the blood vessels to be forced through the walls of these blood vessels and the walls of the glomerular capsule in to the next part of the nephron. The fluid and small components forced into the proximal convoluted tubule (the next part of the nephron) are now referred to as filtrate.
The renal tubule is divided into three parts – the proximal convoluted tubule, the loop of Henle and the distal convoluted tubule. Within these parts, water and small components contained within the water are re-absorbed back into blood. In fact, 99% of water that helps to form the filtrate is re-absorbed within the renal tubule. In other words, for every 100 ml of filtrate that is formed, only 1 ml of that filtrate will become urine as 99 ml will be re-absorbed (see Box 7.1 for a list of filtrate components). Waste products not removed from blood through the process of glomerular filtration are removed by the process of secretion from the surrounding blood vessels (peri-tubular capillaries) into the renal tubule. Secretion is the opposite of re-absorption (Thibodeau and Patton, 2004), where dissolved substances move from blood into filtrate. These can include ammonia, hydrogen ions, potassium ions and certain drugs.
Distal convoluted tubules from several nephrons join to form a single collecting duct. Urine from the collecting duct now exits the renal pyramid into the calyx and renal pelvis before flowing into the ureter (for composition of urine see Box 7.2).
Box 7.1 Composition of glomerular filtrate
Glucose
Amino acids
Fatty acids
Salts (e.g. sodium and potassium)
Urea (from breakdown of proteins)
Uric acid (from breakdown of proteins)
Water
Box 7.2 Composition of urine
96% water
Salts – mainly sodium chloride
Waste products of protein breakdown:
Urea
Uric acid
Creatinine
NB: Urine should not contain blood cells, protein, glucose or ketones.
The glomerular filtration rate (GFR) refers to the amount of filtrate that forms in both kidneys every minute. In males, this amount is ~125 ml per minute, and females produce ~105 ml per minute (Tortora and Grabowski, 2004). The GFR has to be constant. If the GFR is too high, the tubules do not get the necessary time to re-absorb substances and water, and as a result, these necessary substances and excess water are removed from the body. If the GFR falls, the speed at which fluid and its solutes pass through the renal tubules also decreases. As a consequence of this reduction, more time is allowed for re-absorption of dissolved substances and water. The result of which is that waste products are re-absorbed rather than excreted and more water is also re-absorbed, and hence less urine is produced.
Anti-diuretic hormone (ADH) is produced in the hypothalamus and stored in the posterior pituitary gland. ADH is released into the bloodstream when the water content of blood decreases even by a relatively small amount. It is also released in relation to a low blood volume. When it is released it has an effect on the renal tubules. It increases the rate at which water is re-absorbed. As a result of its release, more water is re-absorbed and hence less urine is produced. In the absence of ADH, less water is re-absorbed and hence more urine is produced and excreted from the body. This dilute urine will be of low specific gravity and generally colourless.
Aldosterone is a hormone released from the adrenal cortex. It is released in response to a low blood volume or a low plasma sodium level. When aldosterone is released, it also acts on the renal tubules enhancing the re-absorption of sodium and chloride with the added excretion of potassium. When more sodium is re-absorbed, more water is also re-absorbed. As a result of this, less sodium is excreted and less urine is produced and excreted.
Atrial natriuretic hormone (ANH) is produced by the atria of the heart. It has the opposite effect of aldosterone, where it stimulates the renal tubules to excrete sodium rather than re-absorb sodium. In this way, excess fluid can also be removed from the body.
The production and release of ADH, aldosterone and ANH are dependent upon body requirements and demands. When the body is dehydrated, ADH and aldosterone will be released (Tortora and Grabowski, 2004). On the other hand, if the body requires to lose fluid, ANH will be released and the release of ADH and aldosterone will be inhibited. ADH will also be released in response to raised blood osmotic pressure; a reduction in blood volume; pain or stress (Tortora, 2005).
Renin-angiotensin mechanism
When blood pressure falls, the kidneys respond to this stimulus by releasing the enzyme renin. Renin acts on the plasma protein angiotensinogen and converts it to angiotensin 1. This in turn is converted to angiotensin 2 by angiotensin 1 converting enzyme (ACE). Angiotensin 2 is a very powerful vasoconstrictor in its own right. It also stimulates the release of aldosterone from the adrenal cortex.
Aldosterone, as we have already seen, enhances the re-absorption of sodium and water from the renal tubules. This increased re-absorption of water adds to the volume of fluid circulating in the blood (CBV). In turn, the increased CBV increases venous return (the volume of blood returning to the heart), which increases stroke volume (the volume of blood ejected from either ventricle on each contraction). The increased stroke volume adds to the cardiac output (CO = HR X SV) which in turn helps to increase blood pressure (BP = CO X PR).
Activity
The renin-angiotensin mechanism is complicated and complex. In order to enhance your understanding of these processes, summarise the renin-angiotensin mechanism above into note form and list the events associated with this mechanism which will be initiated when blood pressure falls.
One ureter runs down from each renal pelvis on either side of the vertebral column and enters the urinary bladder. Urine is actively propelled down the ureters by peristaltic action, by the pressure of urine within these tubes and to a lesser degree by the action of gravity.
The bladder
The bladder acts as a temporary reservoir for urine. Its capacity to hold urine is between 700 and 800 ml (Tortora and Grabowski, 2004); however, we begin to experience the need to void when the volume of urine in the bladder lies between 200 and 400 ml. This initial experience of the need to void can be overruled if the time or place is not appropriate. This occurs because the external urethral sphincter muscle and muscles of the pelvic floor are under conscious control and the person can choose when and whether to relax these muscles. However, these preventative actions cannot last indefinitely and there comes a point when voiding cannot be delayed.
Three layers of the detrusor muscle (smooth muscle) make up the bladder wall. The lining of the bladder is wrinkled when the bladder is empty, lying in folds called rugae.
When the bladder fills with urine, these rugae flatten allowing the inner wall to become smooth. This action increases the capacity of the bladder to hold urine.
The urethra
This tube connects the bladder to the external environment. It is ~20-cm long in males and 4-cm long in females. The relative shortness of the urethra in females can contribute to an increased prevalence of UTI in women. The urethra has a single purpose in females – elimination, but a dual purpose in males – elimination and for the passage of semen.
Micturition
As the bladder gradually fills with urine, the pressure within it slowly increases and the bladder walls are stretched. When the volume of urine within the bladder reaches between 200 and 400 ml, stretch receptors within the bladder wall are stimulated. Sensory impulses are then sent to the sacral segment of the spinal cord.
This incoming sensory information is integrated within the sacral segment of the spinal cord. Sensory impulses are also sent to the cerebral cortex, which initiates a conscious desire to expel urine. Once the sensory information has been integrated within the sacral segment of the spinal cord, the micturition reflex is initiated.
Parasympathetic impulses are sent to muscles in the bladder wall and to the internal urethral sphincter. The muscles in the bladder wall contract and the internal urethral sphincter relaxes (autonomic reflex). The cerebral cortex now initiates, if desired, voluntary relaxation of the external urethral sphincter and urination takes place.
Ageing changes in the urinary system
The urinary tract is directly and indirectly affected by ageing. Direct effects are typified by intrinsic cellular changes involving nephrons and the muscles of the bladder. Indirect effects may be secondary to cardiovascular, endocrine or metabolic alterations associated with the ageing process (Timiras and Leary, 2003). The age of onset of change, the speed of change and the overall course of change and its consequences differ between individuals.
From the age of 30, renal function gradually decreases and by the age of 80 it is reduced by one half (Timiras and Leary, 2003). The decline in renal function is associated with a gradual loss of nephrons and a reduction in enzymatic and metabolic activity of cells of the renal tubules. This decrease in renal function can also be associated with pathophysiological changes such as atherosclerosis, resulting in a reduction in blood flow to the kidneys. Indeed, renal blood flow can be decreased significantly as the ageing process progresses. However, ageing kidneys continue to function normally (Christiansen and Grzybowski, 1999).
As previously stated, each kidney has at least 1,000,000 nephrons. As adults age, they can lose as much as 50% of these nephrons (Heath and Schofield, 1999); however, Christiansen and Grzybowski (1999) state that older adults normally lose about 25% of their nephrons. This loss normally has little impact on the body’s ability to regulate body fluids. As a result, adequate fluid homeostasis is generally maintained in later life.
A decrease in kidney weight of 20–30% occurs, with the loss primarily occurring in the cortex. Also associated with the ageing process is a decrease of ~20% in kidney size (Christiansen and Grzybowski, 1999). With increasing age, the glomeruli and associated nephrons are replaced by scar tissue (5–37% between the ages of 40 and 90 years).
Glomerular filtration rate
Ageing results in a gradual reduction in renal blood flow, GFR, sodium excretion and re- absorptive ability of the renal tubules (Forrest, 2000; Muhlberg and Platt, 1999). The loss in numbers of functional nephrons reduces the GFR. Timiras and Leary (2003) state that the decrease in glomerular function may be primarily due to a loss or alteration of glomer- uli and secondary alterations in blood flow. Glomerular filtration appears to be affected at an earlier age, and more severely, than tubular re-absorption and secretion. GFR and renal blood flow decrease progressively and significantly as one ages. Christiansen and Grzybowski (1999) state that GFR gradually decreases from the age of 30 until about 45 and that it continues to decrease more rapidly thereafter.
These changes result in a decrease in GFR after the age of 40, equivalent to 1% per year (Heath and Schofield, 1999). Furthermore, in the presence of decreased cardiac output (associated with changes within the cardiovascular system), the elimination of waste is also reduced. Creatinine clearance can be used as a measure of the effectiveness of GFR. Creatinine clearance declines from 140 ml/minute at the age of 29 to 97 ml/minute at the age of 80 in 30% of people (Christiansen and Grzybowski, 1999). However, these changes have little impact on the well-being of older people.
Changes associated with ageing in glomerular function do not pose any threat to well- being, likewise despite loss of nephrons and a reduction in GFR, renal function is not compromised by ageing. However, problems may occur when intrinsic renal disease exists or other factors such as inappropriate diet contribute to renal dysfunction. Diets high in protein may induce glomerular damage; conversely, a reduction in protein intake can reduce this damage (Timiras and Leary, 2003).
Creatinine
Creatinine is a breakdown product of skeletal muscle. Associated with the ageing process is a reduction in overall skeletal muscle mass. The reduction in the urinary output of creatinine may reflect the reduction in skeletal muscle that occurs with ageing.
Day/night changes in renal function
A circadian rhythm of urine production is usually established by 5 years of age (Ali and Snape, 2004). During adulthood, 75% or more of daily urine production and output occurs during the day, that is, 25% or less of urine production occurs during the night. Kidneys will concentrate urine produced overnight so that voiding is not necessary. After the age of 60, a change to more nocturnal urine production occurs. The ratio of day to night urine flow falls until the latter equals or exceeds the former. The kidneys also lose their ability to concentrate urine overnight and as a result the need to void increases. However, the total 24 hour urine excretion does not change (Ali and Snape, 2004).
This change in urine production and output has been associated with a decrease in renal-concentrating capacity, sodium-conserving ability and decreased secretion in the renin-angiotensin/aldosterone mechanism. A reduced action of the renin-angiotensin mechanism leads to a reduction in the amount of sodium re-absorbed within the renal tubules. This in turn reduces the volume of water re-absorbed within the tubules and, consequently, more urine is produced and excreted. The change in ratio of day to night urine produced and excreted may also involve the hormonal influence of ADH, where there may be a deficiency in its production and secretion (Ali and Snape, 2004; Timiras and Leary, 2003). A reduction of ADH secretion leads to less water being re-absorbed within the renal tubules and therefore an increase in urinary output.
As stated earlier, the ability to concentrate urine may be gradually lost, with the result that older people may be unable to cope optimally with dehydration or fluid overload. Provided the total water intake is 2.5–3 l daily, renal function will be adequate (Timiras and Leary, 2003). Problems may arise when water intake is reduced as a result of social isolation, reduced mobility, confusion, a reduction in the thirst mechanism associated with ageing or fear of incontinence (Timiras and Leary, 2003). In these instances, a reduced fluid intake will automatically lead to a reduction in GFR. This can lead to dehydration. This condition may also occur with the use of diuretics. Older people are also more likely to develop hyponatraemia and hypokalaemia during diuretic therapy as sodium and potassium are excreted in excess from the body.
Hormones
Christiansen and Grzybowski (1999) state that the distal convoluted tubule and collecting ducts probably lose some of their responsiveness to ADH, making it more difficult for water to be returned to the body, that is, more water is lost from the body. They go on to state that a decrease in sodium re-absorption across the loop of Henle can also occur. This can result in a reduction in the volume of fluid being re-absorbed into the body. As a consequence of this, the person may experience a lowering of blood volume and of blood pressure.
Several of the hormonal functions of the kidney have been shown to decline with age (Christiansen and Grzybowski, 1999). Renin secreted by the juxta-glomerular cells and aldosterone secreted from the adrenal glands both decrease with age. A reduction in renin and aldosterone will lead to an increase in urinary output and an increase in sodium excretion.
Anti-diuretic hormone is the main hormone responsible for the regulation of urine production (Ali and Snape, 2004). During the hours of sleep, there is a circadian rhythm of ADH with peak concentration occurring during the night. With ageing, there is a reduction in nocturnal ADH secretion, resulting in day and night levels of this hormone becoming very similar. This will contribute to the increased urinary output overnight.
Atrial natriuretic hormone (ANH), through its action on the kidney, results in excessive loss of sodium in urine and an increase in urinary output. ANH levels have been shown to increase with age which can also lead to an increased urinary output.
ANH has been shown to interact with the renin-angiotensin-aldosterone mechanism (Ali and Snape, 2004). High levels of ANH suppress renal secretion of the enzyme renin, which in turn reduces levels of angiotensin 2 and of aldosterone. As a consequence of this reduction in aldosterone, urine and sodium excretion is increased. ANH also opposes ADH action in the kidney and inhibits ADH release from the posterior pituitary gland (Ali and Snape, 2004). Therefore, ANH may contribute to age-related changes in urine excretion, that is, an increased loss of urine and sodium from the body.
With age, there is a decrease in the ability of the kidneys to concentrate urine (Ali and Snape, 2004). This decline in renal-concentrating ability is thought to be related to a change in renal tubular responsiveness to ADH (Ali and Snape, 2004).
With normal ageing, bladder capacity and bladder compliance decrease as do urinary flow rates and maximal urethral closure pressure. Frequency of detrusor contractions and the volume of urine retained within the bladder post voiding also increase with age (Rutchik and Resnick, 1998). These factors interfere with the bladder’s ability to store urine successfully (Ali and Snape, 2004). As bladder muscles weaken, changes in the bladder storage capacity occur and incomplete emptying may ensue. Hormonal changes associated with ageing in females affect detrusor function and sphincter mechanisms, resulting in an increased likelihood of urinary leakage (Rutchik and Resnick, 1998).
Common problems of the urinary system
In the following section, we shall go on to consider two problems of the urinary system that are particularly evident in older adults. These are urinary incontinence and UTI.
Urinary incontinence
Urinary incontinence has been defined as the involuntary leakage of urine, and it is a common health problem among older people (Mauk, 2006). According to Wagg et al. (2008), it has been estimated to affect ~20% of people living in the community and a range of 30–60% of older people living in institutional care. SIGN 79 (2004) estimates that urinary incontinence rises with increasing age where up to 46% of women and 34% of men over 80 will have urinary incontinence. It is generally categorised as being either transient or established based on its onset and aetiology. Transient incontinence is resolved following treatment and has been associated with many causative factors such as infection, drugs, endocrine disorder, reduced mobility and faecal impaction (Table 7.1). Poor management of patients who have transient incontinence can also lead to the diagnosis of established incontinence. If factors associated with transient incontinence are identified and treated without success, the incontinence is then considered to be established (Mauk, 2006).
Wagg et al. (2008) state that incontinence continues to be under-reported despite the significant impact on quality of life and associated morbidity. Many older people who are incontinent cope in silence or do not present for care (Dingwall and McLafferty, 2006). They may not receive the necessary treatment for their condition (NHS Health Advisory Service, 1997; Wagg et al., 2008). This not only negatively impacts on the life of the individual but also on the lives of their carers.
People who are incontinent require proper assessment and management. The NSF for Older People (DH, 2001) stated that integrated continence services for older people should
Table 7.1 Common causes of transient incontinence.
Common cause | Rationale |
Delirium/dementia | Impaired cognitive ability causes problems in recognising/responding to the need to void |
Infection | UTI can cause urinary frequency and urgency |
Psychological | Depression may result in loss of motivation to maintain continence status |
Medications | Diuretics |
Endocrine disease | Hyperglycaemia, hypoalbuminemia and diabetes insipidus are all associated with polyuria which increases the fluid load on the bladder which increases the risk for urge and stress incontinence |
Reduced mobility | Interferes with the ability to reach a toilet in time resulting in urinary leakage |
Faecal impaction | Over-distension of the rectum or anal canal can obstruct the bladder neck resulting in retention of urine, overflow and urge incontinence |
be established by 2004. However, they did not allocate any resources to promote this objective. Wagg et al. (2008) published results from their audit on continence care which indicates that although older people are asked routinely about bladder problems, this did not guarantee an assessment of the problem, nor lead to appropriate interventions.
In older people, the cause and type of incontinence is often undiagnosed (Wagg et al., 2008). This can impinge on its management as without a known cause, evidence-based, effective treatment cannot be provided. Several authors suggest that management of incontinence in the older population is all too often reliant on conservative methods aimed at containment (Thuroff et al., 2006). This suggests an almost inevitability of incontinence in older adults. Indeed, Stoddart et al. (2001) state that incontinence in older people is often viewed as inevitable, irreversible and as a normal part of ageing. This approach to management denies the older person who is incontinent from the more proactive and curative measures which are utilised with considerable success in younger populations who are incontinent.
Classification of urinary incontinence
There are four different types of urinary incontinence, namely:
- Stress
- Overflow
- Urge
- Functional.
Stress incontinence is where there is an inability to hold urine as the bladder fills up. Urethral pressure is exceeded by pressure in the bladder (Doughty, 2000) and urine is forced from the bladder. This is often due to an incompetent urethral sphincter (sphincter weakness) and usually occurs during exertion, or following increased abdominal pressure, for example, when coughing or sneezing or even when laughing out loud. This can occur in either sex, but is most common in women due to their shorter urethra (Palmer, 1996) and the physical process of childbirth, where the base of the bladder may prolapse slightly through the muscle layer of the pelvic floor, thereby rendering the sphincter mechanism incompetent (Palmer, 1996).
Overflow incontinence is due to obstruction to the outflow of urine during micturition. Bladder outlet obstruction can be due to prostatic enlargement (hypertrophy, carcinoma and inflammation), bladder neck narrowing (contracture) or urethral obstruction (urethral stricture). The bladder over-distends due to increased resistance to the outflow of urine, but urine tends to ‘spill out’. The person typically passes frequent small amounts of urine (Doughty, 2000). Symptoms include frequency, dysuria (pain on passing urine), poor urinary stream, urgency, hesitancy and pre- or post-urination dribble. There is incomplete emptying of the bladder leaving residual urine behind.
Occasionally, rather than involuntary urine leakage, the bladder is unable to expel its urine. This is described as urinary retention. Retention is usually due to complete bladder outflow obstruction, for example, due to prostatic enlargement in males, but can occur in post general anaesthesia due to smooth muscle inactivity.
Urge incontinence may be idiopathic (without a known cause), or secondary to conditions such as cystitis (inflammation of bladder and ureters), urethritis (inflammation of urethra), bladder stones or neoplasm. With this type of incontinence, the urge to pass urine becomes overwhelming and urine is passed before a toilet can be reached. Urge incontinence is caused by early contraction of the bladder which is due to premature sensory impulses being sent between the bladder and the brain. These premature impulses indicate that the bladder is full when it is in fact not and the bladder muscle starts to contract too early (this is also called detrusor instability). There is an ever present desire to void (pass urine), which if not satisfied could lead to incontinence. Typically, the person complains of discomfort, frequency, urgency and nocturia (excessive urination at night).
Functional incontinence refers to incontinence associated with factors external to the urinary tract. These can include cognitive impairment, physical disabilities and environmental barriers (Ouslander, 1994).
Urinary tract infection
Normally urine is sterile, containing water, salts, urea, uric acid and other waste products. Urine is normally free from bacteria, viruses and fungi. Infection occurs when microorganisms, often bacteria from the digestive tract, colonise the urethra and begin to multiply. The distal urethra is commonly colonised by vaginal and/or bowel flora (Baileff, 1999). The commonest cause of UTI isE. coli, which normally lives in the colon. This organism is responsible for an estimated 85–90% of all acute uncomplicated UTIs (Baileff, 1999).
Activity
Identify which other microorganisms are most likely to contribute to a UTI in older people. You may wish to use the following resource to help you: “http://www.merck.com/pubs/mmanual_ha/sec3/ch56/ch56a.xhtml.
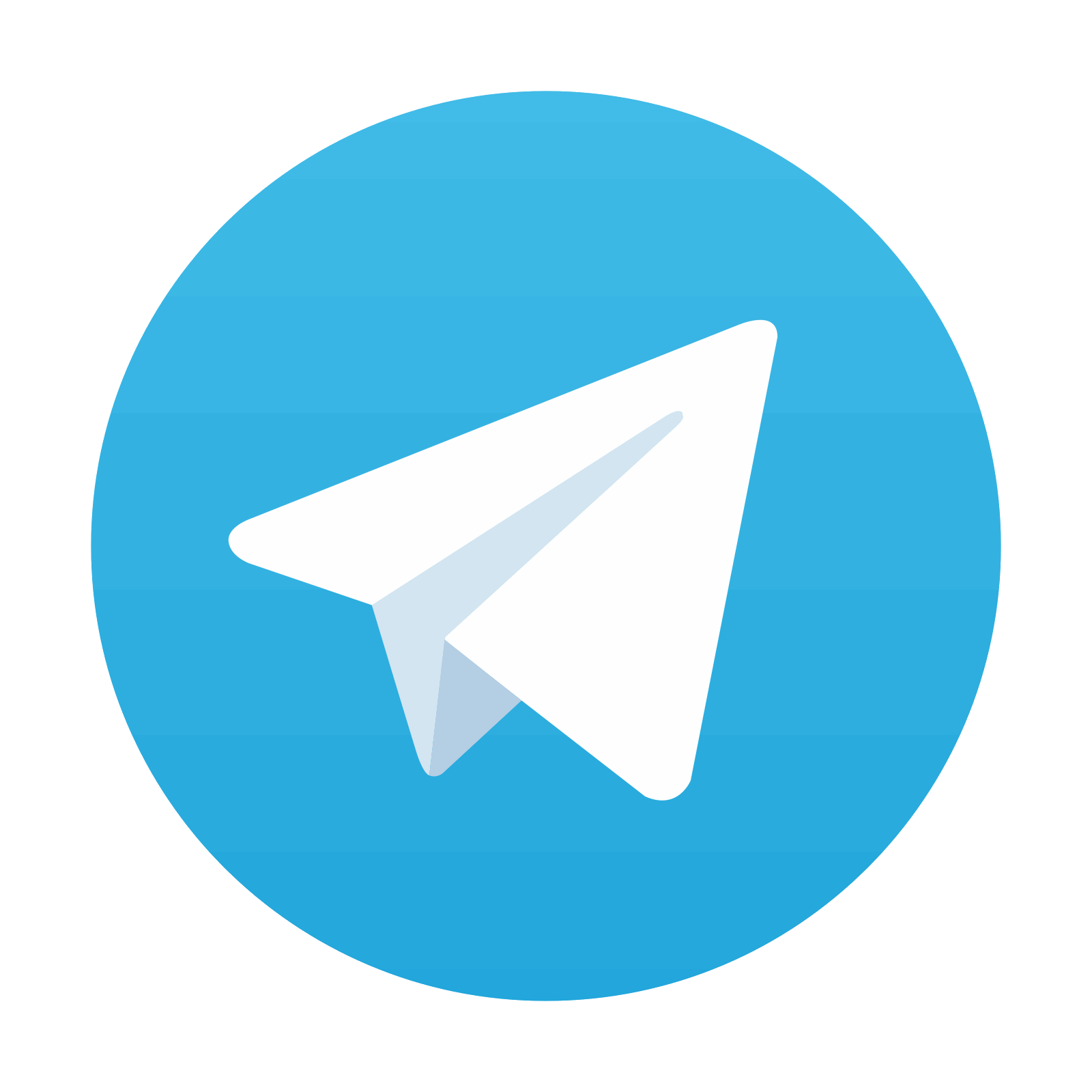
Stay updated, free articles. Join our Telegram channel

Full access? Get Clinical Tree
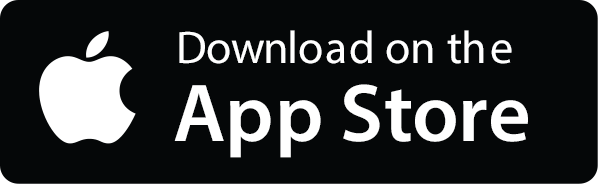
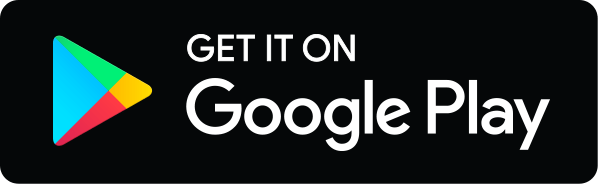