Chapter 35 Endocrinology
Pituitary Disorders
Hypothalamic-Pituitary Axis
The hormonal secretions of the anterior pituitary (the adenohypophysis) are regulated by a number of hypothalamic releasing hormones and inhibitory molecules. These factors reach the pituitary through the portal circulation and, on interaction with specific receptors, either stimulate or inhibit the secretion of the anterior pituitary hormones. The main releasing hormones include thyrotropin-releasing hormone (TRH), gonadotropin-releasing hormone (GnRH), corticotropin-releasing hormone (CRH), and growth hormone–releasing hormone (GHRH). The two major inhibitory factors are dopamine, which principally inhibits prolactin release, and somatostatin, a potent inhibitor of growth hormone (GH), and to a lesser extent, thyrotropin (TSH). Other factors have an important regulatory effect on anterior pituitary function. The kisspeptin hormones are a family of peptides encoded by the KiSS-1 gene and are thought to play a critical role in reproduction. Kisspeptin receptors stimulate GnRH release and activation of the mammalian reproductive axis. Mutations in kisspeptin receptor GPR-54 cause idiopathic hypogonadotropic hypogonadism, characterized by delayed or absent puberty (Jayasena and Dhillo, 2009).
Approach to Pituitary Disease
Hypopituitarism
Causes
Hypopituitarism may result from a genetic disorder or deficiency in hypothalamic releasing factor but more often is the result of pituitary tissue destruction secondary to mass expansion, infiltrative process, autoimmune or infectious disease, vascular accidents, radiation injury, or trauma. In some patients the etiology of hypopituitarism cannot be identified and is considered idiopathic. Most idiopathic cases are sporadic, although there are well-described familial causes of hypopituitarism.
Clinical Manifestations
Failure to lactate may be the first clinical sign of Sheehan’s syndrome (postpartum pituitary necrosis). Lethargy, anorexia, and weight loss; failure to resume normal menstrual periods; and loss of sexual hair may also be present later in the postpartum period. On the other hand, symptoms and signs of pituitary infarction may be subtle and not recognized for years.
Diagnosis
Pituitary hormone secretion is episodic, and in general, dynamic testing is more valuable than single, baseline hormone measurements. For practical reasons, however, the screening can be done with pituitary hormone and target hormone measurements simultaneously. For evaluating suspected hypopituitarism, tests include thyroid function, LH, serum testosterone in men and estradiol in women, IGF-1 (because GH has a short half-life in blood), prolactin, and morning cortisol. Provocative testing for GH and ACTH reserve may be required as well. Patients with known pituitary disease and deficiency of ACTH, TSH, or gonadotropins have a 95% chance of a subnormal provocative stimulus for GH. Also, patients with known pituitary disease and a serum IGF-1 concentration lower than normal can be presumed to have GH deficiency (Gharib et al., 2003). Provocative tests for GH are either physiologic (sleep or exercise) or pharmacologic such as insulin-induced hypoglycemia, GHRH with arginine, and levodopa with arginine tests (Biller et al., 2002). GH deficiency is diagnosed when GH does not rise above 5 ng/mL in response to two or more stimuli.
Treatment
Treatment of secondary hypogonadism depends on the patient’s gender and whether or not fertility is desired. Estradiol and progesterone replacement is the treatment of choice for secondary hypogonadism in premenopausal women who have an intact uterus and do not desire fertility. These hormones can be given cyclically or daily in fixed-dose combinations. In women with hysterectomy, estrogen replacement is sufficient, to maintain vulvar and vaginal lubrication, relieve symptoms of vasomotor instability, and reduce bone loss. Women wanting to restore fertility should be referred to specialized centers for pharmacologic induction of ovulation with exogenous pulsatile GnRH and exogenous FSH and LH treatment. GnRH can be used to restore fertility when hypothalamic disease and tertiary hypogonadism are present. Women over age 50 with secondary hypogonadism should be treated as menopausal, taking into consideration the risk/benefit ratio of estrogen replacement therapy in this age group.
Hyperfunctioning Pituitary Adenomas
Hyperprolactinemia and Prolactinomas
Diagnosis
Hypersecretion of prolactin may be physiologic or pathologic in origin. Physiologic stimulators include exercise, pain, breast stimulation, sexual intercourse, general anesthesia, and pregnancy. Pathologic causes of hyperprolactinemia include prolactinomas, decreased dopaminergic inhibition of prolactin secretion through pharmacologic agents, and decreased clearance of prolactin. Early manifestation of prolactin hypersecretion is galactorrhea and menstrual irregularities, notably amenorrhea, in women and erectile dysfunction or loss of libido in men. Rarely, galactorrhea with gynecomastia can occur in men. These patients are at risk of developing osteoporosis secondary to hypogonadism as well as a result of the direct inhibitory effect of prolactin on bone formation. Galactorrhea is rarely found in postmenopausal women with hyperprolactinemia, in whom mass effect of prolactinomas may cause the principal presenting symptom, such as headache or visual disturbance (Mancini et al., 2008). Similarly, the diagnosis of prolactinomas in men is often delayed because the clinical signs and symptoms of hyperprolactinemia are less obvious.
Cushing’s Disease
Hypercortisolemia (also hypercortisolism, hyperadrenocorticism), caused by either exogenous administration of cortisol or other synthetic glucocorticoids or endogenous overproduction of cortisol, leads to a constellation of clinical and biochemical findings referred to as Cushing’s syndrome (Arnaldi et al., 2003; Findling and Raff, 2005). The multiple causes include pituitary adenomas, excess production of CRH leading to hyperplasia of corticotropes in the pituitary, ectopic production of ACTH and CRH, and adrenocortical adenomas and carcinomas. The term Cushing’s disease specifically refers to pituitary-dependent cortisol hypersecretion (Biller et al., 2008). Pituitary, ACTH-dependent Cushing’s disease accounts for at least 70% of endogenous cases, while the most common cause of ACTH-independent Cushing’s syndrome is prolonged glucocorticoid therapy.
Craniopharyngiomas; Thyrotropin-Secreting Pituitary Adenomas; Gonadotropic and Other Adenomas
See the discussions of these pituitary tumors online at www.expertconsult.com.
Posterior Pituitary Disorders
Antidiuretic hormone (vasopressin) is synthesized in the hypothalamus and migrates down into the posterior lobe of the pituitary to be stored and later secreted. Some ADH is secreted directly into the cerebrospinal fluid (CSF) rather than the posterior pituitary. Thus, pathologic lesions affecting the hypothalamus below the median eminence may preserve some functional ADH that migrates from the CSF into the systemic circulation. The half-life of AVP in circulation is only 20 minutes because of its susceptibility to peptidases. Loss of the terminal amino group in position 1 makes this peptide resistant to degradation, whereas substitution of the levo analog of arginine for dextroarginine in position 8 reduces presser effect without altering its antidiuretic properties. The resultant peptide deamino-8-d-argenine vasopressin (DDAVP) is currently the treatment of choice for central diabetes insipidus.
Central Diabetes Insipidus
Treatment
Patients with partial DI will benefit from oral agents that potentiate AVP action or stimulate the release of AVP. These agents include chlorpropamide, carbamazepine, and clofibrate. In such cases, desmopressin requirements may be lower than available preparations can provide. Patients with nephrogenic DI benefit from thiazide diuretics or indomethacin. Patients with DI should wear a medical alert bracelet. When the ability to drink fluids is impaired, intravenous hydration will be required to avoid dehydration and hypernatremia.
Syndrome of Inappropriate Secretion of Antidiuretic Hormone
Syndrome of inappropriate secretion of antidiuretic hormone (SIADH) is associated with plasma ADH concentrations that are inappropriately high for the plasma osmolality. Laboratory and clinical features of SIADH include (1) euvolemic hyponatremia; (2) decreased measured plasma osmolality (<275 mOsm/kg); (3) urine osmolality >100 mOsm/kg; (4) urine sodium usually >40 mEq/L; (5) normal acid-base and potassium balance; (6) blood urea nitrogen (BUN) <10 mg/dL; (7) hypouricemia <4 mg/dL; (8) normal thyroid and adrenal function; and (9) absence of advanced cardiac, renal, or liver disease (Reddy and Mooradian, 2009). Conditions or factors associated with SIADH include CNS trauma and infections, tumors, drugs, major surgery, pulmonary disease (e.g., TB), hormone administration, human immunodeficiency virus (HIV) infection, hereditary SIADH, idiopathic causes, and cerebral salt wasting (Box 35-1).
Box 35-1 Select Causes of Syndrome of Inappropriate Antidiuretic Hormone Secretion (SIADH)
Modified from Reddy P, Mooradian AD. Diagnosis and management of hyponatremia in hospitalized patients. Int J Clin Pract 2009;63:1494-1508.TB, Tuberculosis; SSRIs, selective serotonin reuptake inhibitors; TCAs, tricyclic antidepressants; MAOIs, monoamine oxidase inhibitors; ACE, angiotensin-converting enzyme; MDMA, 3,4-methylenedioxymethamphetamine.
In some cases it is difficult to differentiate SIADH from mild to moderate depletional hyponatremia. The response of urinary and plasma sodium concentration to an infusion of 1 to 2 L of 0.9% (isotonic) saline may help in the differential diagnosis. In the patient with SIADH who is at equilibrium, the saline will be excreted, and therefore urinary sodium will increase while plasma sodium concentration will either not change or decrease slightly. If the patient has depletional hyponatremia from renal losses, sodium from the administered saline is retained and the excess water excreted. Urinary sodium decreases, whereas plasma sodium concentration increases.
Treatment
KEY TREATMENT
Thyroid Disorders
Anatomy and Physiology
Circulating Thyroid Hormones
Thyroid hormones exert their effect by binding to thyroid receptors (TRs) within cells. At the cellular level, T3 is about twice as biologically active as T4, partly because T3 binds to TRs 10 to 15 times more than T4 (Yen, 2005). T3 is the biologically active form of thyroid hormone. Thyroxine’s role in this process appears to be that of a prohormone, providing a readily accessible reservoir for conversion to T3; otherwise, its exact purpose is unknown (Bianco and Larsen, 2005).
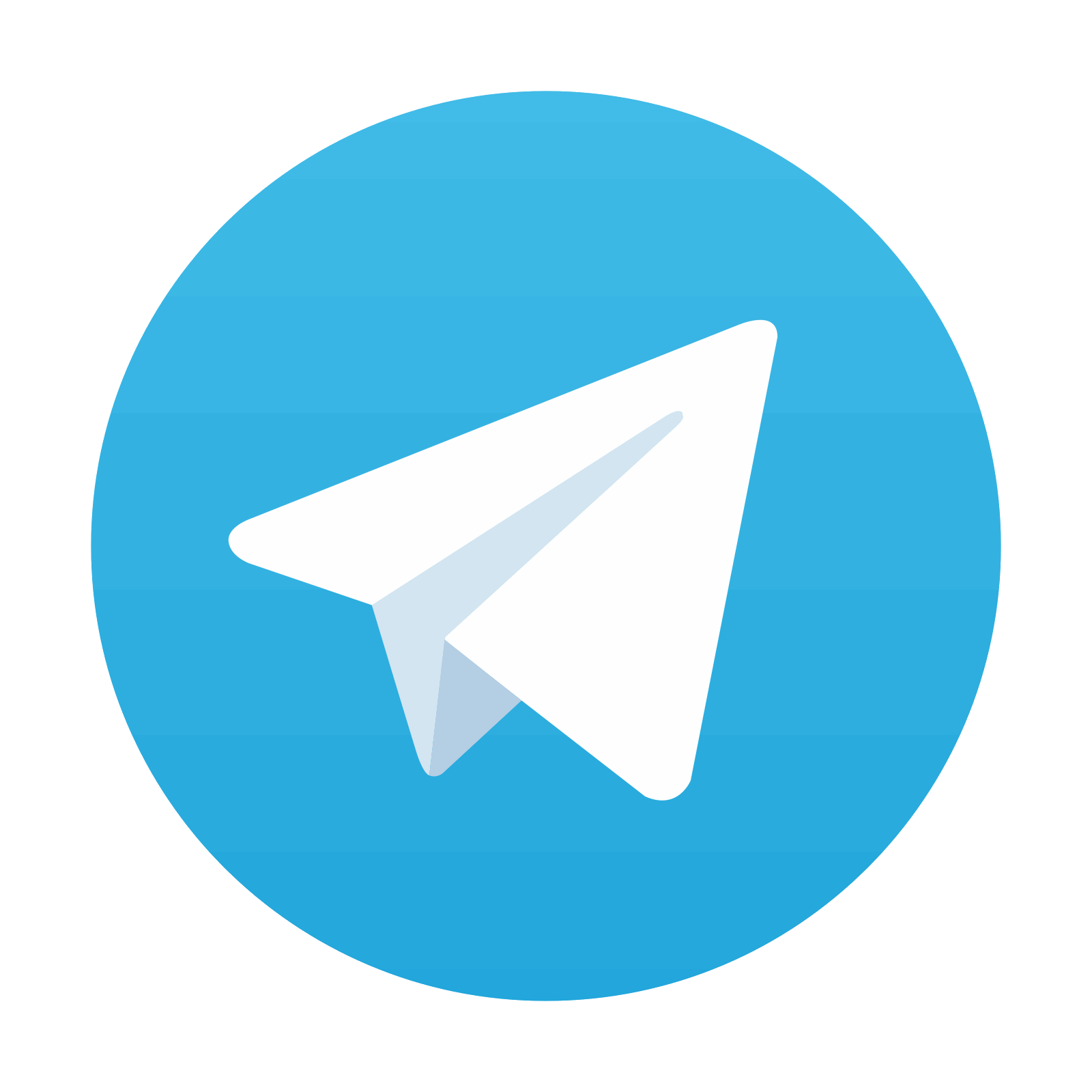
Stay updated, free articles. Join our Telegram channel

Full access? Get Clinical Tree
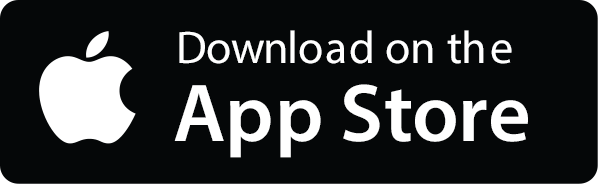
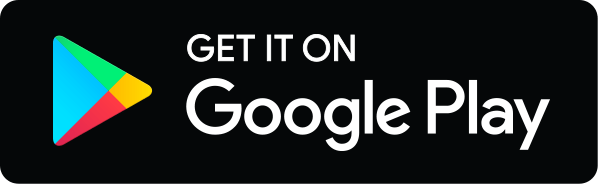