it is known that mutations cause Schmid metaphyseal chondrodysplasia [14]. The dense cytoplasmic glycogen content, which is present at the beginning of the hypertrophic zone, decreases toward the middle of the hypertrophic zone. Oxygen density is low associated with the scarce blood circulation. Under light microscopy, cell cytoplasm takes the form of a cavitary structure. This cavitary structure in the cell cytoplasm increases toward the lower layers of the hypertrophic zone and the nucleus of the cell starts to fragment. The vesicles, which have sprouted from the plasma membrane, accumulate within the matrix between the cartilage cell columns. In the same period, calcium starts to accumulate in these vesicles. Calcium phosphate, hydroxyapatite, and matrix metalloproteinase (MMP), which cause self and surrounding mineralization, are secreted from vesicles. Mineralization and low oxygen pressure attract primary spongious vessels to this zone [15]. Apoptosis starts in the chondrocytes, leaving a scaffold behind for bone formation. With lysosomal activity, endothelial and perivascular cells destroy the transverse cartilage septum, which is found at the very end of the hypertrophic zone [6]. The vast majority of this septum and the lacunae of the cartilage cells, which have undergone apoptosis, are filled with osteoblasts and osteoprogenitor cells. A trabecular network (primary spongiosa) is created from mineralized cartilage and bone matrix. Then, the metaphysis (secondary spongiosa) formed of lamellar bone develops on this covering [6].
Bone Growth and Control Mechanisms
The longitudinal growth of the bone is directly related to the proliferation rate of the cartilage cells in the growth plate. The rate of proliferation of the cartilage cells is controlled by the negative feedback cycle of parathyroid hormone-related peptide (PTHrP), Indian hedgehog (Ihh), and transforming growth factor-beta (TGF-β) expressed from the chondrocytes in the growth plate [11]. This feedback cycle controls the rate of cells, leaving the proliferation zone and turning into hypertrophic cells irreversibly. PTHrP delays hypertrophic transformation in chondrocytes, which have reached to the end of the proliferation zone. Cells, which have started hypertrophic transformation, express Ihh hand this goes back via the perichondrium causing the signal to be forwarded to the periarticular cells in order to the increase PTHrP production. TGF-β expressed from perichondrial cells has a role in the transmission of this signal and PTHrP production is increased [16, 17]. At the same time, by directly affecting cartilage cells, TGF-β can inhibit hypertrophic transformation [18]. The increase in PTHrP causes a decrease in hypertrophic transformation and a reduction in the density of cells that produce Ihh. Thus, the hypertrophic transformation is kept under control. Although it seems as if the basic control mechanism of cell proliferation in the growth plate is the feedback cycle of PTHrP, Ihh and TGF-β, cell proliferation can also be controlled by the local and systemic molecules such as fibroblast growth factor (FGF) and insulin-like growth factor I (IGF-I), which have previously shown to be effective on growth plate cell proliferation.
Chondrocyte hypertrophy has a significant role in longitudinal growth of the bone [19]. Forty-four to fifty-nine percent of the growth in the long bones occurs through chondrocyte hypertrophy and the remainder by extracellular matrix synthesis and chondrocyte proliferation. Hypertrophic chondrocyte dimensions in bones with different growth properties vary together with the growth capacity of the bone. The hypertrophic chondrocytes of the femur, which elongate faster than radius, have been shown to reach greater diameters [20, 21]. Although the reasons for these differences are not known, local, systemic, and genetic factors are thought to have an effect.
Another important function of hypertrophic cells is to prepare the extracellular matrix for calcification. Cartilage cells that have reached the final stage of hypertrophic transformation produce intense amounts of alkaline phosphatase and Type X collagen, which is only found in this zone. Alkaline phosphatase is essential for matrix calcification and is found in dense amounts within the vesicles expressed to the surrounding matrix from the hypertrophic chondrocytes. These vesicles are thought to be where the calcium hydroxy apatite nucleation first starts [22]. Alkaline phosphatase increases the density of phosphate ions, which are necessary for calcification. A deficiency in alkaline phosphatase causes a reduction in matrix mineralization as in hypophosphatasia and the growth plate widens [22]. In the completion of cartilage maturation, bone morphogenetic proteins (BMPs) and receptors are thought to be effective [23, 24]. It has been shown that the cartilage cells undergo hypertrophic transformation when BMP is present in the environment. On the other hand, maturation has been shown to be prevented by BMP inhibition [25]. The other local and systemic factors that are effective on maturation are also thought to have an effect on the cartilage cells through BMP pathway.
Chondrocytes are metabolically active cells that synthesize the extracellular matrix. The matrix undergoes calcification starting from the vesicles expressed from the cartilage cells and these calcified areas function as a template to osteoblasts for bone formation. The calcium accumulation in the matrix vesicles is dependent on a family of calcium channel molecules, which is called Annexins. Both Type II and Type X collagen are effective in the calcification process, causing activation of Annexin-V.
While it was previously thought that a reduction of oxygen pressure in the environment and the necessary nutritional substances resulted in death of hypertrophic chondrocytes, today it is known that those cells also die as a result of a programmed process [26]. Although the physiological mechanisms regulating programmed cell death (apoptosis) in the growth plate have not yet been fully clarified, the relationship of the chondrocytes with the extracellular matrix, various growth factors, and cytokines are thought to affect the process. Extracellular matrix mineralization causes an increase in the phosphate ion concentration in the environment. In vitro studies have shown that increased phosphate ion concentration triggered apoptosis in mature cartilage cells [27].
Controlling the velocity of bone growth comprise a process containing several factors that are effective together. Genetic differences, systemic hormone levels, local effects of growth factors and cytokines, nutritional and vascular status of the growth plate, and the mechanical forces are effective at the cellular level and control the growth rate.
Several cellular events simultaneously occur within the growth plate during the longitudinal growth of bones. While the cells in the proliferation zone are being divided, cells in the hypertrophic zone expand and produce extracellular matrix. Cells in the provisional calcification zone complete their apoptosis process and are replaced by calcified cartilage. Of these cellular events, cartilage cell proliferation rate and extracellular matrix synthesis and expansion of the cells are the key mechanisms providing longitudinal growth.
Clinical Relevance
Guiding Growth Plate as a Means of Treatment Modality
The Hueter-Volkmann law explains the effects of mechanical forces on the growth plate. This describes how compressive forces on the growth plate decrease its activity and inhibits its growth. However, tensile, torsional, and bending forces are also known to have an effect on growth plate activity [28, 29].
Mechanical loading has been shown to have an effect on growth through cell proliferation, cell expansion, and transformation [30]. Compressive forces have been shown to reduce the number and ratio of proliferating chondrocytes in rabbit and rat growth plates [31]. On the other hand, reduction of the loads on the growth plate or the application of distraction forces causes widening of the growth plate [30]. The basic mechanisms achieving widening have been shown to be an increase in the number, height, and volume of chondrocytes [32] (Fig. 21.2).
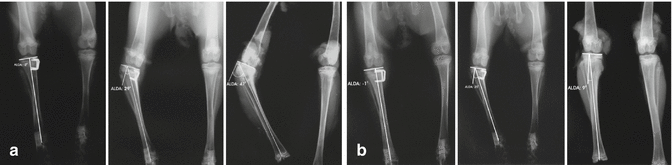
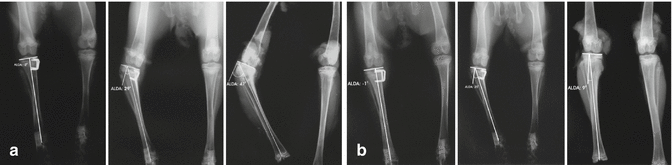
Fig. 21.2
The asymmetrical suppression of the activity of the growth plate in experimental models creates bone deformities (a). The deformity improves spontaneously after the suppression is removed (b)
The effect of mechanical forces on genes and proteins, which function in cartilage formation, has been studied in animal models. It has been shown in the growth plates of rat tibia that compressive forces decreases Type II collagen gene expression in the hypertrophic zone, and Type X collagen gene expression is limited in the lower layers of the hypertrophic zone [33]. However, in a study where chickens roaming free within a cage were loaded with a sandbag, despite the narrowing of the area, where these two collagen types were synthesized, no difference was determined in the gene expression of those proteins [34].
< div class='tao-gold-member'>
Only gold members can continue reading. Log In or Register a > to continue
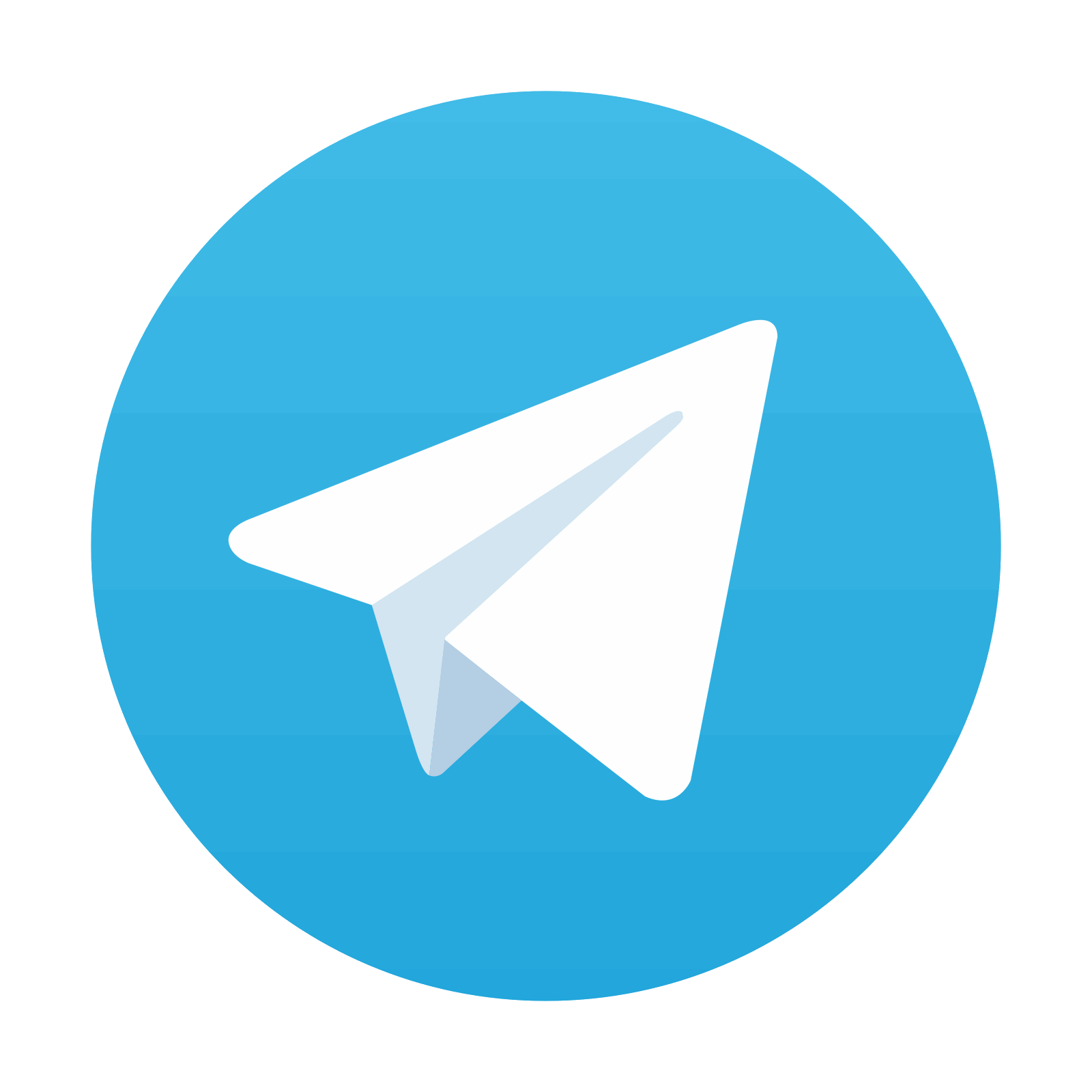
Stay updated, free articles. Join our Telegram channel

Full access? Get Clinical Tree
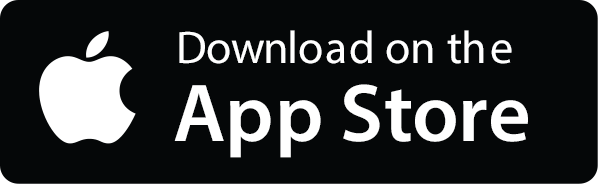
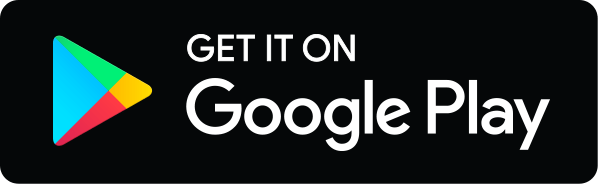