CHAPTER 1 Development of the Spine
The embryologic development of the human spine is an enormously complex process that is only partially understood. Differentiation of the pluripotent tissues of the embryo leads to early formation of a repetitive segmented vertebral structure. Because the embryo is exquisitely susceptible to malformation and developmental error, each step of formation is critical.1–4 Familiarity with these various steps can be helpful in understanding not only congenital syndromes, but also the possible developmental role concerning predisposition to some degenerative spinal processes, typically considered “wear and tear” conditions.5,6 The continuously expanding understanding of the genetic basis of life, with the genetics of spinal development no exception, has aided the understanding of these syndromes.7–13
Early Embryologic Spine Precursors: Day 17 to Week 4
The development of the human spine begins on the 17th day of gestation. This is within the triploblastic stage of the embryo, during which it is shaped as a disc (Figs. 1–1 and 1–2). On one side of the disc is the amnion cavity, and on the other is the yolk sac. On the dorsal layer (which is in contact with the amnion) of the disc, there are epiblastic cells that converge and invaginate into the disc to form the primitive pit or node. When embedded within the tissue, it forms a tubelike structure that extends craniad, “burrowing” deep to the embryonic disc along its ventral surface. The tube cavity is in continuity with the amniotic fluid. This extension is known as the notochordal tube.
The notochord lies ventral to the neural tube in the midline. Mesodermal tissues on either side of these structures condense to form longitudinal columns. By the 19th day, there are three distinct columns on either side of the midline: (1) medial paraxial columns, which give rise to the somites; (2) intermediate mesodermal columns, which form the urogenital organs; and (3) lateral mesodermal plates, which form the gut cavities. In considering the development of the spine, attention is focused on the medial paraxial columns. The juxtaposition to the intermediate columns may help explain, however, why abnormalities of the urogenital tract are frequently associated with vertebral anomalies.1
The somites are arranged in consecutive fashion along the dorsal aspect of the embryo. They are first formed in the rostral (or cranial) aspect of the embryo, continuing caudad to form 42 to 44 individual segments over a period of days where the medial paraxial columns previously existed. Because they are close to the dorsal surface, they are visibly apparent as a series of beaded elevations (Fig. 1–3).
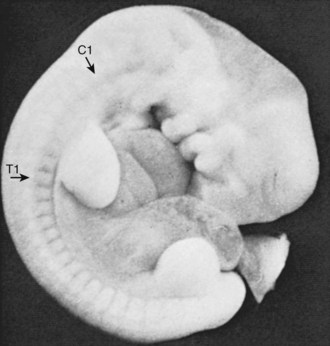
FIGURE 1–3 Somites of the human embryo are externally represented as a series of dorsolateral swellings.
Within the somite different regions have specialized fates (Figs. 1–4 and 1–5). The dorsolateral cells become the dermomyotomes. These eventually give rise to the skin (lateral) and muscle (medial) overlying the spine. The ventromedial cells within the somite become the sclerotomes. These are the precursors of the skeletal components (vertebrae) of the spine. The neural tube is fated to become the spinal cord.
From Somites to Spinal Column
Precartilaginous (Mesenchymal) Stage: Weeks 4 and 5
In metameric fashion, the sclerotomes are organized into a consecutively stacked arrangement. The next step in spinal development has been explained by the “resegmentation” theory.14–18 Resegmentation describes the division of each sclerotome into a cranial and caudal half. The cranial half is loosely arranged, whereas the caudal half is composed of densely packed cells. A small portion of the densely packed cells migrate superiorly to form the annular portion of the intervertebral disc, surrounding the notochord. Most of the densely packed cells fuse with the loosely packed cells of the adjacent caudal sclerotome. This fusion creates the centrum, the precursor of the vertebral body. The centrum develops from portions of two neighboring sclerotomes. This has significance on the anatomy of the fully developed spinal column. Initially, the segmental nerve precursors are located at the midportion of each sclerotome, whereas the segmental artery lies at the junction between two adjacent levels. After resegmentation, the nerve lies at the level of the disc and the artery lies at the mid-centrum, where one would expect to find them in the fully developed specimen.
Experimental data support the resegmentation theory.8,19–22 The crux of these experiments includes implanting a quail somite (from a quail embryo) within a chick embryo’s native somites. The quail somite is juxtaposed to a chick somite, and they develop together as the embryo grows. The quail tissue can be differentiated from the chick tissue using special staining techniques. Eventually, the somites give rise to sclerotomes that develop into centra. With the use of this model, it has been shown that the centrum does arise from the caudal and cranial halves of adjacent sclerotomes. The posterior arches (i.e., laminae) appear to follow this same pattern of growth.
This process seems to be highly influenced by the Pax1 and Pax9 genes.22 It is unclear whether the spinous process develops from one sclerotome or two adjacent levels.8,22 Other investigators have produced evidence of resegmentation using genetic labeling techniques.20 These studies involved injection of retroviral particles containing the lacZ transducing vector BAG into a single somite of a chick embryo. In other words, a single somite was genetically altered so that its cells would produce the lacZ gene product—the protein β-galactosidase. When the investigators evaluated the developed embryo, they detected β-galactosidase in the caudal and cranial halves of two adjacent vertebrae, suggesting that cells from the labeled somite were incorporated into two neighboring vertebrae.
Cartilaginous Stage: Weeks 6 and 7
Before the 6th week, the embryonic spinal precursor is composed of mesenchymal cells. Starting in the 6th week, cartilage-producing centers, or chondrification centers, form within each developing vertebra. Although type II collagen production within the extracellular matrix has been detected in the 5th week, it is most active during the cartilaginous stage; it tapers off during the ossification stage, but its production persists within the notochordal remnants of the nucleus pulposus.12 Two chondrification centers form in each half of the centrum, which eventually fuse into a solid block of cartilage. A hemivertebra is formed because of a failure of chondrification in one half of the vertebral body. The segmental arteries from either side of the centrum fuse at its middle aspect. Chondrification centers also form within each half of the vertebral arch and eventually fuse with each other in the midline and to the posterior aspect of the centrum.
Next, primitive cartilaginous transverse processes and spinous processes develop from the vertebral arch. More recent evidence has shown that the cartilaginous spinous process is formed from Msx1 and Msx2 (two embryologic proteins), producing mesenchymal cells, which require BMP4 to differentiate.23 These relationships highlight the important interactions of primordial proteins in governing further development of the spine.
Ossification Stage: Week 8 and Beyond
Primary ossification centers develop in utero. In the spine, ossification centers form within the cartilaginous template. There are three primary ossification centers in the typical embryonic vertebra: one in the center of the centrum and one in each of the vertebra arch halves. At about the 9th week, the preparation for ossification of the centrum is heralded by anterior and posterior excavations of the cartilaginous centrum produced by the invasion of pericostal vessels.24 These vessels produce ventral and dorsal vascular lacunae, which support the initial ossification (Fig. 1–6). Ossification of the centra starts first at the lower thoracic spine working craniad and caudad from that point.25
Secondary ossification centers develop after birth. In the spine, these appear after puberty. There are five centers: one in the tip of the spinous process, one in each transverse process tip, and one ring epiphysis in the superior and inferior endplates of the vertebral bodies. This development occurs at about 15 or 16 years of age, but eventually these ossification centers fuse in the middle of the 3rd decade (Fig. 1–7).26 The transverse processes of the lower cervical vertebrae, particularly C7, may show an additional costal center of ossification that produces the troublesome cervical rib; this reinforces the concept that all vertebrae primitively had the potential of forming ribs.
A pair of embryologic joints, known as neurocentral joints, is not present in the fully developed spine. These are located at the junction of the vertebral arches and the centrum but are anterior to the site of the future pedicle. Although not true “joints,” they allow expansion of the vertebral arch and spinal canal along with growth of the vertebral body. This expansion is most rapid between 18 and 36 weeks of gestation.2 At birth, the spinal canal diameter at L1 through L4 is approximately 70% of adult size, whereas at L5 it is only 50%.2 This indicates differential growth within regions of the vertebral column during fetal development. Full adult dimensions are reached by 1 year of life at L3 and L4 levels. The neurocentral joints persist until 3 to 6 years of age. The fusion of the fetal vertebral arches to the centra occurs well anterior to the pedicles, at the site of the neurocentral joints. The definitive vertebral body includes more than just the bone derived from the ossification center of the centrum, so the terms body and centrum are not accurately interchangeable (Figs. 1–8 and 1–9).
It is commonly thought that isthmic spondylolysis occurs because of a stress-type fracture within the pars interarticularis of the lower lumbar vertebrae, most commonly L5. Specific anatomic features of the adult lumbar spine, such as variation of the dimensions of the “lateral buttress” within the lumbar spine, have been described. Prenatal factors have been sought, but with limited success. Sagi and colleagues5 analyzed histomorphologically the lumbar spines of fetal spines aged 8 to 20 weeks to determine the sequence and location of ossification of the pars interarticularis of the various levels. They reported several findings: First, the pars begins to ossify in the 12th to 13th week of gestation. In the upper lumbar levels, ossification begins at the posterior portion of the pedicle and continues caudad, creating uniform ossification and trabeculation of the pars interarticularis. In contrast, the pars of the lower lumbar levels begins within the center of the pars itself, extending from this point to connect to the neighboring structures. Sagi and colleagues5 found that this resulted in uneven ossification. This finding may help explain areas of weakness within the pars interarticularis of the lower lumbar levels and may suggest that there is a prenatal predilection for a stress fracture in most individuals.
Fate of the Notochord
In the early embryo, the notochord serves as a rigid template around which the future vertebral column develops. It is a uniform structure that is present throughout the entire length of the primordial spinal column. A sheath exists around the notochord in its early stages. Immunohistochemical staining studies of 4- and 5-week embryos identified that a complex of extracellular matrix molecules is already present within this sheath, including sulfated glycosaminoglycans, hyaluronic acid, fibronectin, laminin, tenascin, and collagen II.27 Aggrecan, keratan sulfate, and other large aggregating proteoglycans (present in the mature spine) were not detected at this stage, suggesting that these appear later in development. The notochordal cells themselves showed reactivity to transforming growth factor-β, suggesting an early influence of this growth factor on the developing extracellular matrix milieu.27
In the 20-mm embryo, the notochord becomes an intrinsically segmented structure in the thoracic and lumbar region; in the 30-mm embryo, this structure is evident in the cervical region as well. Segmentation leads to areas of fusiform enlargements in the region of the intervertebral disc, while the notochord is slowly obliterated in the region of the developing vertebral bodies. Within the developing vertebral body, the notochord is stretched into a “mucoid streak” (see Fig. 1–5B). With continued growth, the mucoid streak disappears, leaving behind only bone.
The notochord expands in the region of the intervertebral disc to form the nucleus pulposus. This was originally described in detail by Luschka.28 The notochord is a major source of the nucleus pulposus, and it has been shown histochemically and autoradiographically that notochordal cells proliferate and remain vital several years after birth.29 Although notochordal cells generally do not seem to be demonstrable in the human nucleus pulposus of individuals older than 5 years of age, Schwabe30 reported their survival in the incarcerated discs of the sacrum in a series of specimens ranging from 22 to 45 years in age. A chordoma is abnormal neoplastic growth of notochord cells that remain within the spine in adult life. This suggests that notochordal rest cells can persist well into middle age in some individuals. These neoplasms may develop at any point along the original notochordal track but are usually in the rostral (basisphenoid or basiocciput) and caudal (sacral) regions.
As a theme of development of the spine, the region of the previous notochord lies anterior to the center of the fully developed vertebral body. This has been verified by Nolting and colleagues,25 who detected remnants of notochordal tissue anterior to the cartilaginous body center in 13 fetal spines aged 10 to 24 weeks. This finding further reinforces that using the terms centrum and vertebral body interchangeably is inaccurate.
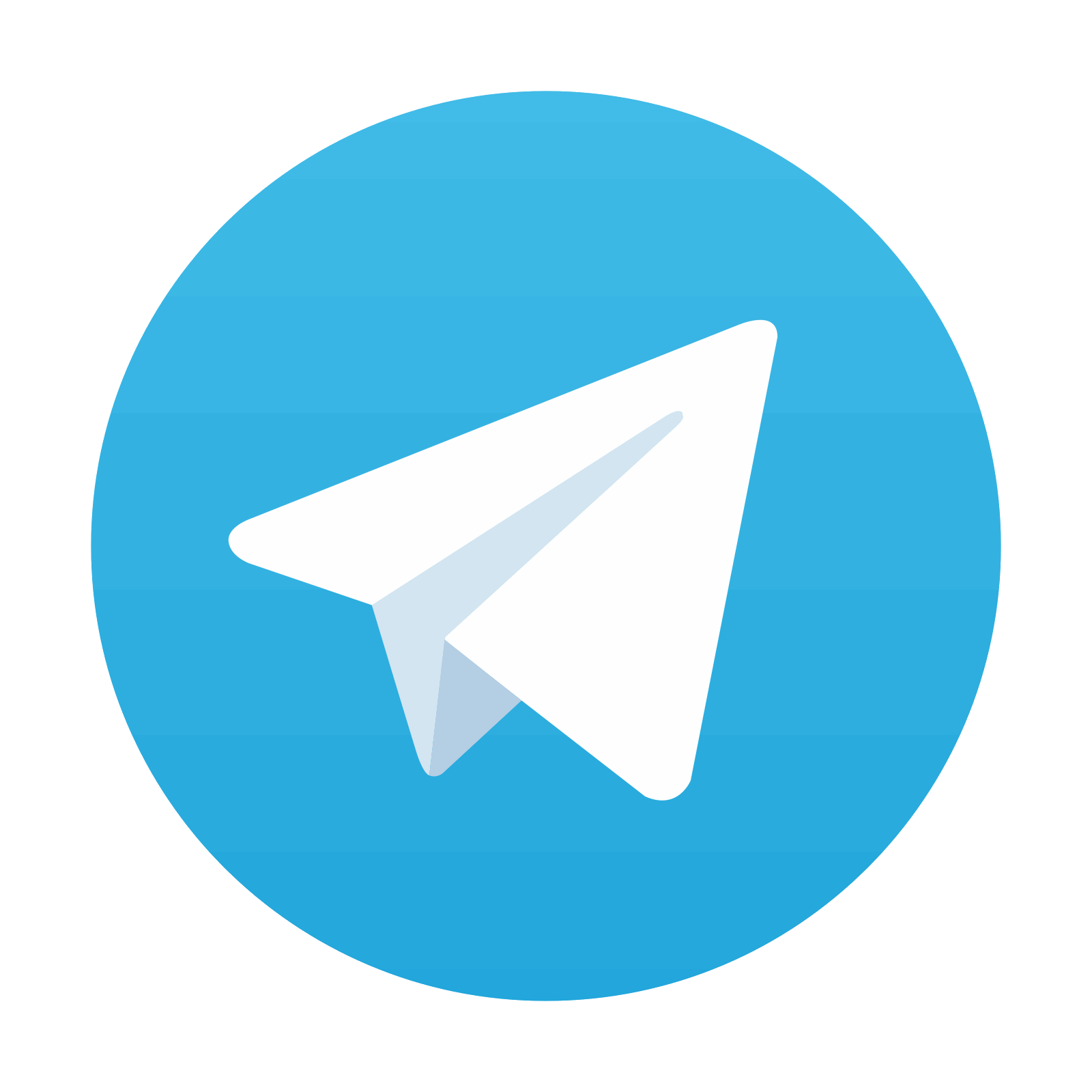