Fig. 8.1
Diagram of quadriceps tendon-patella bone (QTPB) harvesting. PT denotes patellar tendon [119]
Allograft
The American Orthopaedic Society for Sports Medicine (AOSSM) has estimated that approximately 60,000 allografts were used in knee reconstruction procedures alone in 2005 [82]. Because of potential graft necrosis and the relatively large size of the native PCL , larger graft options are preferred for allograft PCL reconstruction . The Achilles tendon (Figs. 8.2 and 8.3), with its large cross-sectional area, is currently the most frequently used graft for acute (43 %) and chronic (50 %) PCL reconstructions [78] due to its large size. Double-stranded anterior and posterior tibial tendons (Figs. 8.4 and 8.5) are also commonly used allografts. Other allograft options include B-PT-B (Fig. 8.6), hamstrings (Fig. 8.7), and QTB (Figs. 8.1 and 8.8).
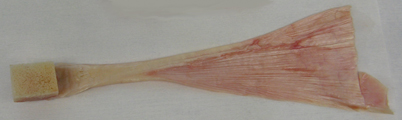
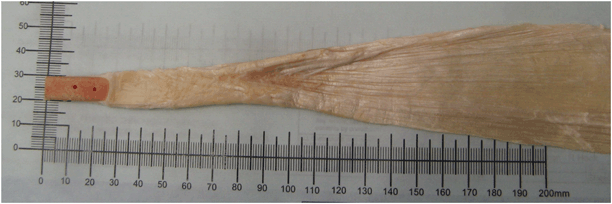

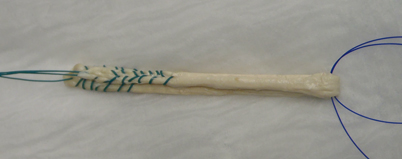
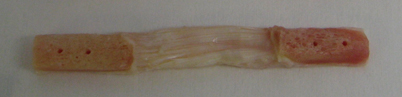
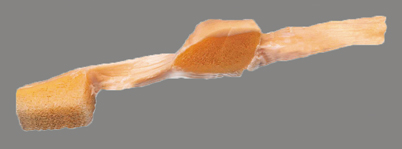
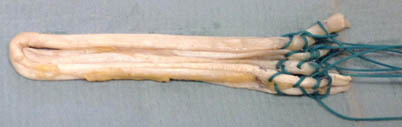
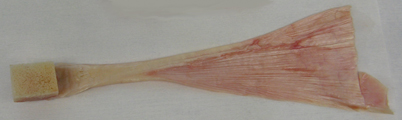
Fig. 8.2
Achilles tendon–bone allograft removed from package. Image kindly provided by Musculoskeletal Transplant Foundation (MTF) [120]
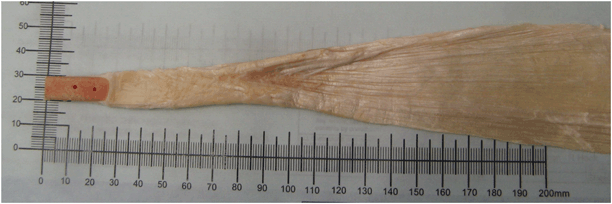
Fig. 8.3
Achilles tendon–bone allograft being prepared for implantation. Image kindly provided by Musculoskeletal Transplant Foundation (MTF) [120]

Fig. 8.4
Tibialis anterior allograft. Image kindly provided by Musculoskeletal Transplant Foundation (MTF) [120]
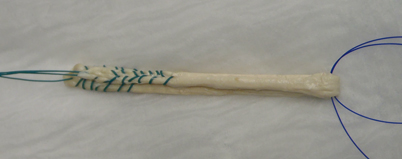
Fig. 8.5
Tibialis anterior allograft ready for implantation. Image kindly provided by Musculoskeletal Transplant Foundation (MTF) [120]
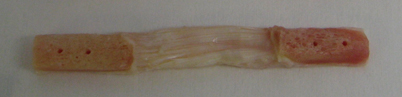
Fig. 8.6
Bone–patellar tendon–bone allograft ready for implantation. Image kindly provided by Musculoskeletal Transplant Foundation (MTF) [120]
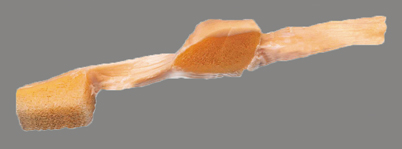
Fig. 8.7
Quadriceps tendon–patellar bone–patellar tendon–tibial bone allograft after removal of packaging [120]
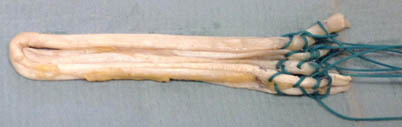
Fig. 8.8
Quadrupled hamstrings allograft
Surgeons are attracted to allograft ligament reconstructions because they eliminate donor-site morbidity as well as the additional risks associated with autograft tissue harvest. Furthermore, allografts provide multiple graft size options, shorter operative and tourniquet times, as well as fewer incisions as a result of not needing to harvest autograft tissue [22, 27, 83, 84]. Unfortunately, the use of allograft tissues is also associated with its own set of complications, such as small risk of infectious disease transmission, slower incorporation of graft tissue, and the potential for immunologic rejection [1, 21, 34, 85–91].
Risk of Infectious Disease Transmission
Infectious disease transmission, albeit exceedingly rare, is a distinct possibility when implanting allograft musculoskeletal tissues and there have been multiple documented cases of disease transmission in this manner, some of which have resulted in the death of the patient [1]. It is possible to transmit human immunodeficiency (HIV) virus type 1 and type 2, HBV, HCV, bacteria, such as clostridia or treponema pallidum, fungi, parasites, West Nile virus (WNV), and human transmissible spongiform encephalopathies.
The risk of HIV transmission in a properly screened donor ranges between 1 in 173,000 and 1 in 1 million and the corresponding risk of HCV is 1 in 421,000 for unprocessed tissue [1]. The most concerning incident regarding HIV transmission in the setting of allograft ligament implantation was in 1986 when a fresh-frozen B-PT-B allograft, which was not secondarily sterilized and was derived from a young male donor with no known risk factors for HIV whom tested negative for HIV-1 antibodies, was implanted into a patient [86]. Three weeks following surgery the recipient was treated with supportive therapy for flu-like illness and lymphopenia was noted. The patient was not diagnosed with HIV until several years later after an investigation was carried out to identify the cause of seroconversion in a woman whose only risk factor for HIV was the receipt of bone allograft from the same donor. Other non-musculoskeletal allografts from the same donor also resulted in disease transmission. At the time of this incident, HIV testing of donors was performed via detecting the presence of anti-HIV antibodies, which may take several months to become detectable in the peripheral blood of recently infected individuals [86]. Currently, nucleic acid testing (NAT) is now required by American Association of Tissue Banks (AATB). HIV, although it is a retrovirus, synthesizes DNA that is detectable within the leukocytes it infects and NAT can be carried out effectively within 48 h of a donor’s death. In addition to this case of HIV transmission, there have been at least two separate documented reports of hepatitis C transmission as a result of receiving patellar ligament allografts from infected donors [92, 93]. Again, these incidents occurred as a result of harvesting tissue from an anti-HCV antibody negative donor where NAT was not performed. Although the pool of allograft donors who fall into the category of anti-HCV antibody negative yet HCV-RNA positive is unknown, in 2003 this serology pattern was present in approximately four out of every one million blood transfusion donors [92]. Although sterilization of allografts will be discussed later, it should be noted that studies have demonstrated that although freeze-drying and radiation may decrease the already low risk of HIV transmission it does not eliminate this risk completely [86, 94, 95].
In addition to viral transmissions, several bacterial infections have resulted from musculoskeletal allograft implantation [1, 96]. Allograft tissue s distributed by vendors operating with questionable standards that occurred between 2001 and 2005 prompted the FDA to require more stringent surveillance of organizations procuring allograft tissue. As a result, all tissue banks are now required to register with the FDA and follow Current Good Tissue Practice requirements designed to minimize risk to allograft recipients [1, 96]. These examples bring three points to light: (1) there is a definite time lag between a donor contracting a virus and our current ability to detect its presence (approximately 7–10 days with NAT testing), (2) secondary processing and sterilization processes have the potential to effectively decrease the risk of viral disease transmission yet, and (3) there will always be a finite risk to patients when implanting musculoskeletal allografts [1, 97].
As mentioned previously, the risk of HIV and HCV is exceedingly low and the authors are unaware of any documented transmissions in the setting of appropriately screened donors and modern NAT. Additionally, an investigation by Greenberg et al. in a large series of patients failed to demonstrate an increased risk of bacterial disease transmission associated with implantation of allograft tissues [98]. Again, this underscores the importance of the surgeon becoming knowledgeable about the procurement practices of their allograft provider so that the surgeon can help patients make informed decisions about their care.
Delayed Incorporation of Allograft
Healing of a ligament graft occurs in three phases: inflammatory, proliferative, and remodeling. Within the inflammatory phase, neutrophils and other inflammatory cells arise and the water content of the graft increases ultimately leading to decreased biomechanical properties of the tendon itself. Graft necrosis then occurs, which is believed to be the cause of the permanent strength loss observed in reconstructed ligaments, when compared to their biomechanical strength at the time of implantation [87]. Next is the proliferative phase in which fibroblasts and synovial cells infiltrate the graft from the bone tunnels and vascular granulation tissue engrafts into the ligament matrix. Finally, the disorganized fibroblast and extracellular matrix mass is reorganized into a more highly cellular tissue with tensile-strength properties. This process is termed “ligamentization.” Although a similar pattern of revascularization and incorporation of the graft with host tissue occurs among both autograft and allograft tissues, it has been well documented that autograft tissues incorporate faster than allograft tissues [87–90, 99]. It may take up to one and a half times longer for allograft to completely remodel and gain comparable strength to autograft [100]. ACL retrieval studies at autopsy suggest that allograft incorporation continues for more than 2 years [101]. Despite the slower rate of incorporation, the eventual healing is almost identical to the healing of autograft [102, 103]. Inherent to this delayed incorporation is the potential for graft rejection. Although this has been reported in musculoskeletal allograft, it rarely impacts the clinical course of the patient [104, 105].
Procurement of Allograft Donor Tissue
The screening of acceptable donors is quite rigorous as this is the first barrier to preventing disease transmission. Prospective donors or their relevant family begin by completing a questionnaire detailing their medical, social, and sexual history. An inquiry is made regarding drug use, neurologic diseases, autoimmune diseases such as rheumatoid arthritis, metabolic disease, collagen disorders, and exposure to hepatitis, HIV, or Creutzfeld–Jacob disease, or unprotected anal sex. Any positive response disqualifies them as a donor. Next, a thorough physical exam is performed, evaluating for signs of infectious diseases such as sexually transmitted diseases, hepatosplenomegaly, lymphadenopathy, thrush, and skin lesions. Again, any positive findings disqualify the donor. Next, a blood sample is obtained. The FDA requires that recovered tissue must be negative for HIV-1 NAT, HCV NAT, and hepatitis B core antibody. American Association of Tissue Banks (AATB)-accredited banks require additional testing for HIV type 1 and type 2 antibody, hepatitis B surface antigen, total antibody to hepatitis B core antigen (IgG and IgM), HTLV-I/HTLV-II antibody, HCV antibody, a syphilis assay, as well as NAT for HCV and HIV-1. Tissues are then harvested using sterile techniques within 15 h of asystole for an unrefrigerated donor or within 24 h of asystole for refrigerated donors. Specimens are contained in wet ice for transport with a maximum of 72 h on wet ice before transfer to colder environment is required [1, 96, 97].
Sterilization of Allografts
In 2006, a survey of 365 members of the AOSSM indicated that 86 % of them utilized allografts, yet 21 % were not aware of whether their allograft source was accredited by the AATB [1]. Furthermore, the vast majority of surgeons surveyed believed that the sterilization process had deleterious effects on the biomechanical strength of these allograft tissues. Gamma irradiation to 1.5 mrad, combined with antibiotic soaks, is a common method of sterilization. Yet, gamma irradiation to a level of greater than 3.5 mrad is estimated to be required to eliminate HIV [95]. Furthermore, gamma irradiation above 3 mrad has been shown to decrease allograft maximum failure force by up to 27 % and strain energy to maximum force by up to 40 % and, as a result, doses below 2.5 mrad are currently recommended to prevent damage to graft biomechanical properties [97, 106]. In response to this, research involving the use of free radical scavengers in conjunction with radiation is currently underway in order to balance adequate prevention of infectious disease with the preservation of biomechanical properties [107].
Ethylene oxide (EtO) was formerly a commonly implemented sterilization technique. However, after an association of a resultant chronic inflammatory reactions (effusions) and increased graft failures with its use was demonstrated, it was eliminated from AATB approved tissue banks [108, 109].
There are many other proprietary sterilization techniques involving serial soaks alternating tissue-culture-grade water with denatured 70 % ethanol, biologic detergents, dimehtylsulfoxide, antibiotics, or hydrogen peroxide. Additional treatments may consist of ultrasound, centrifugation, and repeated irradiation cycles [96]. Some tissue banks with proprietary sterilization techniques claim that tissue integrity is not damaged by the sterilization processes [110]. However, sterilized grafts have been associated with poor clinical outcomes in several investigations [111–113].
Storage of Allograft
Cryopreservation is a process of slowly cooling a graft while extracting the intracellular water using various chemical soaks such as dimethylsulfoxide or glycerol. Following the chemical soaks, a controlled rate of progressive freezing to −135 °C is carried out, with the graft ultimately being stored at −196 °C for up to 10 years. This controlled freezing in cryoprotectant solution inhibits the formation of ice crystals and thus preserves collagen integrity. It was theorized that this would also preserve cellular integrity and thus be associated with an increased risk of graft rejection. However, a minimal histological inflammatory response at the allograft ligament as well as normal, rather than accelerated, rejection of corresponding allograft full-thickness skin graft was demonstrated. This, as well as a complete absence of donor DNA by 4 weeks post-transplantation, indicated that there was minimal cell survival among these cryopreserved allografts [113].
Fresh-frozen treatment of allografts is the most commonly utilized storage modality and consists of rapid freezing of the graft to −80 °C or −100 °C without additional sterilization processing. It has been shown to eliminate cellular components that lead to immunologic rejection of allograft tissue [88]. Freeze-dried samples are created by removing the marrow and blood from the specimen and freezing the tissue for a quarantine period. After quarantine, the tissues are thawed, treated with antibiotic soaks, and exposed to serial alcohol rinses in order to dehydrate the specimens. They are subsequently lyophilized and packaged. The resultant graft can be stored for up to 5 years. There is very little immunogenic response when implanted. However, unlike freeze-dried bone, the biomechanical properties of freeze-dried tendons have been demonstrated to be inferior to fresh-frozen specimens and the potential for viral disease transmission is not completely eliminated [94, 114, 115].
Author’s Recommendation
It is clear that allograft tissue plays a substantial role in PCL reconstruction . Any surgeon utilizing banked tissue should become familiar with the practices, protocols, and proven results of whichever allograft vendor is to be utilized. Some organizations providing allograft tissues surpass the requirements of the AATB and US Food and Drug Administration (US FDA). It is our recommendation that surgeons, at the very least, utilize allograft tissues from organizations whose processing and distribution comply with all of the required AATB and US FDA criteria for current good manufacturing practices. Furthermore, surgeons should be familiar with any sterilization processes used for grafts which will be implanted. Because of the potential deleterious effects of the sterilization processes on both the biomechanical and biological properties of allografts, the authors currently utilize only fresh-frozen nonirradiated allografts from an AATB member tissue bank. Routine culturing of allograft tissue in the operating room immediately prior to implantation is not currently recommended because there is little correlation with swab culture results and future allograft-associated infection [1, 116].
Conclusion
Graft selection in PCL reconstruction remains controversial, as there is a relative paucity of research on graft options for PCL reconstruction as compared to ACL reconstruction. While much of the knowledge of graft selection is based upon the experience with ACL grafts, the PCL is biomechanically different from the ACL [117, 118], and thus the results of specific graft use in PCL reconstruction may vary from those of the ACL [13]. To date, the literature has not shown significant differences in clinical outcomes with the use of autograft versus allograft or among the different types of each graft. Thus, the patient’s specific characteristics and goals should be considered to help the patient make an informed decision.
References
1.
McAllister DR, Joyce MJ, Mann BJ, Vangsness CT. Allograft update: the current status of tissue regulation, procurement, processing, and sterilization. Am J Sports Med. 2007;35(12):2148–58.PubMed
2.
Lee YS, Jung YB. Posterior cruciate ligament: focus on conflicting issues. Clin Orthop Surg. 2013;5(4):256–62.PubMedCentralPubMed
3.
Jung YB, Jung HJ, Kim SJ, Park SJ, Song KS, Lee YS, et al. Posterolateral corner reconstruction for posterolateral rotatory instability combined with posterior cruciate ligament injuries: comparison between fibular tunnel and tibial tunnel techniques. Knee Surg Sports Traumatol Arthrosc. 2008;16(3):239–48.PubMed
4.
Levy BA, Dajani KA, Whelan DB, Stannard JP, Fanelli GC, Stuart MJ, et al. Decision making in the multiligament-injured knee: an evidence-based systematic review. Arthroscopy. 2009;25(4):430–8.PubMed
5.
Tzurbakis M, Diamantopoulos A, Xenakis T, Georgoulis A. Surgical treatment of multiple knee ligament injuries in 44 patients: 2–8 years follow-up results. Knee Surg Sports Traumatol Arthrosc. 2006;14(8):739–49.PubMed
6.
Harner CD, Waltrip RL, Bennett CH, Francis KA, Cole B, Irrgang JJ. Surgical management of knee dislocations. J Bone Joint Surg Am. 2004;86-A(2):262–73.PubMed
7.
Fanelli GC, Orcutt DR, Edson CJ. The multiple-ligament injured knee: evaluation, treatment, and results. Arthroscopy. 2005;21(4):471–86.PubMed
8.
Ranawat A, Baker CL, Henry S, Harner CD. Posterolateral corner injury of the knee: evaluation and management. J Am Acad Orthop Surg. 2008;16(9):506–18.PubMed
9.
LaPrade RF, Johansen S, Agel J, Risberg MA, Moksnes H, Engebretsen L. Outcomes of an anatomic posterolateral knee reconstruction. J Bone Joint Surg Am. 2010;92(1):16–22.PubMed
10.
Montgomery SR, Johnson JS, McAllister DR, Petrigliano FA. Surgical management of PCL injuries: indications, techniques, and outcomes. Curr Rev Musculoskelet Med. 2013;6(2):115–23.PubMedCentralPubMed
11.
Nancoo TJ, Lord B, Yasen SK, Smith JO, Risebury MJ, Wilson AJ. Transmedial all-inside posterior cruciate ligament reconstruction using a reinforced tibial inlay graft. Arthrosc Tech. 2013;2(4):e381–8.PubMedCentralPubMed
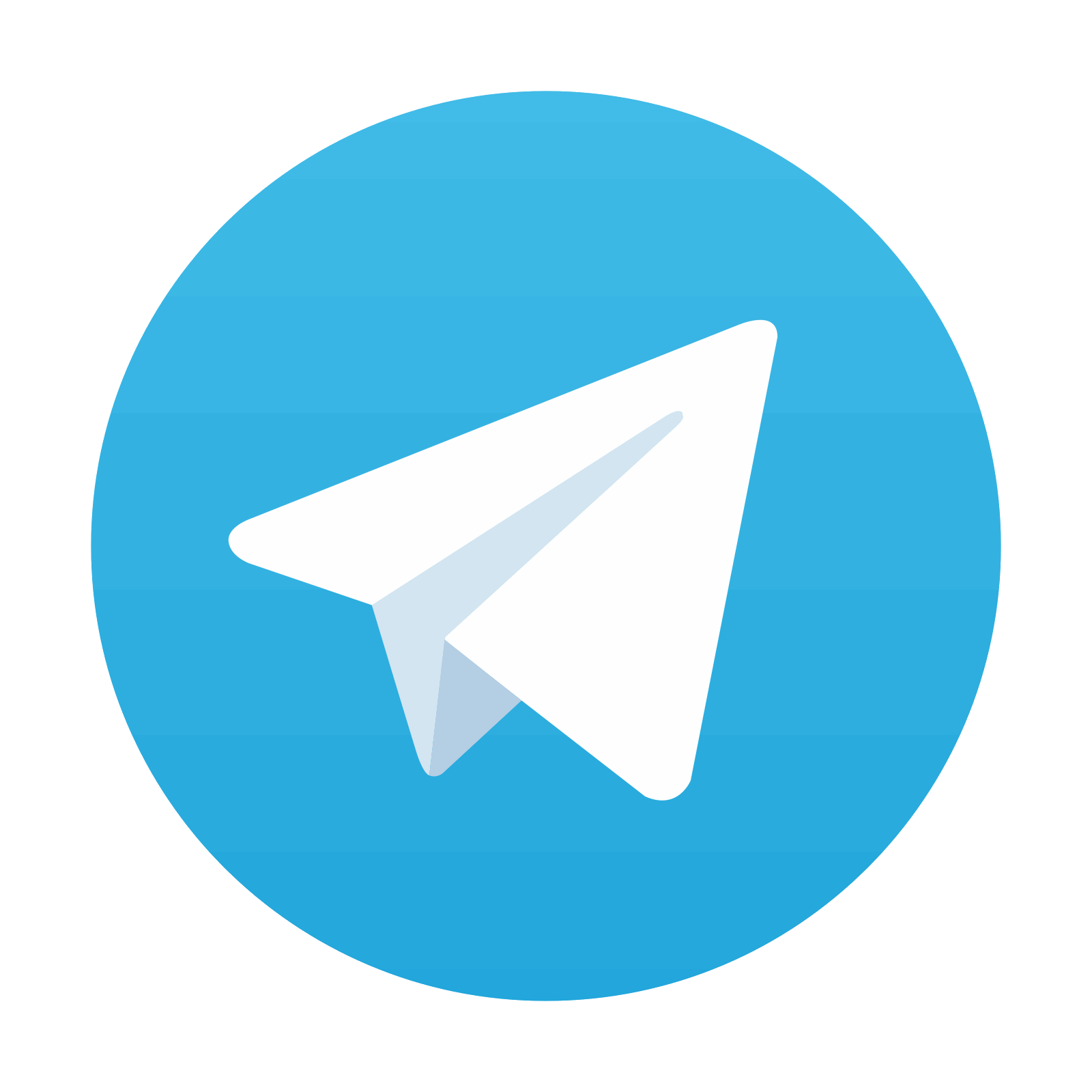
Stay updated, free articles. Join our Telegram channel

Full access? Get Clinical Tree
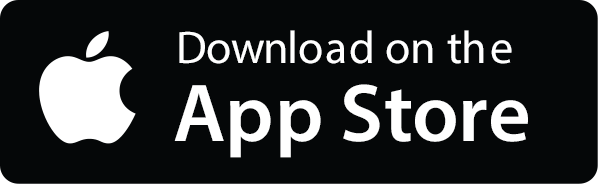
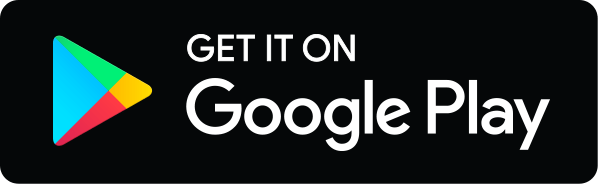