1.1 General anatomy of the muscle fasciae The soft connective tissues associated with muscle tissue can be referred to as muscle fasciae (MF). A recent description of MF structure and function in both skeletal muscle and cardiac muscle (Purslow 2008) lists 15 previous reviews in addition to a great deal of original source material, and the reader is referred to these substantial sources for detailed information. Here we shall summarize the main features of their structure, composition, and functional properties. There is a tendency in previous literature to call MF “tubes” or “sheaths” that surround each fiber or fasciculus. The concept of muscle fasciae as connective tissue structures provides a better understanding of the functional anatomy of these structures. MF form a three-dimensional matrix that is continuous throughout the entire organ, providing connections between fibers and fascicles, rather than separating them. The following description of the general structure of fasciae associated with striated muscles summarizes the consensus of a number of sources and is schematically shown in Fig. 1.1.1. Generally speaking, these connective tissue layers are composed of collagen fibers (and occasionally also elastin fibers) in an amorphous matrix of hydrated proteoglycans (PGs), which mechanically links the collagen fiber networks in these structures. Listrat et al. (2000) and Passerieux et al. (2006) identified seven molecular types of collagen in muscle (types I, III, IV, V, VI, XII and XIV). Types I, III and V are fibrillar collagens (fiber-forming types). Types I and III are the most prevalent in mammalian striated muscle. Types XII and XIV are thought to act as molecular bridges connecting the fibrillar collagens to other components in the amorphous matrix. The basement membrane layer of the muscle fibers contains non-fibrous type IV collagen, together with proteoglycan components such as laminin and fibronectin and heparin sulfate-containing PGs, and forms the boundary between the phospholipid cell membrane and the collagen fiber networks of the “reticular layer” of the endomysium. Collagen fibers are mechanically stabilized by the formation of covalent crosslinks. The formation of crosslinks is essential for the mechanical strength and stiffness of collagen fibers, as without them the collagen molecules slide past each other under load and the fibers have no strength. Throughout gestation and during postnatal maturation there are substantial changes in the types and amounts of covalent crosslinks that mechanically stabilize the collagen molecules in muscle fasciae. The subject of crosslink formation during maturation and aging of connective tissues is reviewed in excellent detail by Avery & Bailey (2008). Here, we shall just note that muscle fasciae are rich in covalent crosslinks, that these crosslinks are known to undergo maturation changes in both endomysial and perimysial connective tissue, and that compounds promoting glycation crosslinks can be incorporated into the body from dietary sources and from tobacco smoke. Thus, diet and lifestyle may conceivably affect the mechanical properties of some connective tissues, including muscle fasciae, via crosslinking of collagens. The amounts and composition of muscle fasciae vary between different muscles in the body. A comparison of transverse sections through different muscles from the same species (Purslow 2005, Fig. 1.1.2) shows that the continuous perimysial network surrounds or separates fascicles of very different sizes and shapes in different muscles. This difference also results in different thicknesses of perimysial connective tissue. These variations, especially in the amount and spatial organization of the perimysium, have long been attributed to variations in mechanical roles of different anatomical muscles. If this is correct, then the muscle fasciae must play strong roles in the normal physiological functioning of each muscle. Some possible explanations of these roles are emerging but are far from complete. 1. At the surface of the fiber is the plasma membrane (plasmalemma) of the muscle cell, which is approximately 9 nm thick. 2. Outside the plasma membrane is the endomysial basement membrane. It is approximately 50–70 nm thick and is composed of two layers: the lamina lucida (or lamina rara) next to the plasma membrane, and an outer lamina densa. 3. The collagen fiber network (or reticular) layer, comprised of a network of collagen fibrils and fibers in a proteoglycan matrix. Schmalbruch (1974) reported reticular layer thicknesses of 0.2–1.0 μm in frog sartorius muscle. As shown by classical transmission electron microscopy images of longitudinal muscle sections (Trotter & Purslow 1992, Fig. 1.1.2), the thickness of the endomysium varies with muscle length, becoming thicker at short muscle lengths and thinner as the muscle is extended. The fibrous reticular layer is a common structure shared between adjacent muscle cells and forms a continuous network that runs across the whole muscle fascicle. Muscle cells (with their individual plasma membranes and basement membranes) occupy the polygonal “holes” in the endomysial network. The reticular region of the endomysium is often described as a random or quasi-random network of irregularly wavy fine collagen fibers. The network is not truly random. There is a preferred direction in the wide distribution of collagen fiber orientations, and this preferred orientation changes with muscle length (Purslow & Trotter 1994). A large number of muscles in animals from many phyla contain muscle fibers which are not continuous along the entire length of fascicles, from tendon to tendon. Trotter (1993) lists 28 studies on a wide range of muscles from humans, mammals and birds showing series-fibered architecture. Muscle fibers in series-fibered muscles are relatively short compared to the length of the fascicle, particularly so in avian species where individual fibers can be as short as 0.4–2.6 cm. The endomysium is the only structure that links these muscle fibers together in the fascicle. Transmission of tension generated in intrafascicularly terminating fibers to the ends of the fascicles necessitates transmission of force through the endomysial network, as this is the only structure continuously linking the fibers. The endomysium is very compliant to tensile forces acting within the plane of the network and so can easily deform to follow the length and diameter changes of muscle fibers in contracting and relaxing muscles. However, the transmission of force between adjacent muscle fibers by shear through the thickness of the endomysium (translaminar shear) is a different property, and one that turns out to be an efficient force transduction pathway (Trotter & Purslow 1992; Purslow & Trotter 1994; Trotter et al. 1995). Any linkage that transmits force from intrafascicularly terminating muscle fibers to tendinous attachments must not deform too much in order to be efficient. Especially in isometric muscle contractions, any significant stretching in the length of the fascicle due to stretchy connections would result in a very poor transmission of contractile force. Purslow (2002) showed that the displacements along the long axis of the muscle due to translaminar shearing of the endomysium are insignificant. The functional significance of this is that the endomysium provides a shear linkage of force from one muscle cell to its neighbors which is highly efficient while still being able to deform easily in the plane of the network so as to allow the muscle fibers to change length and diameter as they contract and relax. The functional anatomy of the endomysium linking adjacent muscle fibers in continuous fibered muscles appears identical to that of endomysium in series fibered muscles. The obvious inference is that load sharing between adjacent muscle cells is a common function in both continuous-fibered and series-fibered striated muscles and even cardiac muscle (Purslow 2008). The endomysium, therefore, forms a continuous three-dimensional connecting matrix which tightly shear-links adjacent fibers together to coordinate force transmission in a fascicle and keep fibers in uniform register.
Introduction
General structure and composition of muscle fasciae
Functional anatomy of the endomysium
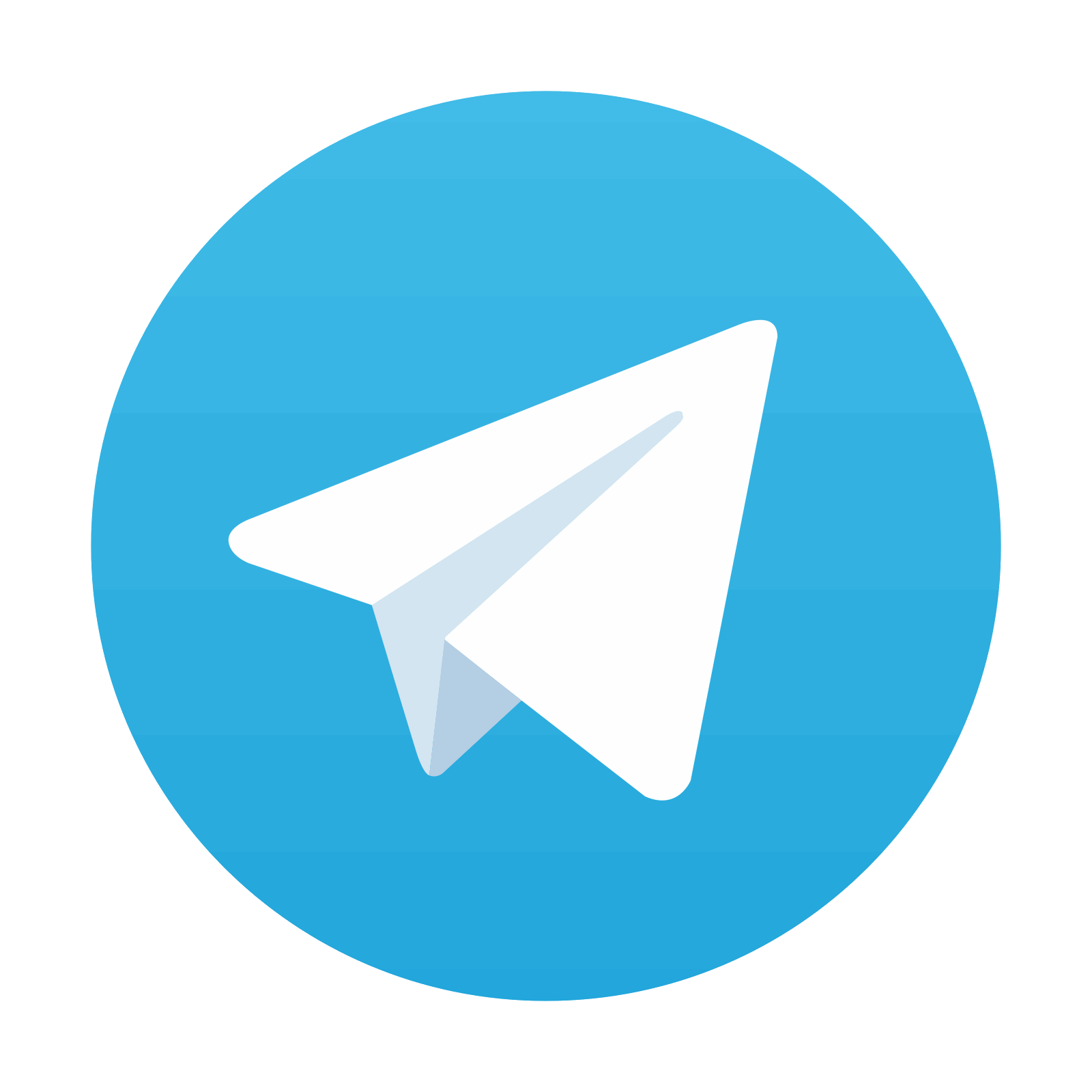
Stay updated, free articles. Join our Telegram channel

Full access? Get Clinical Tree
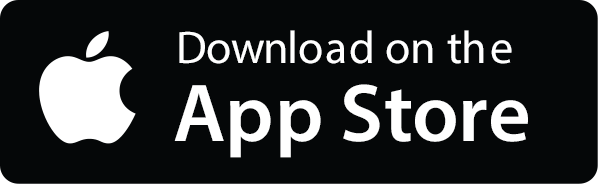
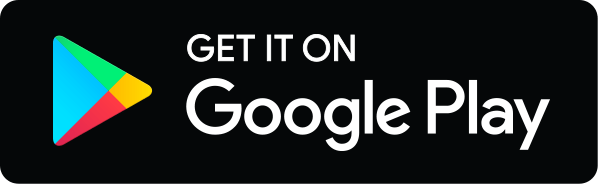