Fig. 37.1
Equinoxe reverse shoulder (Exactech; Gainesville, FL)
A recent radiographic study demonstrated the Equinoxe to have scapular notching rate of 13.2 % in 151 patients at a mean follow-up of 28.3 ± 5.7 months (range 24–44), with only 2.6 % grade 2 notches and no grade 3 or 4 notches [17]. Another large-scale radiographic study demonstrated that 26 of 256 patients (10.2 %) had a scapular notch (20 grade 1 and 6 grade 2; no grade 3 or 4 notches observed), where 38-, 42-, and 46-mm glenospheres had a notching rate of 14.2 % (16 grade 1 and 6 grade 2), 4.4 % (4 grade 1), and 0 %, respectively, at an average follow-up of 22.2 ± 8.7 months (range 10–44) [16]. In both studies [16, 17], patients with notching had baseplates implanted significantly higher on the glenoid than patients without notching. Based on these results, it is recommended that the Equinoxe baseplate be implanted 1–2 mm below the inferior rim of the glenoid to avoid notching. These results represent a significant reduction in the reported scapular notching rate of the Grammont design, which has an average reported scapular notching rate of 68.2 % [2–5, 8, 12, 18, 19].
Design Goal #2: Reduce the Aseptic Glenoid Loosening Rate
Aseptic glenoid loosening was the historical failure mode of pre-Grammont reverse shoulder designs that did not utilize a hemispherical glenosphere to minimize torque on the glenoid fixation surface [2, 20, 21]. The Equinoxe RTSA leveraged the Grammont clinical history by maintaining the center of rotation (CoR) near the face of the native glenoid and incorporated the optimization analysis recommendations for ideal CoR placement 2 mm lateral to the native glenoid. To neutralize this slightly increased torque on the fixation interface, the baseplate surface contact area with the native glenoid was increased by changing the shape, size, and backside curvature from the circular 29-mm-diameter flat-back Grammont design. Additionally, by minimizing humeral liner impingement (by the aforementioned design changes), polyethylene wear is reduced, thereby reducing particulates that are the primary osteolytic agents in aseptic loosening [22].
The Equinoxe baseplate is an oval 25 × 34 mm curved-back design (Fig. 37.2). The design evolution of the glenoid prosthesis with anatomic shoulder arthroplasty (ATSA) started with circular profile devices that ultimately converged to an anatomic pear-shaped profile device. For the same reasons, the Equinoxe baseplate is superiorly elongated in the primary loading direction to neutralize any destabilizing action of the deltoid. By superiorly elongating the baseplate, the surface contact area is increased, affording the possibility to reduce the anterior/posterior width from 29 to 25 mm and facilitate a more anatomic fit in smaller glenoids [23]. Recent work evaluated 8 baseplate designs and demonstrated that the Equinoxe had the largest surface contact area on the backside of the plate, 20 % larger than the next largest baseplate [24]. The screw footprint is maximized by positioning the screws to the edge of this enlarged periphery and increasing the number of screw options from 4 to 6 to provide surgeons with additional intra-operative flexibility. To maximize the length of screws used to achieve fixation, poly-axial compression screws that provide 20° of angular variability are utilized and each screw is locked with a cap to prevent backing out. The importance of screw angular variability is emphasized by the results of a recent study using an alternative baseplate design with fixed-angle locking screws [25]. In that cadaveric study, only very short screws (13–15 mm) were able to be utilized anteriorly and posteriorly prior to bicortical purchase. The orientation of those screws violated the subscapularis muscle belly in 20 % of the inferior screws, 50 % of the superior screws, and 100 % of the anterior screws, and most concerning, 30 % of posterior screws damaged the suprascapular nerve or artery [25].
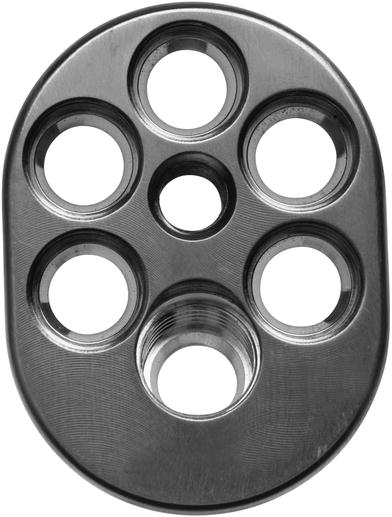
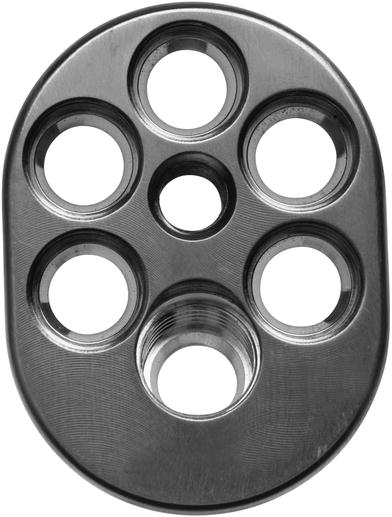
Fig. 37.2
Equinoxe glenoid baseplate
The Equinoxe baseplate has a 2-in.-radius curved backside geometry that closely matches the native glenoid curvature preserving cortical and cancellous bone and increases cortical bone contact to maximize baseplate support [23]. Recent work quantified the cortical and cancellous glenoid bone removed by 3 different commercially available RTSA prosthesis designs [26]. In that study, the DJO RSP removed the most overall glenoid bone (3.7 cm3) despite having the smallest baseplate, the DePuy Delta III removed the second most (3.6 cm3), and the Exactech Equinoxe removed the least (3.3 cm3) despite having the largest baseplate. When each prosthesis was implanted along the inferior glenoid rim, the Equinoxe had the most cortical, cancellous, and overall glenoid bone surface contact (501.3 mm2), the RSP had the least cortical but second most cancellous and second most overall (386.0 mm2), and the Delta III had the second most cortical but least cancellous and least overall (360.6 mm2). Using larger baseplates was also advantageous in a 10-mm medially worn glenoid, where the Equinoxe had the most surface contact (383.1 mm2), the RSP was the second (296.9 mm2), and the Delta III had the least (274.1 mm2) [26].
While preserving glenoid bone, achieving optimal screw length/placement, and maximizing baseplate surface contact are all important contributors to fixation, these are but a few of the variables that establish initial fixation. Exactech has worked with the American Society for Testing and Materials (ASTM) since 2010 to develop a testing standard to objectively evaluate and quantify the fixation of RTSA prostheses before and after a clinically relevant cyclic loading pattern. This RTSA glenoid loosening test standard was approved in November 2013 and will be made effective in 2014 as a part of ASTM F 2028-14 [27]. The ASTM RTSA glenoid loosening test has previously demonstrated differences in fixation between screw configurations [28], medialized/lateralized CoR [29–31], glenoid baseplate designs [30–32], scapular defects and wear patterns [10, 33], and different densities of substrates [28, 31, 32]. Two recent studies quantified the fixation of 6 different commercially available RTSA prostheses in both low- and high-density polyurethane blocks [31, 32]. These standardized studies objectively demonstrated that the Equinoxe (both standard and expanded) and Delta III devices had significantly better glenoid fixation than each of the Zimmer, DJO, and BIO-RSA devices. Additionally, catastrophic failure was observed in at least 1 of each of the Zimmer, DJO, and BIO-RSA test components during cyclic loading; no failure occurred in either of the Equinoxe or Delta III devices [31, 32]. While designs with a more lateralized CoR generally performed worse, this was not the only factor impacting fixation. The Exactech performed significantly better than the Zimmer device, despite each having identical 2-mm lateralized CoRs. Thus, subtle differences in baseplate design can significantly impact fixation.
Aseptic glenoid loosening is more likely in eroded scapular morphologies. Generally, surgeons eccentrically ream an eroded glenoid to correct the defect. Unfortunately, eccentric reaming medializes the joint line and removes non-worn glenoid bone to correct the defect, which may compromise fixation [33, 34]. To conserve glenoid bone, increase prosthesis surface contact area with cortical bone, and to better restore the native joint line when performing RTSA in eroded scapular morphologies [26, 33, 35], Exactech is the first and only provider of augmented glenoid baseplates (Fig. 37.3). These augmented implants, released in early 2011, are implanted with off-axis reaming rather than eccentric reaming [33, 34] (Fig. 37.4). When using RTSA in glenoids with superior wear, superior augment baseplates are recommended; for glenoids with posterior wear, posterior augment baseplates are recommended; and for glenoids with superior–posterior wear, superior–posterior augment baseplates are recommended. For medial wear, a +10-mm extended cage baseplate is recommended to facilitate grafting of severely medially eroded glenoids and a +4-mm laterally offset glenosphere is recommended for less severely medially eroded glenoids.
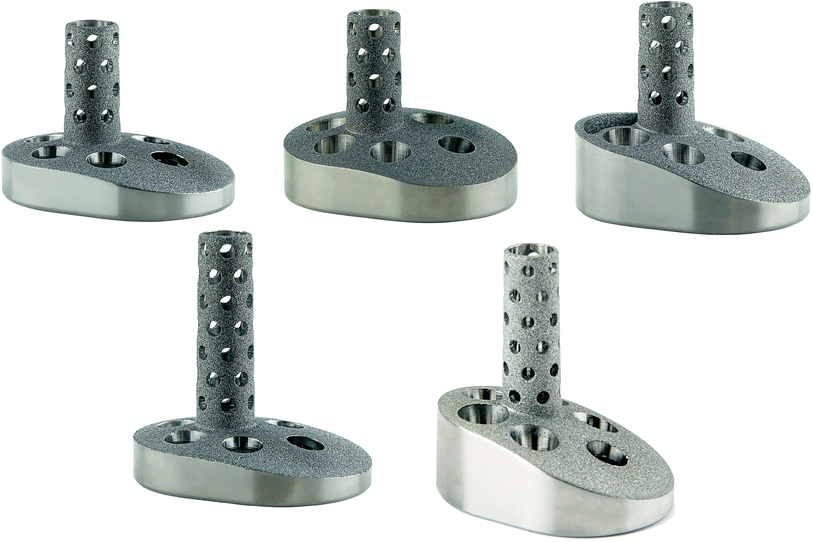
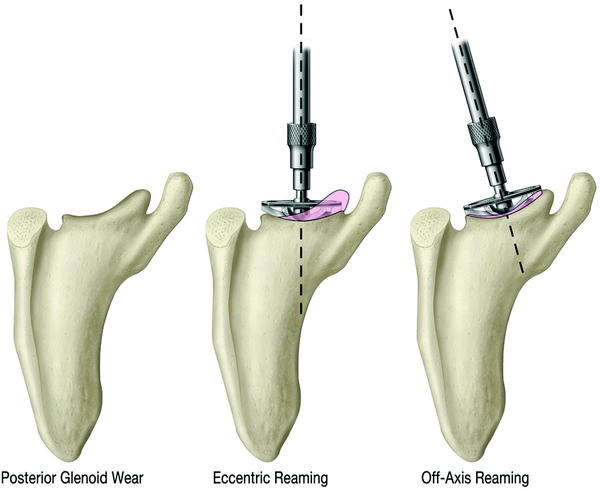
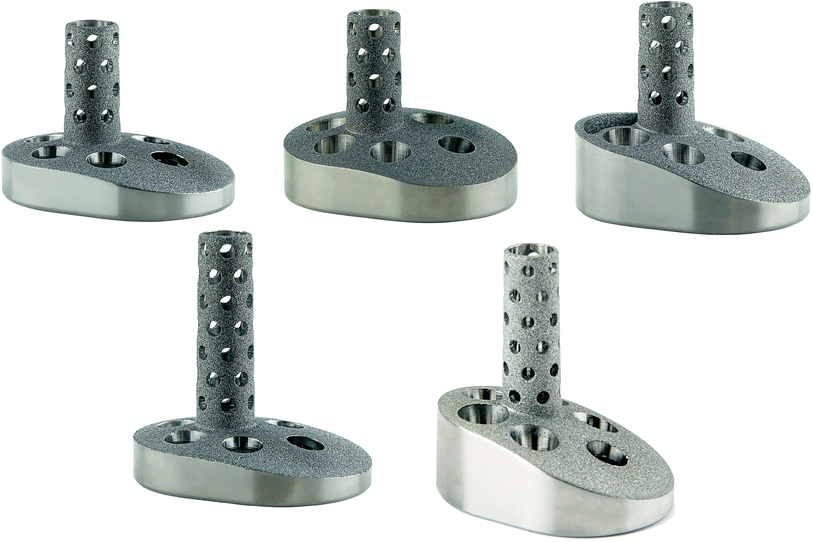
Fig. 37.3
The Equinoxe RTSA baseplates from left to right (top row): standard, 8° posterior, 10° superior, (bottom row) +10mm extended cage peg, and 10° superior/8° posterior augment baseplates
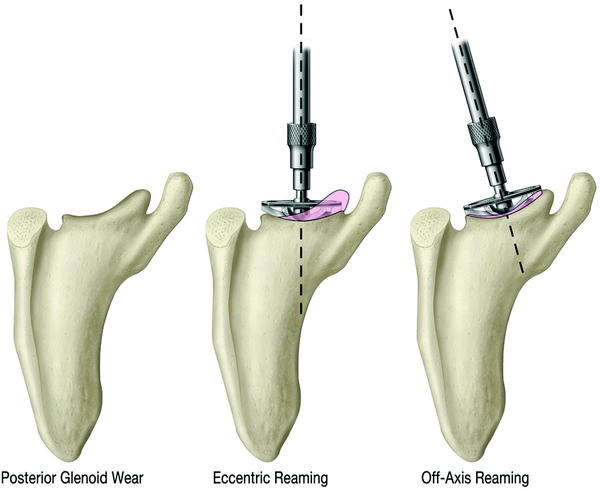
Fig. 37.4
Comparison of eccentric and off-axis glenoid reaming techniques with posterior glenoid wear; pink shading denotes bone removed with each technique. Note all augmented glenoids are implanted with off-axis reaming to conserve glenoid bone
Design Goal #3: Reduce the Instability Rate and Improve Restoration of Active Rotation
By minimizing humeral liner impingement with the scapula, the lever-out mechanism can be eliminated and the instability rate can be potentially reduced. Restoring the lateral position of the humerus may reduce the instability rate and also improve active internal/external rotation [2, 11, 22, 35–43]. The Grammont reverse shoulder medializes the humerus to such a degree that the rotator cuff is under-tensioned and deltoid wrapping around the greater tuberosity is reduced [35, 37–43]. By lateralizing the greater tuberosity to a more anatomic location, the Equinoxe can better restore rotator cuff muscle tension (Fig. 37.5) and deltoid wrapping (Fig. 37.6) [35, 37–44].
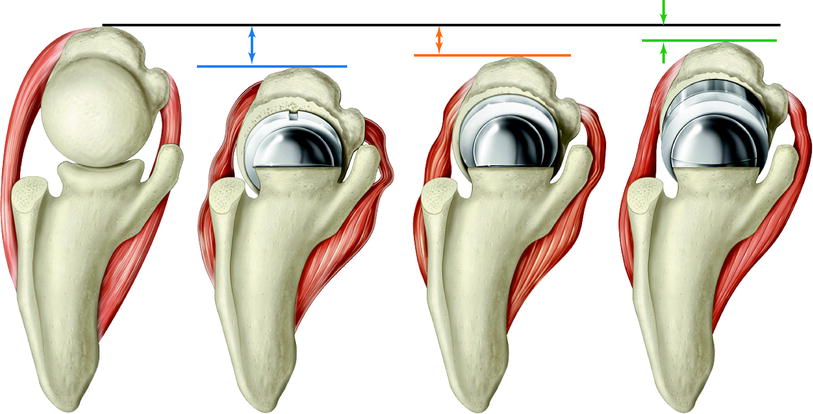
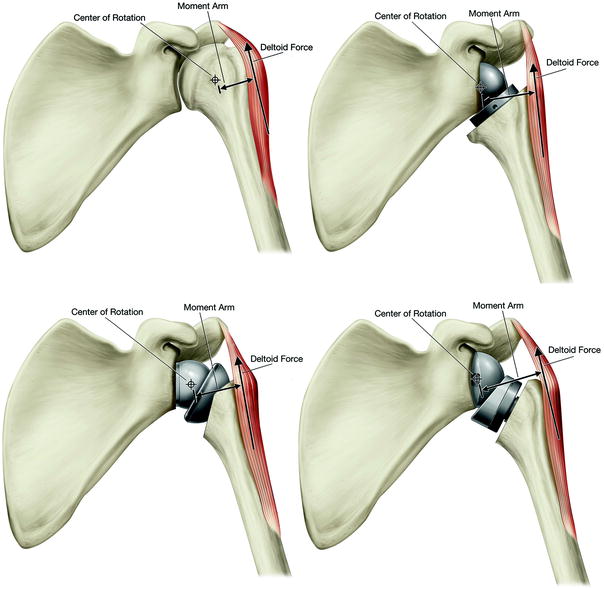
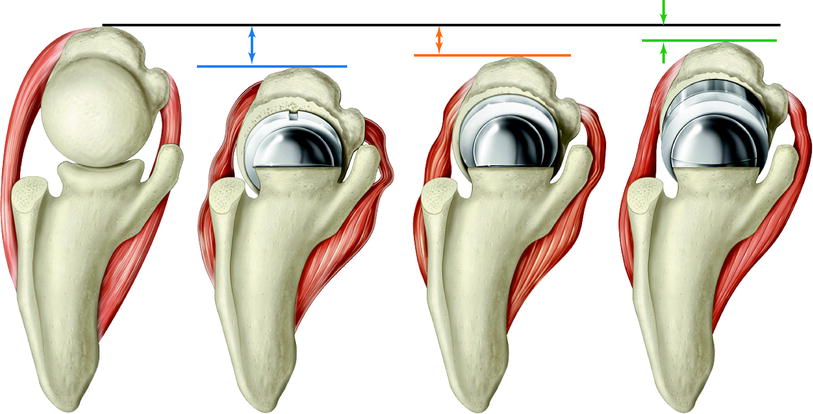
Fig. 37.5
Impact of humeral medial/lateral positioning on rotator cuff tensioning with RTSA; from left to right: anatomic shoulder, Grammont, DJO RSP, Exactech Equinoxe reverse shoulders
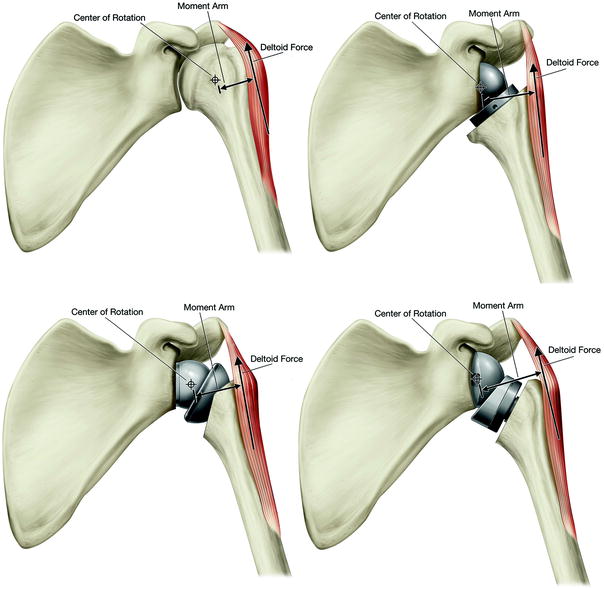
Fig. 37.6
Impact of position of the center of rotation and humerus on deltoid abductor moment arm and deltoid wrapping; from left to right (top row): anatomic shoulder, Grammont, (bottom row) DJO RSP, and Exactech Equinoxe reverse shoulders
To investigate the impact of humeral position with RTSA on muscle length, muscle moment arms, and deltoid wrapping, the aforementioned computer impingement model was adapted into a virtual shoulder model by adding 9 different muscles [34, 35, 37–40, 42–44]. Muscle lengths were calculated during simulation of abduction and internal/external rotation as the average length of the 3 lines simulating muscle at each position during the ROM relative to the anatomic shoulder. Moment arms were calculated from the muscle lines at each position relative to the CoR during each simulated motion. The angle of abduction in which the middle deltoid stops wrapping around the greater tuberosity was also measured.
The virtual shoulder model has previously quantified muscle lengths [34, 35, 37, 42–44], moment arms [37–40, 44], and deltoid wrapping of different RTSA prosthesis designs [35, 40, 42–44], implanted using a variety of implantation techniques [42] [glenosphere tilt, humeral retroversion, bony increased-offset RSA (BIO-RSA)] and in a variety of different glenoid morphologies (medial wear and posterior wear) [34, 35]. One recent study compared the muscle lengths and deltoid wrapping associated with the DePuy Delta III, DJO RSP, and Exactech Equinoxe designs along with the BIO-RSA technique. These results objectively demonstrated that designs and surgical techniques that resulted in more lateral humeral positioning were associated with more deltoid wrapping and better tensioning of the rotator cuff [42]. The Delta III positioned the humerus most medially and shortened the rotator cuff by as much as 45.3 %. We theorize that this magnitude of muscle shortening may be the primary mechanism for the limited improvements in active internal and external rotation reported with that prosthesis. The Equinoxe had the most lateral humeral position, most deltoid wrapping, and best restored the anatomic tension of the subscapularis, infraspinatus, teres major, and teres minor relative to the other RTSA prostheses evaluated [42].
These observations related to more anatomic rotator cuff tensioning and deltoid wrapping are likely responsible for the favorable ROM and clinical outcomes reported with the Equinoxe in a recent multicenter study [45]. Flurin et al. [45] reported on the clinical outcomes of 200 patients who received either an Equinoxe anatomic shoulder (n = 73) or reverse shoulder (n = 127) for osteoarthritis or cuff tear arthropathy, respectively. No instances of instability or glenoid loosening were reported in either cohort, and one instance of infection occurred in the RTSA cohort. Comparing results of ATSA and RTSA demonstrated no statistical difference between the postoperative Constant scores (ATSA: 76.5 ± 11.6; RTSA: 74.2 ± 8.6; p = 0.1237); whereas, ATSA patients were observed to have statistically higher postoperative ASES scores (ATSA: 90.3 ± 14.6; RTSA: 86.7 ± 10.1; p = 0.0451), active forward flexion (ATSA: 157.3 ± 18.2°; RTSA: 149.3 ± 16.9°; p = 0.0019), and active external rotation (ATSA: 37.3 ± 16.7°; RTSA: 32.6 ± 13.6°; p = 0.0319) than RTSA patients at similar postoperative follow-up (ATSA: 32.5 ± 12.1 months; RTSA: 30.8 ± 8.0 months). Despite differences with ATSA, these RTSA results [45] compare favorably with that previously reported [2, 4, 6, 9, 12, 36, 46] for other RTSA prosthesis designs.
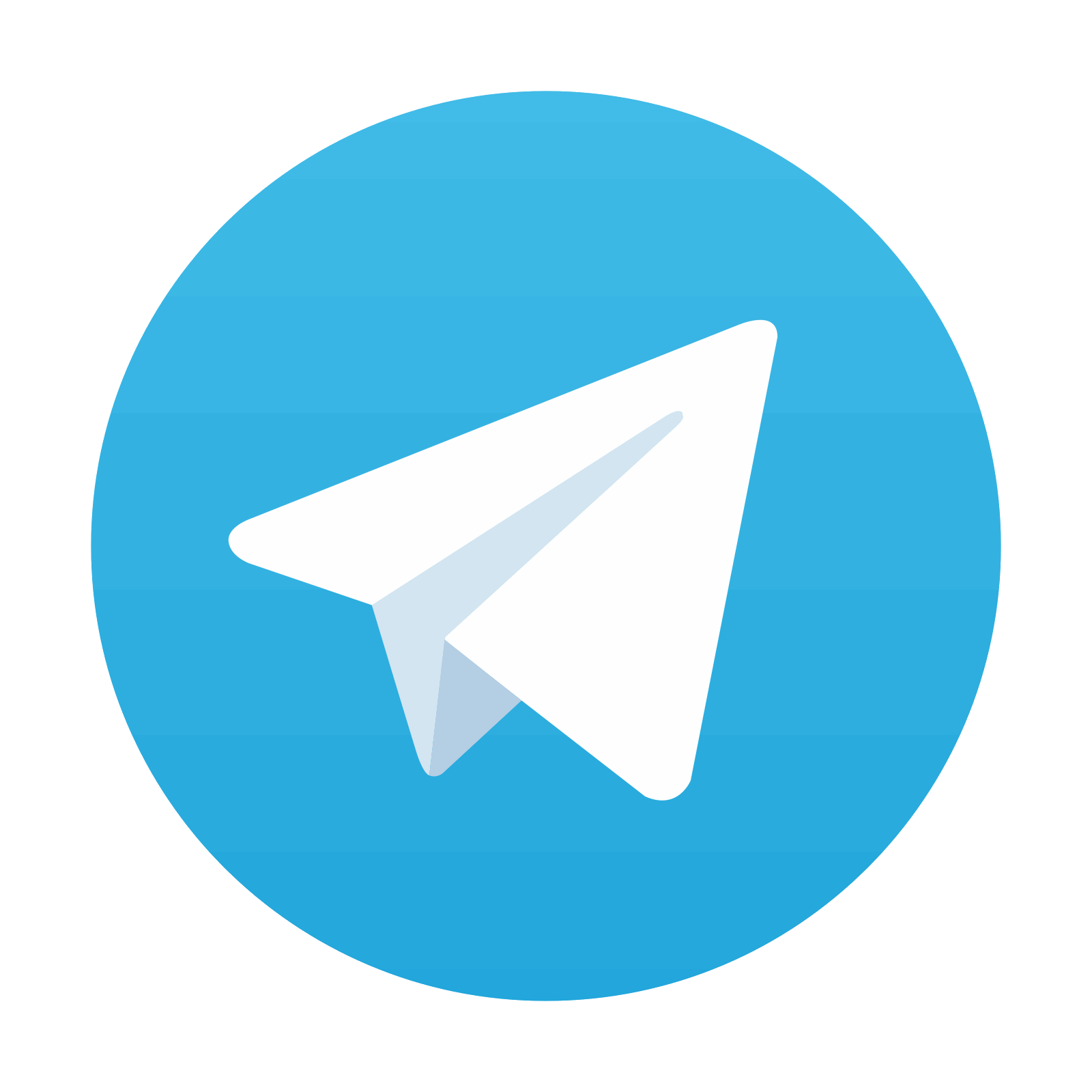
Stay updated, free articles. Join our Telegram channel

Full access? Get Clinical Tree
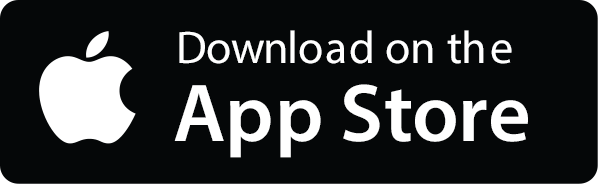
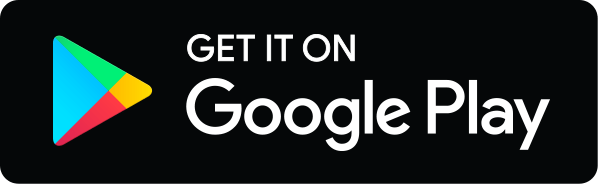