Chapter 116 Computer-Navigated Total Knee Arthroplasty
History of Computer-Assisted Knee Surgery and Evolution of Basic Concepts
Although a large volume of important work that became the foundation for computer-assisted knee surgery was being carried out throughout the 20th century, the initial clinical applications for knee surgery began in the 1980s.109 In 1986, Kaiura, from the University of Washington presented a thesis on robotic-assisted total knee arthroplasty (TKA).39,62 This work led to the design of one of the first computer robotic assistive systems for TKA, described by Matsen and colleagues86 in 1993.137 In the early 1990s, Kienzle and associates65 and Stulberg134 also described a computer-assisted robotic total knee replacement system. The desired position of the femoral and tibial cutting blocks was determined on a three-dimensional model derived from a computed tomography (CT) scan obtained preoperatively. The robot was secured to the operating table and to the bones and then positioned a drill to make holes for the pins over which the femoral and tibial cutting blocks were placed. The surgeon then performed the cuts with a standard oscillating saw. The accuracy of block placement with this system was within 1 mm and 1 degree. This work also introduced a method for determining the center of the femoral head by means of a kinematic registration technique. This technique was subsequently incorporated into all current navigation systems. Dynamic reference frames that were tracked by a camera were placed on the femur and the hip was put through a range of motion. The center of the sphere described during the circumduction maneuver represented the center of the femoral head.
The rapid evolution of surgical navigation systems to support the performance of knee surgery was made possible by the availability, in the early 1990s, of optical and electromagnetic tracking systems (Fig. 116-1). Optical tracking systems have played a special role in the development of surgical navigation systems for knee surgery because of their accuracy and reliability. These tracking systems, also referred to as optical localizers, have charge-coupled devices (CCDs, or cameras) mounted on a rigid frame. These cameras measure the position and orientation of multiple tracking markers, also called trackers, or rigid bodies. Each tracker incorporates a set of light-emitting diodes (LEDs), or reflective spheres, mounted in precise relative positions. These trackers can be affixed to bones, tools, and implants. The optical tracker is therefore able to monitor the precise position of these objects at any point during the surgical procedure.
During the first half of the 1990s, a great deal of basic research was performed to develop surgical navigation systems using these optical tracking systems.109 The first clinical applications incorporating these efforts began in 1995 in the field of spine surgery. Although these applications were based on the acquisition of anatomic information by means of preoperative imaging techniques, they were the basis for the development in the late 1990s of the image-free systems currently most widely used in knee surgery.
Four types of surgical navigation models are used in computer-assisted orthopedic surgery: (1) preoperative image–based (e.g., CT scans)68,75; (2) intraoperative image–based (e.g., fluoroscopy)84,85; (3) image-free; and (4) individual templating.37,75,93,112,130 The anatomic information on which the surgical plan is made is acquired differently in each model. Although each model has its advantages and drawbacks, the image-free method for acquiring critical anatomic information has proved to be most amenable to the methods used currently to perform knee surgery. This method was first used clinically in 1993 to place grafts during anterior cruciate ligament (ACL) surgery.109 Image-free navigation was subsequently applied to TKA surgery by Leitner and colleagues.80 The first image-free computer-assisted TKA was performed in Grenoble, France, by Picard and associates in 1997.107 The system used became the first commercially available image-free navigation system for knee reconstructive surgery (the OrthoPilot).106 It identified critical anatomic landmarks using both kinematic (e.g., femoral circumduction, as described by Kienzle and coworkers in 198965) and surface registration techniques. Krackow and colleagues74 subsequently developed surgical navigation systems based on these concepts. There are now a large number of image-free navigation systems available for use with almost every total knee system.
Rationale For Use
Successful surgical reconstruction of the knee requires proper patient selection, appropriate perioperative management, correct implant selection, and accurate surgical technique. The consequences of performing a knee reconstruction inaccurately have been well documented for TKA, unicondylar arthroplasty, ACL reconstruction, and high tibial osteotomy.*
A surgeon can only use computer-assisted navigation safely and effectively if he or she is familiar with and comfortable with the procedure’s goals and the surgical techniques necessary to achieve those goals. Numerous reports have confirmed that when surgeons experienced in the manual performance of the knee reconstruction procedure use CAS techniques, average implant and limb alignment is improved and the incidence of outliers is reduced.* Moreover, a recent study has indicated that when experienced surgeons and coworkers use techniques to perform knee reconstructive procedures, their ability to perform these procedures manually improves.145
Finally, CAS provides a unique and unprecedented opportunity to train residents and orthopedic surgeons to perform knee reconstruction procedures accurately.95,140,148 CAS as a training tool has now been used more frequently. Applications have been developed and are now being used to allow surgeons to carry out self-assessment evaluation of their surgical skills for performing TKA and ACL surgery. Applications are also being developed to test the skills of surgeons and residents to learn various knee reconstruction procedures. Possibly, the most compelling rationale for applying CAS to knee reconstruction will prove to be the potential to revolutionize how surgeons develop and evaluate their surgical skills.
Hardware and Software Requirements
Hardware
Hardware devices common to CAS systems are (1) imaging devices; (2) computers, peripherals, and interfaces to allow them to function in the operating room; and (3) localizers and trackers (see Fig. 116-1).
Computers, Peripherals, and Interfaces
The computers used in CAS are obviously the core of these systems. They integrate information from medical images, implant data, intraoperative tracking, and surgical plans to guide the surgeon in the performance of a knee procedure. The speed of computing, memory, storage capacity, and communication ability with peripherals have reached a level where even midrange, less expensive personal computers can satisfy the needs of image-free CAS knee applications. All current CAS knee applications use a range of platforms based usually on the UNIX or Windows operating systems. It is likely that applications will soon be written on the open Linux operating system. The computers are currently mounted on transportable carts (or operating room booms) that include the computer, monitor, keyboard, mouse, power transformer and isolation unit, and tracker controller unit with ports to plug in the tracker and tracking markers. Communication between the surgeon and computer is necessary for continuous monitoring of the procedure. This can be accomplished with single or double foot pedals, keypads, touch screens, pointer-integrated controls, or voice-activated controls (see Fig. 116-1A).
Localizers and Trackers
Optical Localization
Optical localization via infrared light is currently the most widely used method of communication between the operated extremity and computer. Two types of optical tracking are used, active and passive. Systems with active tracking use markers (also called trackers, or rigid bodies) with LEDs that send out light pulses to a camera (optical localizer). Three or (for redundancy) more of these LEDs are attached to screws or wires that are rigidly attached to the femur and tibia. The camera system to which the light is sent consists of two planar or three linear CCDs that are rigidly mounted onto a solid housing (Polaris, Northern Digital, Ontario, Canada, is a commonly used camera system). Passive systems use reflecting spheres placed on tracking markers that are attached to screws or pins rigidly implanted in the femur and tibia. Infrared flashes sent by LED arrays on the camera housing illuminate the spheres. The two planar or three linear CCDs observe the reflections and interpolate the spatial location of each light source. It is important for the surgeon and staff to realize that the arrays on the tracking markers, whether active or passive, are specific to each CAS system. One company’s trackers cannot be used on another company’s CAS system, even though the trackers may appear to be similar (see Fig. 116-1).
Ultrasound Systems
Ultrasonic-based navigation systems measure how long a sound impulse needs to travel between the emitter and microphone. Calculation of the position of each tracked object is based on the speed of sound. Although technically feasible, these systems require delicate calibration. Precision depends on the speed of sound, which may vary with differences in temperature. Sterilization of ultrasonic equipment can also be difficult.83
Measured Gap Resection Technique
Preferred Approach
I prefer the navigated measured resection approach for the following reasons:
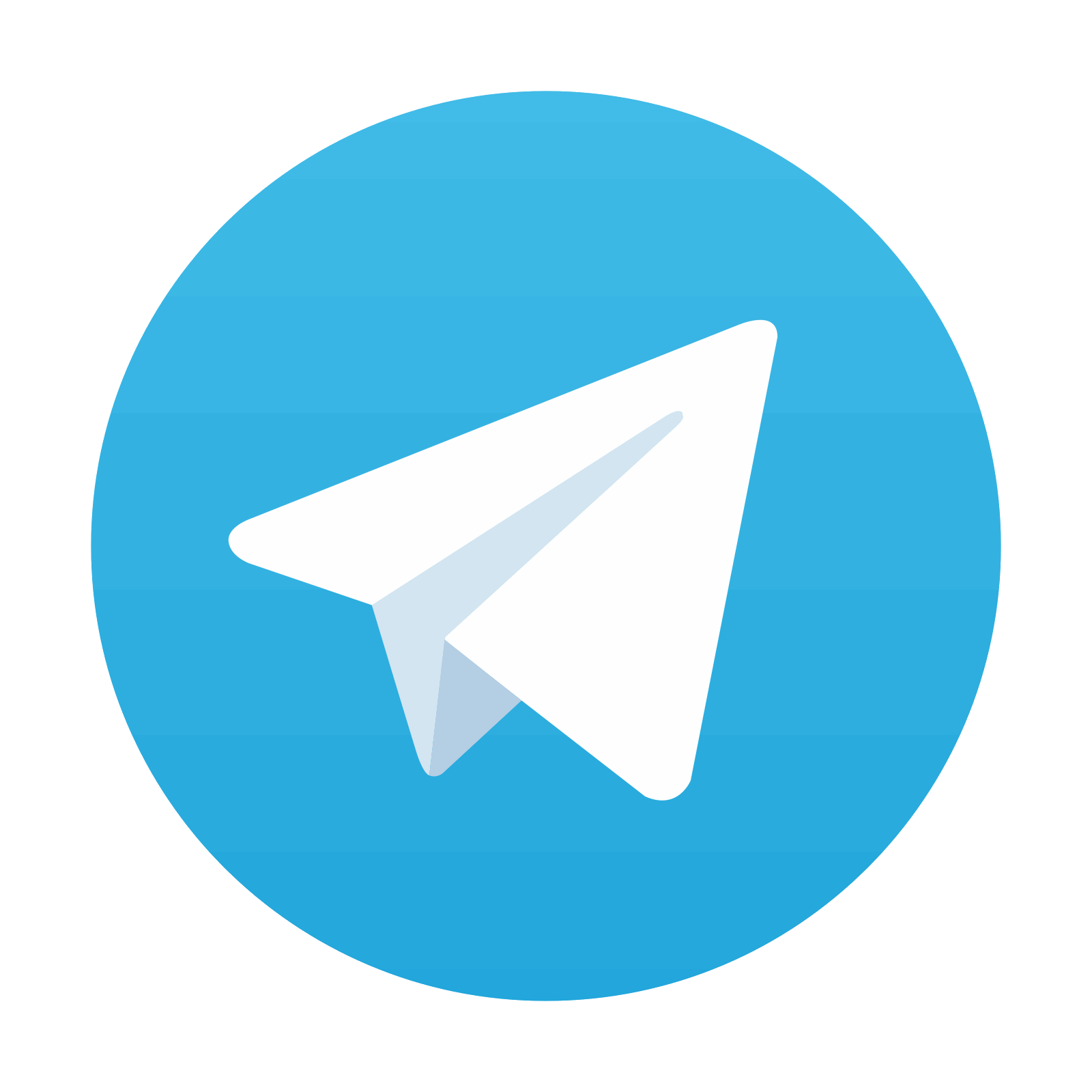
Stay updated, free articles. Join our Telegram channel

Full access? Get Clinical Tree
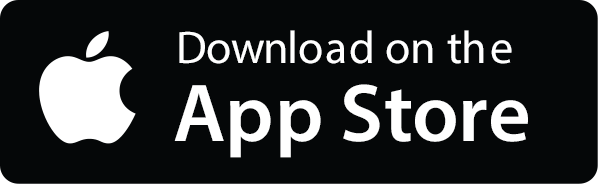
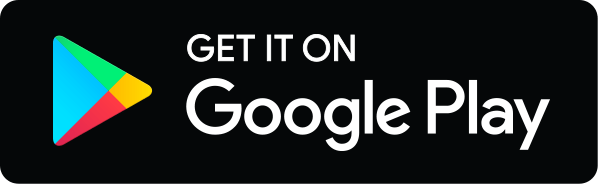