Efferent nerves are further divided into somatic nerves (those under voluntary control) and autonomic nerves (not under voluntary control). The autonomic nerves are also divided into two types – sympathetic nerves (which generally prepare the body for action) and parasympathetic nerves (normally associated with rest and digestive functions).
Neurones
The functional cells of the nervous system are called neurones. There are about 12 billion of these functional cells in the brain, spinal cord and nerves. They are capable of transmitting impulses to and from all parts of the body.
Some neurones are very short, having to transmit impulses extremely short distances within the brain, while some are very long such as those which transmit impulses from the spinal cord to the feet. In addition, various types of supporting cells are found in the CNS and the PNS where they nourish, protect and insulate neurones (Seeley et al., 2003). These cells, called neuroglia, do not transmit nervous impulses. Schwann cells are an example of neuroglia and are found wrapped around many of the axons in the PNS. These cells insulate and protect the axons in the PNS. They also speed up the rate at which nerve impulses are transmitted.
The brain
The brain lies within the cranial cavity of the skull, where it is surrounded by fluid, membranes and bones for protection. It consists of:
- Cerebrum
- Cerebellum
- Diencephalon
- Brainstem.
Cerebrum
The cerebrum is the structure that most people think of when they think of the brain. It is divided into two hemispheres. The motor nerves in each hemisphere control the opposite side of the body. The two hemispheres are internally connected by white matter containing axons connecting the two hemispheres, known as the corpus callosum. These connections allow for communication between the two hemispheres and contribute to, for example, the coordination of movement and the ability to think about complex information.
The surface (or cortex) of the cerebrum is convoluted by folds called gyri and grooves called sulci. The convolutions greatly increase the surface area of the brain in order to accommodate the neuronal cell bodies (which form the grey matter of the brain) which process and integrate information and initiate responses. Without this greatly increased surface area, we could only fit a brain of about one-third the size into the cranial cavity with a corresponding loss of processing power (Saladin, 2001).
Revision Point
Identify where in the brain you can find the following functional areas:
- Visual cortex
- Motor cortex
- Speech cortex
- Sensory cortex
- Auditory centres
- Centre for behaviour and motivation.
The basal nuclei (ganglia) are paired masses of grey matter which lie deep within the cerebral hemispheres. They help to plan, and control muscular movements and posture (Seeley et al., 2005). Damage to the basal nuclei can cause movement disorders such as PD. As we will see later, this disease can impact on a person’s ability to communicate.
Cerebellum
The cerebellum is situated posterior to the medulla oblongata and the pons varolii and inferior to the cerebrum. It is similar in structure to the cerebrum, that is, convoluted on the surface and divided into two hemispheres. The cerebellum is involved in maintaining balance, muscle tone and coordinated movement. It does this together with the cerebrum and basal ganglia.
Diencephalon
The diencephalon lies between the cerebrum and brainstem. It consists of several structures including the thalamus and hypothalamus. The thalamus acts as a relay station for most sensory input before the impulse reaches the sensory cortex. The hypothalamus has several important functions outlined as follows:
- Regulation of homeostasis (constancy within the body)
- Autonomic nervous system (ANS) and endocrine system regulation
- Temperature regulation (thermostat)
- Regulates thirst, hunger and satiety (sense of fullness)
- Regulates emotional responses such as anger, fear, pain and pleasure
- Regulates behavioural patterns in relation to sexual arousal
- Regulates sleeping and wakefulness.
Limbic system
The limbic system is found within the cerebrum and diencephalon and it is, together with the hypothalamus, involved in regulating emotional behaviours such as pain, pleasure and anger. Emotions such as these are often largely communicated using non-verbal means such as facial expression and gestures.
Table 4.1 Vital centres located in the medulla oblongata.
Centre | Regulates |
Cardiac centre | Heart rate and stroke volume |
Respiratory centre (in addition to that located in the pons) | Breathing patterns |
Vasomotor centre | Blood pressure |
Reflex centres | Coughing, sneezing and vomiting |
Brainstem
The brainstem consists of the midbrain, the pons varolii and the medulla oblongata.
The midbrain is composed mainly of nerve tracts passing to and from the brain and spinal cord. It also contains cells involved in eye movements, pupil diameter, the startle reflex and the reflex of turning the head to loud noise or bright light.
The pons contains nervous connections between the cerebrum and cerebellum. It also contains nuclei whose function is to regulate breathing.
The medulla oblongata is the most inferior part of the brainstem and continues inferiorly with the spinal cord (Seeley et al., 2003). It contains ascending and descending nerve fibres as well as a number of cranial nerve nuclei (see section on Cranial Nerves), other nuclei and part of the reticular system. It is an area in which nerves descending from the brain cross over to the opposite side, thus allowing one side of the brain to influence the other side of the body. This also explains how damage to one side of the brain can produce clinical features of disorder on the opposite of the body, as in stroke. The medulla also contains several important ‘vital centres’ which ensure that key physiological functions are maintained. These are indicated in Table 4.1.
The reticular activating system (RAS) is found in the brainstem. This area of the CNS helps to maintain consciousness and is involved in the regulation of the ‘sleep–wake cycle’. Activation of the RAS generally leads to wakefulness, whereas inactivation of the RAS produces sleep.
The spinal cord
The spinal cord lies in the vertebral canal and runs from the brainstem to the level of the second lumbar vertebra. Thirty-one pairs of spinal nerves enter and leave the spinal cord down its length. It is composed of cervical, thoracic, lumbar and sacral segments, taking their name from the part of the vertebral column at which their nerves enter and exit. The spinal cord is shorter than the vertebral column because the former does not grow as fast during development. Sensory nerves carrying information from the body to the brain enter the posterior part of the spinal cord. Motor nerves carrying information to the body from the brain leave the anterior part of the spinal cord.
Internally, the spinal cord is composed of grey matter (cell bodies) found in columns and arranged in an H shape with white matter (nerve pathways) found in tracts surrounding this. A central canal runs down the middle of the cord. This contains a small amount of cerebrospinal fluid. The spinal cord exits the skull at the opening in the base known as the foramen magnum.
The posterior (dorsal) horns of the H shape manage sensory information entering the CNS from the body. The cell body of the sensory neurones lie outside the spinal cord in the posterior root ganglion. The anterior (ventral) horns of the H shape manage motor information leaving the CNS to have an effect on the body.
The axon of a sensory neurone enters the posterior horn of the spinal cord and synapses (interconnects) with another sensory neurone, the axon of which passes up sensory pathways in the spinal cord to the brain. Following interpretation of this information, the axon of a motor neurone passes down the motor pathway in the spinal cord. At the appropriate level in the spinal cord, the first motor neurone will synapse with a second motor neurone in the anterior horn of the spinal cord, and the axon of this neurone then forms the motor nerve. The neurone from the brain down the motor pathway of the spinal cord (first motor neurone) is known as the upper motor neurone, and the neurone from the spinal cord to the muscle (second motor neurone) is the lower motor neurone.
Reflex arc
Most of the impulses arriving at the spinal cord from the peripheral nerves are transmitted to the brain for interpretation and action. However, when the sensory neurones entering the spinal cord interpret a signal as dangerous (e.g. touching a hot surface, standing on a nail), a message is transmitted across the spinal cord to a motor neurone from where the message can be directed straight to a muscle bringing about the action of removing the body from danger. This is called a reflex action and happens without conscious control. At the same time as the reflex is happening, messages are being transmitted up the spinal cord to the brain so that you become aware of the action after it has happened.
Protection of the nervous system
The brain and spinal cord are protected by the bones of the skull and vertebral column, soft tissue membranes known as the meninges and cerebrospinal fluid. The meninges are a triple layer of tissue surrounding the brain and spinal cord. The outermost layer is the dura mater, the middle layer is known as the arachnoid mater and the inner layer is the pia mater. Between the arachnoid and pia mater is a space known as the sub-arachnoid space which contains cerebrospinal fluid. This fluid acts as a shock absorber, further protecting the brain and spinal cord.
Blood supply
Like every other tissue, nervous tissue must have a supply of oxygen and nutrients in order to produce energy for transmitting impulses. The right and left vertebral arteries originate from a major branch of the aorta. On the underside of the brain, they unite to form the basilar artery.
The right and left internal carotids also originate from the aorta. Within the skull, they unite with the basilar artery to form the circle of Willis. Arteries arising from this circle supply the brain with oxygenated blood. The main veins which return blood to the heart from the brain are the internal jugular veins.
Cranial and spinal nerves
Cranial nerves pass to and from the brain and brainstem through holes in the skull. All but one (the vagus or 10th cranial nerve) are concerned with sensation and/or function of the head and neck.
Revision Point
Identify the 12 cranial nerves and their function.
The autonomic nervous system
The CNS is responsible for allowing voluntary control, that is, the things we choose to do or not, for example, sitting, standing, walking, chewing. The ANS is responsible for involuntary control, that is, the things that go on in the body which are not normally under voluntary control but help to maintain homeostasis, such as digestion, control of blood pressure, regulation of body temperature, regulation of amount of light entering the eye and so on.
The ANS has two divisions, namely:
1. Sympathetic division which has a major effect under conditions of stress and activity and prepares the body for action in order to maintain homeostasis. This is known as the ‘fight or flight’ response.
2. Parasympathetic division which is responsible for the day-to-day maintenance of homeostasis largely through rest and digestive functions.
In many organs, the two divisions work in cooperation having the opposite effects in order to maintain homeostasis despite changes in circumstances.
Revision Point
Determine the effects stimulation by the sympathetic and parasympathetic divisions of the ANS will have on the following:
- Heart rate
- Respiratory rate
- Pupil size
- Digestion.
The CNS and ANS work closely together and many structures of the CNS also contain functional parts of the ANS.
Ageing changes in the nervous system
Brain
When healthy, the ageing brain continues to function normally throughout old age. The brain is able to adapt and compensate for any cellular and neuronal changes brought about by the ageing process and an older person remains able to learn during old age. However, the ageing process does affect the different cells and functions of the brain in different ways (Timiras, 2003). Timiras goes on to state that healthy older people seem to display no significant differences when examined using imaging techniques for the weight, size, volume and metabolism of the brain as compared to younger people.
The meninges which surround the brain thicken with age. The dura mater, which is the outer layer of the meninges, holds fast to the skull in very old adults. The blood vessels in the arachnoid layer reflect the vascular condition of the rest of the body (Christiansen and Grzybowski, 1999). This is also reflected in the cerebral blood flow as it diminishes with age. However, the amount of oxygen which is delivered to the brain remains adequate as a result of more oxygen being released from haemoglobin, as increased amounts of oxygen are extracted from the blood (Redfern and Ross, 2001).
There appears to be some shrinkage of the healthy brain during ageing but it does not seem to have any significant effect on an older person’s brain function, including the ability to continue learning (Timiras, 2003). Brain weight declines from ~1400 g in a typical young male adult to about 1200–1300 g in very old adults. Until the age of 50, there appears to be only a small amount of brain weight loss; however, this loss speeds up after the age of 60 (Christiansen and Grzybowski, 1999). Timiras (2003) accounts for the change in the brain weight as a result of the loss of neurones. However, Lueckenotte (2000) suggests that the decrease in brain weight may be due to a reduction in the size of some neurones rather than the loss of neurones.
The loss of brain weight is accompanied by alterations in the appearance and organisation of the brain (Christiansen and Grzybowski, 1999). The gyri flatten and decrease in size while the sulci widen and deepen, resulting in a decrease in the cortical area in the brain. Interestingly, this loss of hemispheric volume occurs at a faster rate in men than in women (Linton and Matteson, 1997). The general shrinkage of brain size results in increased separation from the skull and an increase in the size of the ventricles (Christiansen and Grzybowski, 1999; Linton and Matteson, 1997). Loss of lipid-rich myelin also occurs, resulting in an increase in water and protein content of the brain (Linton and Matteson, 1997).
Neuronal loss
There seems to be a steady loss of neurones in the brain and spinal cord with ageing. At the age of 75, the loss is estimated to be about 10%. However, because there are very large numbers of neurones in the brain, this progressive loss of neurones does not result in a significant loss of mental functioning (Roach, 2001).
Loss of neurones appears to be patchy and affects only some areas of the brain. Neuronal loss also seems to vary from individual to individual. In some areas of the cerebral cortex and in the cerebellum, the number of neurones remains virtually unchanged throughout life, except when people become very old and loss then starts to appear in the cerebellum. Neuronal losses do occur in the locus caeruleus (an area of the brainstem concerned with the physiological response to stress and panic) where catecholaminergic neurones are found, the substantia nigra where dopaminergic neurones are found, the nucleus basalis of Meynert (NBM) and the hippocampus where cholinergic neurones are found. Overall, neuronal loss seems to be fairly small. However, a severe loss of cholinergic neurones or loss of dopaminergic neurones has to occur before the development of Alzheimer’s disease or PD becomes apparent, respectively.
There seems to be a steady loss of neurones with ageing. However, once lost, neurones are not replaced with other neurones. They are replaced with cells known as neuroglial cells, which do not directly influence electrical impulse transmission (Linton and Matteson, 1997). This increase in number of neuroglial (glial) cells is a normal compensatory response to ageing changes in the CNS (Timiras, 2003). However, the number of neuronal cells in the brainstem remains fairly constant into old age. This is significant due to the presence of many vital centres found within the brainstem (Redfern and Ross, 2001).
Neuronal networks
Organisation of neuronal networks is preserved in healthy older people. However, with continued advancing age, the number of dendrites and dendritic branches may decrease. The reduction of dendrites and dendritic branches can result in isolation of neurones and a slowing down or failure of communication between neurones. However, as dendrites undergo continuous renewal throughout their lifespan, the reduction in the number of dendrites may not reflect an actual loss but reflect a slowing down of the renewal process (Timiras, 2003). In areas of the CNS where numbers of dendrites and their branches reduce, there is also a decrease in numbers of synapses. Attempts are made to compensate for this loss by increasing the number of synapses provided by surrounding neurones (Timiras, 2003). There is an increase in dendritic growth in some neurones found in the cerebral cortex and hippocampus. This increase may be associated with an attempt to compensate for neuronal loss in specific areas of the brain (Linton and Matteson, 1997).
With advancing age, a number of changes occur in neurones including a reduction in RNA, mitochondria and enzymes contained within the cytoplasm. The size of nuclei also decreases. Axons degenerate, swell and lose myelin – a process known as dying back. Dendrite changes affect synaptic transmissions as do changes in neurotransmitters (Linton and Matteson, 1997).
Neurotransmitters
Neuronal activity slows down with advancing age. This slowing down is related to the lowered production and metabolism of the main neurotransmitters, especially where nerve conduction is across multi-synaptic junctions (Linton and Matteson, 1997). In healthy older people, neurotransmitter levels and activities undergo minor changes only. Timiras (2003) suggests that functional ability of older adults can be altered due to a loss of balance between excitatory and inhibitory neurotransmitters. An example of such imbalance can be seen in PD where levels of the inhibitory neurotransmitter dopamine decline but levels of the excitatory neurotransmitter acetylcholine remains the same. Due to this change in balance between these two neurotransmitters, one of the most notable features of PD – tremor – is experienced. A decline in norepinephrine has also been found and lower levels of dopamine have been measured especially in the basal ganglia of older people (Christiansen and Grzybowski, 1999).
Lipofuscin and neurofibrillary tangles
Many CNS cells in the older adult contain considerable quantities of the yellow/brown pigment known as lipofuscin. This pigment is linked to the accumulation of free radicals within a number of body cells including cells in heart muscle and in the skin. Lipofuscin is thought to be derived from waste products from partially broken down cell membranes and other cell structures. These wastes are thought to cram the cytoplasm resulting in less effective cellular work (Lueckenotte, 2000). Accumulation of this pigment is part of the normal ageing process and found within the normal ageing brain (Christiansen and Grzybowski, 1999).
Neurofibrillary tangles are insoluble twisted fibres found within cells of the brain. They are composed of a protein known as tau which forms part of a structure called a microtubule. These tangles are found within the cells of the brain as part of the normal ageing process (Redfern and Ross, 2001; Timiras, 2003). However, they are also found within neurones of patients diagnosed with Alzheimer’s disease.
Movement
In most healthy older adults, there is no noticeable loss of cognition. However, there is a degree of loss in sensory function due to the loss of sensory neurones. This can result in impairment of hearing, vision, smelling, temperature regulation and pain sensation (Roach, 2001). Loss of postural control is influenced by the loss of sensory cues, especially visual, tactile and auditory cues. These losses contribute to the increased risk of falls in many older adults. Slower reflexes associated with ageing are common as is a delayed response to stimuli. These changes are related to a reduction in the number of nerve conduction fibres (Roach, 2001). Overall reaction time is slower but older adults are generally aware of this and seem to compensate by responding more slowly but more precisely (Linton and Matteson, 1997). Given time, older people are capable of performing many tasks as good as younger people.
Activity
Consider the environment in which you work or live. Overall, does it help or hinder the movement of older adults? What changes might make it safer?
Changes in the spinal cord
Changes in nerve cells in the spinal cord are similar to the changes found in the brain. By the age of 90, 30–50% of anterior horn cells are lost and 30% of myelinated axons in the posterior roots and peripheral nerves are lost. Larger nerve fibres are lost in the sympathetic chain, which are thought to account for the increased incidence of postural hypotension in older age. The efferent system that carries information away from the CNS also loses its largest fibres, resulting in slower conduction of nerve impulses.
The overall efficiency of the ANS is reduced with advancing age. Loss of nerve cells, slower conduction of nerve impulses and deterioration in peripheral nerve functions all lead to a decrease in its efficiency. Recovery from stress takes longer than before and is often incomplete. Stressors such as heat, cold and extreme exercise can be particularly harmful and even life-threatening (Linton and Matteson, 1997).
There are also changes in the peripheral nerves associated with ageing. Some axons lose their myelin sheath and Schwann cells may become less efficient or cease to function altogether. It is important to recall that Schwann cells insulate the axon of a nerve cell and also speed up the rate of nerve impulse conduction.
Nerve cells and processes in the spinal cord are also lost as the person ages. Over time these nerve cells are replaced with glial cells in a process called gliosis. Deep tendon reflexes deteriorate with age and many reflexes are lost by the age of 90 (Christiansen and Grzybowski, 1999).
With ageing, many tissues and organs respond less well to sympathetic stimulation. Cardiovascular and blood pressure reflexes are significantly reduced with ageing (Redfern and Ross, 2001), making older adults more prone to problems associated with sudden changes in body positions. An example being postural hypotension, where the vasoconstriction reflexes are not quick enough to maintain systolic blood pressure as a person moves from a sitting or lying position to a standing position. If the vasoconstrictor reflexes are not responsive enough, the person may experience dizziness, light-headedness and blurred vision. This also increases the likelihood of falls.
The vertebral arteries supplying blood to the brain tend to become more tortuous with ageing as a result of changes in the cervical vertebrae and intervertebral discs. Specific movements of the neck can make them kink which can temporarily reduce the supply of blood to the brain. Cerebral arteries and capillaries normally have thinner middle and outer layers compared to other arteries in the body. As with other arteries throughout the body, cerebral arteries are also prone to developing atherosclerotic changes associated with ageing. However, the brain is less tolerant of vascular changes and even minor haemorrhages or emboli can have considerable effects on overall function (Christiansen and Grzybowski, 1999).
The eye
Structures of the eye
Each eye is located in a deep cavity in the skull called the orbital cavity. The eyeball is a hollow fluid-filled sphere composed of three layers. It is divided into anterior and posterior compartments. Of the total surface area of the eye, only the anterior 1/6th is exposed (Tortora and Derrickson, 2009). The outermost layer is composed of the sclera and cornea. The sclera, which makes up the posterior 5/6th of the eyeball, is composed of white, fibrous tissue. Anteriorly, the sclera is continuous with the cornea in the front of the eye which is transparent. The sclera maintains eye shape and allows the attachment of muscles. There are six muscles that are attached to the sclera by small tendons, these muscles control eye movement. The cornea is convex in shape and is involved in refracting (bending) light to bring it into focus at the back of the eyeball.
The choroid lines the posterior 5/6th of the inner surface of the sclera (Waugh and Grant, 2006). The choroid contains a large amount of melanin and thus appears black. This black pigmentation absorbs stray light rays so that light is not reflected inside the eye (Seeley et al., 2005). The choroid contains many blood vessels that nourish the retina.
The choroid terminates at a structure called the ciliary body. The ciliary body contains smooth muscles (and is therefore not under conscious control). The lens is suspended from these muscles by suspensory ligaments which protrude from this body. The lens is a transparent, flexible, biconvex disc. By contracting and relaxing these muscles, it is possible to change the shape of the lens and thus ensure light rays are brought to a focus on a point at the back of the eye in the central fovea. This area contains only cone cells; cones are the nerve cells responsible for detecting colour.
The iris is the coloured portion of the eye. It is composed of circular and longitudinal muscles. The iris is attached to the anterior edge of the ciliary body and sits in front of the lens. The iris determines how much light enters the eye. Its diameter is controlled by autonomic reflexes via the 3rd cranial (oculomotor) nerve. The activity of the iris protects the sensitive retina from excessive, harmful light rays.
The retina is the vascular innermost lining of the eye where the light-sensitive nerve cells, rods and cones, are found. Cones are responsible for colour vision and function predominantly in daytime. The rods are most active in night vision.
Aqueous humour and vitreous humour
The anterior compartment that lies between the cornea and the front of the lens contain a fluid known as aqueous humour. This compartment is divided into two chambers. The anterior chamber lies between the cornea and the front of the iris. The posterior chamber lies behind the iris but in front of the lens. This is a fluid which maintains the eye’s shape. There is a continuous circulation of aqueous humour, such that it is completely replaced every 90 minutes (Tortora and Derrickson, 2009). Vitreous humour is found in the posterior compartment between the lens and the retina. This jelly-like substance prevents the eye from collapsing.
Accessory structures
The accessory structures of the eye consist of eyebrows, eyelids, lacrimal (tear) apparatus and eyelashes. All have protective functions. They prevent dust, sweat and foreign objects from entering the eye. Tears are formed in lacrimal glands; they keep the eye moist, the tears then flow into the nose via the nasolacrimal ducts located at the inner aspect of the eye.
Physiology of vision
In order to see clearly, light must be brought into focus on the retina. This is achieved through a number of mechanisms and these are discussed below.
Refraction
Refraction refers to how light waves are bent as they move from one medium to another. Moving through the different structures in the eye, the light rays bend four times. The result of all this bending is that the light rays land on a portion of the retina which is rich in nerve endings.
Accommodation
To ensure that large objects and small objects can both be projected on the back of the eye, it is necessary to have a mechanism in the eye which can alter its capacity to bend waves. The lens of the eye does this. It can become fat and squat and thus bends waves acutely or can become flat and thin thus hardly bending them at all. This manipulation of waves is called accommodation.
Constriction
The pupil is an open space which allows light to enter the eye. It is created by the iris which is composed of circular and radial muscles. By contracting or relaxing, the iris widens or reduces the hole through which light enters the eye. Thus, the entry of light into the eye is controlled and we are not blinded in bright light and we can see reasonably well in dark conditions.
Convergence
As we have two eyes, it is important that light from an image strikes the same area in each retina. In this way a clear image will be produced. To achieve this, the eyes will move, or converge, to bring the object into a clear focus. Convergence is controlled by the ANS activating the external muscles of the eye. If this did not occur, the two eyes would focus on slightly different parts of an object resulting in double vision or diplopia.
Retina and the conversion of light to an electrical signal
The retina is the photosensitive portion of the eye, that is, it is sensitive to light. When light hits the rods and cones within the retina, photosensitive pigments within them breakdown and action potentials are generated. The action potentials are carried via the optic nerve (second cranial nerve) through the thalamus to the visual cortex in the occipital lobe of the brain where they are interpreted. It should be noted (Figure 4.2) that the information from half of each image is carried to the opposite side of the brain.
Visual purple (rhodopsin) is a photosensitive pigment which is only found in rods. This pigment requires an adequate supply of vitamin A for proper functioning. If lacking in vitamin A, a person may suffer from night blindness in which they have difficulty in seeing in dim light. The cones contain different photosensitive pigments that are sensitive to coloured light, namely red, green and blue. We can see a wide range of colours as cones are stimulated in different combinations.
Figure 4.2 Diagram of visual pathways. (Reproduced from Tortora and Derrickson (2007). With permission from John Wiley & Sons.)
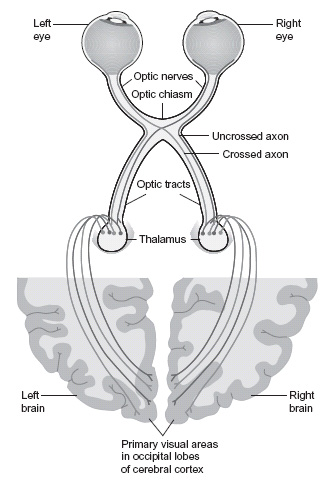
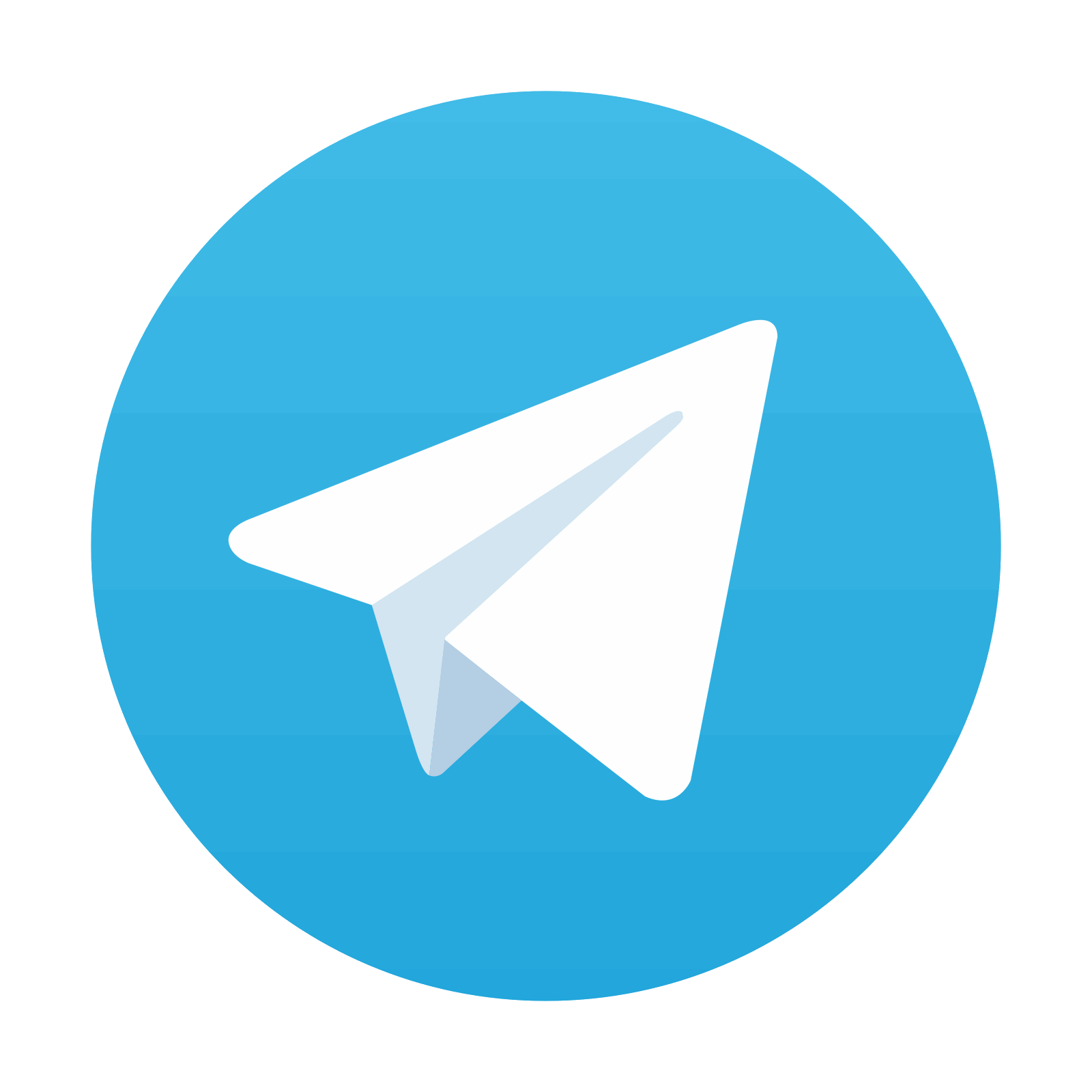
Stay updated, free articles. Join our Telegram channel

Full access? Get Clinical Tree
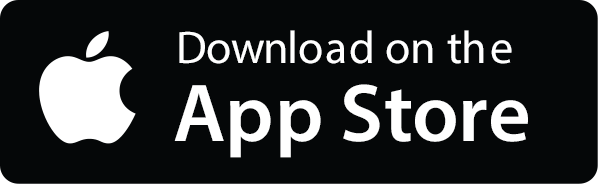
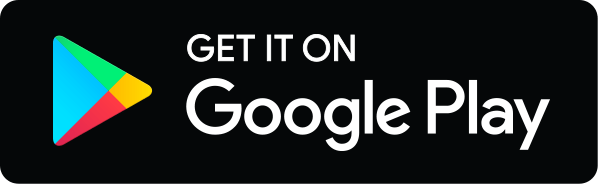