38 Key Points 1. MEPS and SSEPs are predictive of hand and ambulatory capacity. MEP amplitudes and latencies do not appear to correlate with recovery in longitudinal studies 2. The sympathetic skin response can indirectly assess supraspinal pathways and reflects the integrity of the autonomic pathways. SSRs are absent below the level of the spinal lesion and should be used to identify populations at risk for autonomic hyperreflexia (AH). 3. The electrical perceptual test may be a better test to assess sensory physiology and recovery after SCI. Used in conjunction with dSSEPs, the two tests provide better results than traditional SSEP tests. There is an increasing interest in the use of clinical electrophysiological assessments in the evaluation of severity and outcomes after spinal cord injury (SCI). Electrophysiological measures offer several advantages over qualitative clinical measures. First, electrophysiological recordings provide quantitative, objective data that can be analyzed by blinded researchers.1 This is particularly relevant in designing appropriate clinical trials that aim to compare functional outcome measures. For example, the reliability of measurements between assessments and between investigators is increased relative to traditional clinical tests, which are dependent on human evaluation. Second, the measures are more flexible and environmentally independent, thus allowing researchers to perform recordings on unresponsive, uncooperative, or comatose patients.2 The greater feasibility and flexibility that electrophysiological measures provide give clinicians and investigators freedom to assess previously unexaminable patients. Third, measures of evoked potentials complement existing SCI recovery assessments, such as the American Spinal Injury Association (ASIA) sensory and motor scores, because they are able to assess specific parts of the spinal segments and peripheral nerve tracts and autonomic nervous system. In particular, measures can target specific spinal segments below the level of injury.3 Finally, combinations of recording techniques can provide detailed quantitative information about a patient’s condition that cannot be determined through other clinical means. There is much promise in using these measures to assess SCI, predict functional outcomes, and inform clinicians about the planning and results of therapeutic interventions. This chapter provides an overview of the most common electrophysiological tests: motor evoked potential (MEP), somatosensory evoked potential (SSEP or SEP), dermatomal somatosensory evoked potential (dSSEP), electromyography (EMG), nerve conduction studies and F waves, sympathetic skin response (SSR), H-reflex recordings, and electrical perceptual threshold (EPT) recordings. A summary of these techniques is provided in Tables 38.1 and 38.2. MEPs are produced by transcranial magnetic stimulation (TMS). Developed in 1985,4 TMS generates a magnetic field, which, in turn, induces an electrical current that elicits descending corticospinal volleys, which are recorded as MEPs using surface electrodes on muscles of interest. Measurable MEP parameters (Table 38.1) include the stimulation intensity that elicits MEPs (MEP threshold), amplitude and latency of MEPs, and the slope of plots of amplitude against intensity (input–output or recruitment curve)5 (Fig. 38.1). These parameters provide information about the state of cortical excitability after injury. The slope of the steep portion of the curve increases with increasing cortical excitability, such as following treadmill training (Fig. 38.2).6 Additional information about sensorimotor physiology can be obtained by performing paired pulse TMS studies. In paired pulse studies, a conditioning subthreshold or suprathreshold stimulus is followed by a second test pulse to determine the effect of the conditioning pulse on the size of MEP response generated by the test pulse alone. At interstimulus intervals between 1 and 5 msec, subthreshold conditioning pulses inhibit suprathreshold test pulses and are referred to as short-interval intracortical inhibition (SICI). Intracortical facilitation (ICF) is at interstimulus intervals between 10 and 25 msec. By varying parameters other types of inhibition have been described. Suprathreshold conditioning pulses paired with suprathreshold test pulses at interstimulus intervals of 50 to 200 msec cause long-interval intracortical inhibition (LICI). Conditioning median nerve stimulation inhibits test MEP responses at ISIs between 20 and 30 msec or at ISIs between 100 and 200 msec. These are referred to as short-latency afferent inhibition (SAI) and long-latency afferent inhibition (LAI), respectively (Fig. 38.3). The central conduction time (CCT) measures the conduction time between the cortex and motor neuron pool. The silent period refers to a period of EMG silence following the MEP when TMS is performed during a sustained muscle contraction.
Electrophysiological Measures after Spinal Cord Injury
Motor Evoked Potentials
TMS parameter | Function | Main findings in SCI |
MEP amplitude | Measure of cortical excitability and corticospinal tract integrity | ↓↓ |
MEP threshold | Measure of cortical excitability | ↑↑ |
MEP latency | Measure of total conduction time | ↑↑ |
CCT | Measure of conduction time to motor neurons | ↑↑ |
Cortical silent period | Measure of cortical and spinal inhibition | ↑↑ |
SAI | Measure of sensorimotor integration | Not studied |
SICI | Measure of intracortical inhibition | Not studied |
Slope of input–output curve | Measure of cortical excitability and corticospinal tract integrity | Not studied |
MEP facilitation |
| ↓↓ |
Abbreviations: CCT, central conduction time; MEP, motor evoked potential; SAI, short-latency afferent inhibition; SCI, spinal cord injury; SICI, short-interval intracortical inhibition; TMS, transcranial magnetic stimulation.
Table 38.2 A Summary of Electrophysiological Tests for Assessment of Spinal Cord Injury
Test | Key features | Key findings and potential use in SCI |
Motor evoked potentials (MEPs) | Assess descending corticospinal tract function via signals induced by transcranial magnetic stimulation (TMS) of the motor cortex | MEP latencies remain unchanged over time, suggesting that recovery in SCI patients is not related to improvements in spinal conductivity Correlated to recovery of hand and ambulatory capacity Can be used to predict level of injury Absence is indicative of poorer prognosis Increase in amplitude may correlate to functional recovery |
Somatosensory evoked potentials (SSEPs) | Assess ascending spinal tract function via stimulation of peripheral nerves Record response in the somatosensory cortex using an electrode on the subject’s scalp | Signal amplitudes have been shown to correlate with functional outcomes SSEPs can be used to determine injury level in unexaminable patients |
Dermatomal somatosensory evoked potentials (dSSEPs) | Similar to SSEPs but are obtained by cutaneous electrical stimulation of dermatomal points Appear to correlate with EPT results | When paired with electrical perceptual threshold tests and electrical pain perception, dSSEPs reliably measure segmental integrity |
Electromyography (EMG) and F-waves and compound muscle action potentials | Assess peripheral nerve injury and anterior horn damage; F-waves are elicited by supramaximal stimulation of peripheral nerves and test the integrity of the efferent peripheral motor pathway and may test excitability of the motor neuron pool | Analysis of surface EMG patterns may be able to identify patients with discomplete injuries |
Sympathetic skin response (SSR) | Assesses spinal cord autonomic integrity using electric or magnetic stimulation from above the spinal lesion Records response on the palmar, plantar skin surface or perineum using surface electromyographic (EMG) electrodes | SSR is absent below the level of complete lesion Loss of palmar SSR was associated with development of AH in 93% of patients Useful in assessing injury level and injury completeness |
Hoffmann reflex (H-reflex) | H-reflex is a measure of spinal cord excitability elicited by submaximal stimulation of a mixed peripheral nerve and caused by activation of a motor neuron Records response with surface EMG electrodes | Amplitude of H-reflex increases over time post-SCI The H-reflex test may be useful as a marker of spinal cord excitability and plasticity |
Electrical perceptual thresholds (EPTs) | Assess the level and degree of sensory impairment of SCI by cutaneous electrical stimulation of American Spinal Injury Association (ASIA) sensory points Record subject’s perceived response at minimal ascending and descending stimulation intensities | Provide a more objective test of sensory function that is as accurate as clinical assessment according to ASIA classifications |
Fig. 38.1 Input–output recruitment curve of the resting tibialis anterior muscle. On the right are examples of motor evoked potentials at intensities of 1.2 and 1.5 times motor threshold.
Fig. 38.2 Representative recruitment curves from three different subjects before (—) and after (- – -) treadmill training. (Top) Recruitment curves from the tibialis anterior (TA) and vastus lateralis (VL) muscles (middle; right). In all three examples, the recruitment curves after training rested above the recruitment curves before training, especially at intermediate- and high-stimulation intensities. The slope of the steep portion of the sigmoid line fit to the recruitment curve also increased after training. (Bottom) The mean background electromyography (EMG) results after training (• • •) were similar to the background EMG results before training (—). Data points represent trial means ± standard deviation. (From Thomas SL, Gorassini MA. Increases in corticospinal tract function by treadmill training after incomplete spinal cord injury. J Neurophysiol 2005;94:2844–2855. Reprinted with permission.)
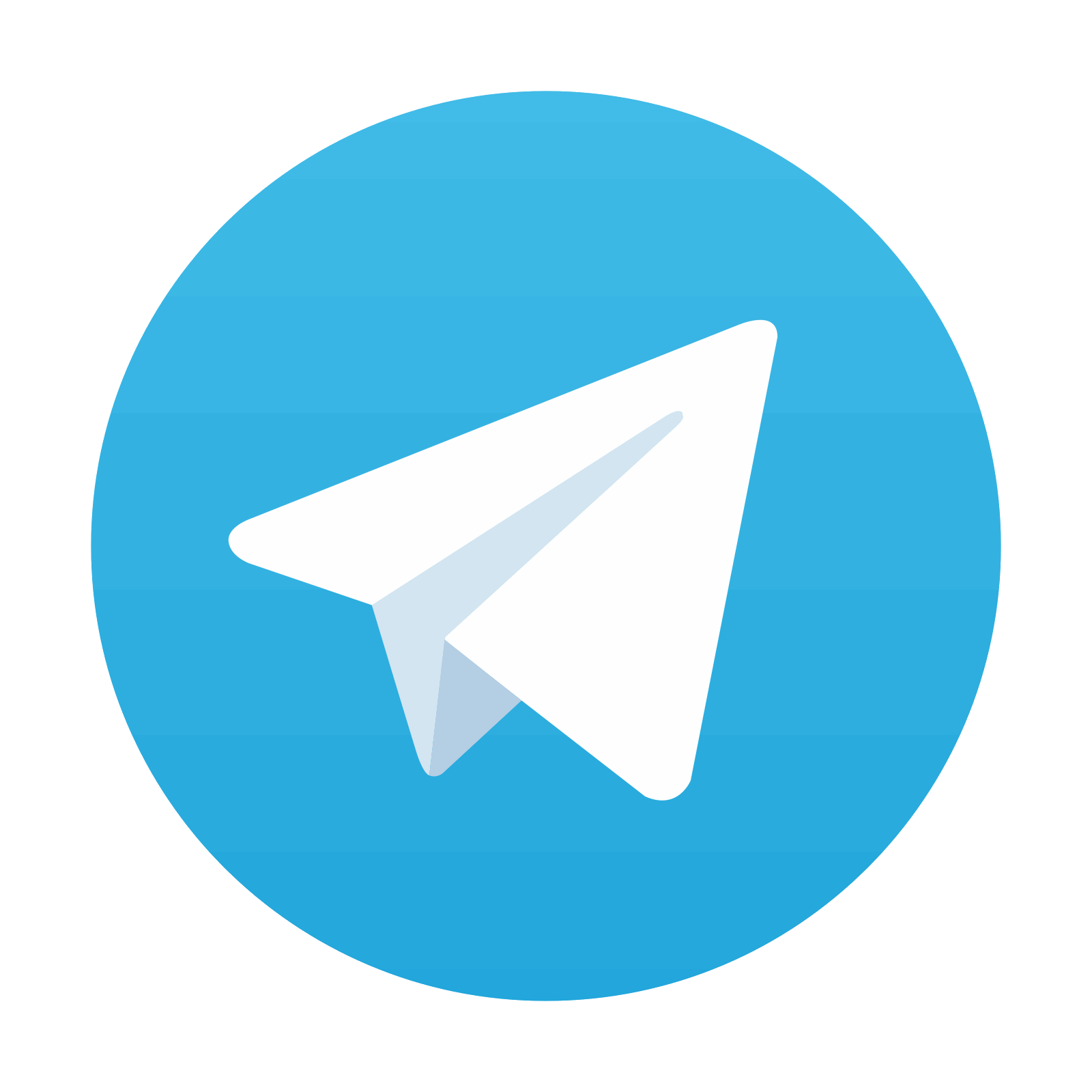
Stay updated, free articles. Join our Telegram channel

Full access? Get Clinical Tree
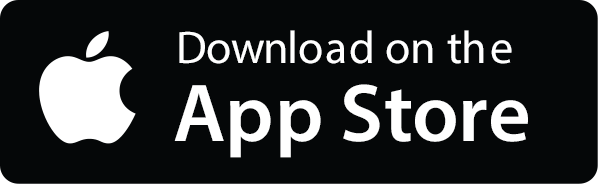
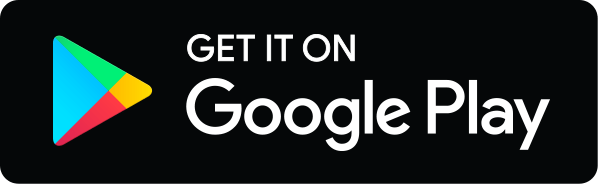