CHAPTER 1 Clinical anatomy and biomechanics of the glenohumeral joint (including stabilizers)







Introduction
The glenohumeral joint provides the largest range of motion among all diarthrodial joints but also has the greatest propensity for instability. The glenohumeral joint has six degrees of freedom of motion that can be described by three rotations and three translations with respect to the anatomic coordinate system (Fig. 1-1). The plane of elevation is defined relative to the coronal plane perpendicular to the transverse plane. Abduction is described as the angle of elevation in this plane. The rotation of the humerus around its long axis is described as internal and external rotation relative to its anatomic neutral position. The translations of the glenohumeral joint can be described by the amount of the translation in anterior-posterior, superior-inferior, and medial-lateral directions. The glenohumeral joint motion is a result of a complex interplay between the passive and active stabilizers that require intricate balance and synchronicity. This chapter addresses the anatomy and biomechanics, especially the recently acquired knowledge, pertaining to diagnoses and treatments frequently encountered by the shoulder surgeon. In addition, the anatomy and biomechanics are described from their functional perspective as passive and active stabilizers. The bony passive stabilizers include the glenoid, humeral head, and the proximal humerus. The soft tissue passive stabilizers include the glenoid labrum, the glenohumeral ligaments, and the glenohumeral joint capsule. The active stabilizers include the tendons and the muscles of the rotator cuff, biceps, deltoid, pectoralis major, and the latissimus dorsi.
Anatomy and biomechanics
Passive bony stabilizers
The bony passive stabilizers include the glenoid, humeral head, and proximal humerus (Fig. 1-2). Glenohumeral articulation is minimally constrained and thus permits both rotations and translations. This inherent instability of the glenohumeral joint is reflected by the fact that only 25% to 30% of the humeral head is in contact with the glenoid surface at any given anatomic position.1–3 This dimensional relationship between the humeral head and the glenoid, referred to as the glenohumeral index, can be calculated as the maximum diameter of the glenoid divided by the maximum diameter of the humeral head (Figs. 1-3 and 1-4). It has been reported that this ratio is approximately 0.75 and 0.6 in the sagittal plane and transverse plane, respectively.4 These authors also reported that the contact area moves superiorly with elevation.4 More recently, it has been reported that there was a slight articular mismatch in adduction but that the glenohumeral joint became more congruent and stable in abduction.5 The contact area shifts from an inferior region to a superior and central-posterior region, whereas the glenoid contact area shifts posteriorly.6,7
To characterize bony stability, Lippitt et al8 defined the “stability ratio” as the force necessary to dislocate the humeral head from the glenoid divided by the compressive load. This stability ratio is dependent on the concavity of the glenoid and therefore increases with higher glenoid depths (Fig. 1-5). The labrum also contributes to stability as the stability ratio decreases by approximately 20% after the labrum is removed, and it further decreases after creating a chondrolabral defect.9 More recently, Halder et al10 reported the average stability ratios with and without the labrum according to the anterior-posterior and superior-inferior directions (Fig. 1-6). These authors reported that the stability ratio was greatest in the inferior direction and was greater with the arm in adduction compared to abduction. The average contribution of the labrum to the stability was 10%. The aforementioned minimally constrained glenohumeral articulation results from the unique and variable anatomy of the proximal humerus, including the humeral head and the glenoid. The following sections describe the bony stabilizers with respect to specific anatomy.
Proximal humerus
The proximal humerus includes an ellipsoidal humeral head covered with hyaline articular cartilage. The anatomic neck consists of a bony transition from cartilage to capsular attachment and tendinous insertion. The tuberosities are located lateral to the anatomic neck. The lesser tuberosity contains the subscapularis insertion, and the greater tuberosity, which has superior, middle, and inferior facets, contains the supraspinatus, infraspinatus, and teres minor insertions.11 The insertions on these tuberosities create an interconnected cuff of tendons that surrounds the humeral head. The transition point to the humeral shaft, just distal to the tuberosities, marks the surgical neck. The bicipital groove is flanked by both tuberosities and provides a stabilizing path for the long head of the biceps tendon (Fig. 1-7).
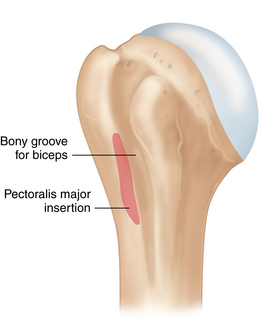
(From Reider B, et al: Operative techniques, sports medicine surgery, Philadelphia, 2010, Saunders.)
The morphologic measurements of the proximal humerus vary greatly due to size variations. Robertson et al12 reported the intramedullary and extramedullary morphology of 30 pairs of proximal humeri. Head retroversion averaged 19 degrees (range, 9 to 31 degrees); head inclination, or neck-shaft angle, averaged 41 degrees (range, 34 to 47 degrees); head radius averaged 23 mm (range, 17 mm to 28 mm); head thickness averaged 19 mm (range, 15 mm to 24 mm); medial head center offset averaged 7 mm (range, 4 mm to 12 mm); and posterior head center offset averaged 2 mm (range, 1 mm to 8 mm) (Fig. 1-8). These extreme variations in proximal humeral anatomy suggest the importance of recognizing the patient specific anatomic geometry of the proximal humerus. For example, restoring the correct prosthetic anatomy during arthroplasty has significant clinical implications for range of motion, kinematics, and impingement.13 Specifically, inferior malpositioning of more than 4 mm can create increased subacromial contact, and an offset of 8 mm in any direction compromises passive range of motion. Minimizing subacromial contact and maximizing glenohumeral motion after humeral head replacement can be achieved with anatomic reconstruction of the humeral head–humeral shaft offset to within 4 mm.
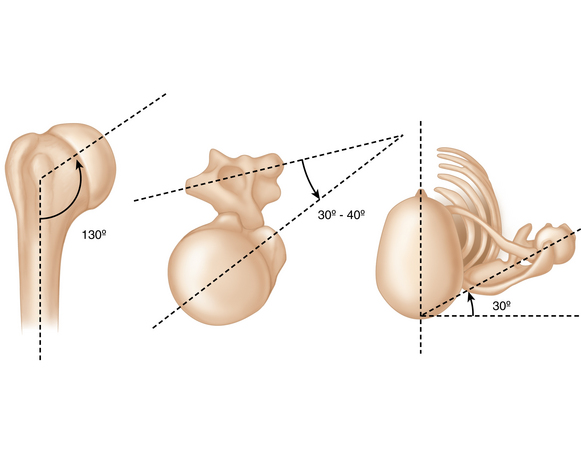
FIGURE 1-8 Drawing of the proximal humerus showing the humeral head inclination and retroversion.
(Redrawn and colorized; original drawing provided by Angie Lee.)
For surgeons, this anatomic variation of proximal humerus must be carefully considered. In order for a surgeon to consistently orient the anatomic geometry of the proximal humerus, the bicipital groove is commonly used during surgery as a bony landmark of the proximal humerus.14,15 In a computed tomography (CT) analysis of embalmed cadavers, the bicipital groove was measured at both the anatomic and surgical necks relative to the transepicondylar axis at the elbow.14,15 As the bicipital groove coursed distally, it was found to internally rotate; the mean change in rotation was 15.9 degrees (SD ± 6.8 degrees; range, 4 to 32 degrees). A similar study found a 9.3 degrees difference (range, 3 to 22.5 degrees) between the bicipital groove at the anatomic neck and the surgical neck.14 The shoulder surgeon must carefully consider this variability whenever the bicipital groove is used as a landmark in humeral head replacement. It is a particularly relevant factor during humeral head replacement involving a three-part or four-part fracture, when the proximal bicipital groove often cannot be used as a reliable anatomic landmark. Placing the lateral fin of the prosthesis either 9 mm or 12 mm posterior to the bicipital groove at the anatomic neck has been recommended.16,17 However, using the bicipital groove at the surgical neck as an anatomic landmark may create excessive retroversion of the humeral head. Therefore, bicipital groove anatomy is only a potential guide when individualizing anatomic restoration during arthroplasty.
The anatomic restoration of the tuberosities is very important for restoring shoulder function. Although the bicipital groove stabilizes the long head of the biceps tendon, the tuberosities that flank the groove determine the rotator cuff length and tension relationships which are responsible for shoulder function. This importance of restoring tuberosity anatomy has been supported by biomechanical studies.18,19 Medial-to-lateral tuberosity displacement in a four-part fracture model altered kinematics and increased the torque necessary to externally rotate the humerus 50 degrees.18 This finding is relevant to the use of the bicipital groove as a landmark for prosthesis placement because after the prosthesis orientation is determined, the tuberosity position is obligatory. In another four-part fracture model, 10 mm of inferior tuberosity placement resulted in significantly more superior glenohumeral force displacement, which suggests a compromise in the mechanical advantage of abduction.19 These studies provide a biomechanical rationale for optimizing the restoration of proximal humeral anatomy during arthroplasty, which can be difficult with a four-part fracture. For anatomic restoration of the proximal humerus, the extreme variability of the osseous proximal humerus anatomy necessitates an individualized anatomic bony restoration approach to create the most favorable environment for soft tissue function.
Glenoid
The glenoid functions as the shallow socket of the glenohumeral joint on the lateral side of the scapular body. The glenoid is pear shaped, with a wider inferior half (Fig. 1-9, A); on average, the size and the shape vary widely. The glenoid is inclined and retroverted by a small amount, again with large variations. The average superior-inferior dimension of the glenoid was reported to be 39 mm (± 3.7 mm; range, 30 mm to 48 mm); the anteroposterior dimension of the lower half of the glenoid averaged 29 mm (± 3.1 mm; 21 mm to 35 mm); and the anteroposterior dimension of the upper half at its midpoint averaged 23 mm (± 2.7 mm; 18 mm to 30 mm). The ratio of the anteroposterior dimension of the lower half of the glenoid to that of the upper half was 1:0.8 (± 0.01).20 The average inclination and the retroversion of the glenoid has been reported to be 4.2 degrees (range −7 to 15.8 degrees; a positive value denotes a superior inclination, and a negative value, an inferior inclination) and 1.23 degrees (± 3.5 degrees; range, 9.5 degrees anteverted to 10.5 degrees retroverted), respectively21 (Figs. 1-9, B–1-9, D). The glenoid anatomy, as with other structures in the shoulder, varies greatly among individuals.
For surgeons, the complex and variable anatomy of the glenoid presents a challenge in the face of pathologic bone loss for reconstruction procedures such as in shoulder arthroplasty. Recently, the effect of glenoid malpositioning on humeral head stability was reported.22 In this study, the glenoid component was retroverted 15 degrees to simulate the posterior glenoid bone loss often seen with osteoarthritis, and modular components were used to test the proximal humeral stem in anatomic and 15 degrees anteversion. The findings showed that because an anteverted humeral component could not stabilize the shoulder or compensate for a severely retroverted glenoid, reaming the glenoid to neutral version might be preferable to altering humeral version. However, it is unknown whether these findings would be altered by the presence of a patulous posterior capsule, which is often encountered in conjunction with posterior glenoid bone loss. More recently, a study using a three-dimensional finite element model of the shoulder concluded that glenoid retroversion greater than 10 degrees can lead to major biomechanical alterations and possibly increase the risk of loosening.23 This was further supported by a cadaveric study.24 Therefore, a surgeon should consider forgoing glenoid resurfacing if retroversion cannot be corrected below 10 degrees (Fig. 1-10).
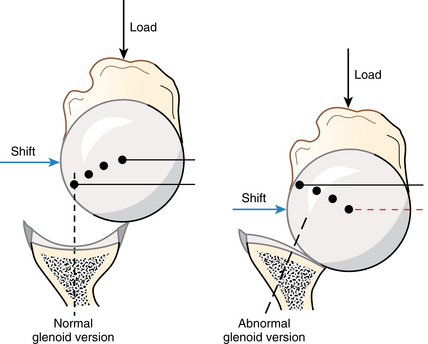
FIGURE 1-10 Glenoid hypoplasia. When the glenoid rim is hypoplastic, it cannot contribute normally to the glenoidogram.
(From Matsen FA, III, et al: Shoulder surgery: Principles and procedure, Philadelphia, 2004, WB Saunders, p 111.)
The pathoanatomy of osteoarthritis can lead to posteroinferior glenoid erosion and wear. Posterior glenoid wear, as shown on transverse-plane imaging, is a recognized measure of pathoanatomy that must be considered when performing arthroplasty.25,26 Glenoid inclination, as seen in the coronal plane, is another useful tool for surgical decision making. Standard radiographs of 100 consecutive patients with primary osteoarthritis were compared with radiographs of 100 otherwise healthy patients with shoulder pain. Inferior glenoid erosion was classified by degree of inclination to represent increasing inferior glenoid wear. In type 0, the mean angle of inclination was 1.7 degrees (range, 7 to 7 degrees); in type I, 7.1 degrees (16 to 2 degrees); in type II, 16 degrees (32 to 5 degrees); and in type III, 17.7 degrees (28 to 12 degrees). These authors recommended normalizing type II and type III glenoid inclination as well as retroversion of more than 15 degrees for glenoid replacement.27 However, excessive medialization should be avoided. For anatomic restoration of the glenoid, again, the extreme variability of the anatomy necessitates an individualized anatomic bony restoration approach to create the most favorable environment for soft tissue function.
Passive soft tissue stabilizers
The passive soft tissue stabilizers include the glenoid labrum, glenohumeral ligaments, and the glenohumeral joint capsule (Figs. 1-11 and 1-12). These soft tissue stabilizers help limit glenohumeral joint motion for both rotation and translation in a position dependent manner. The complex anatomy of each structure accommodates their complex role as a primary stabilizer, as well as a secondary stabilizer, depending on the glenohumeral position. The following sections describe the passive soft tissue stabilizers with respect to specific anatomy.
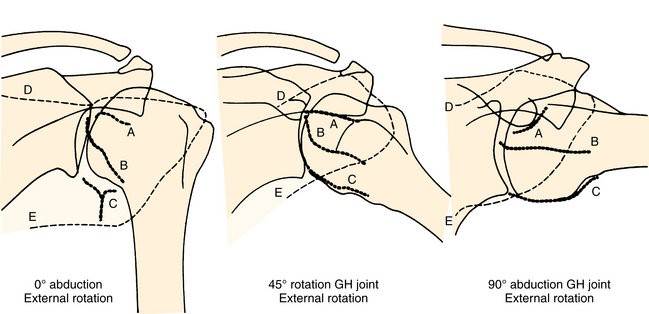
(Modified from Turkel SJ, et al: Stabilizing mechanisms preventing anterior dislocation of the glenohumeral joint. J Bone Joint Surg Am 63:1208–1217, 1981.)
Inferior glenohumeral ligament complex (ighlc)
The inferior glenohumeral ligament (IGHL) is the most important anterior stabilizer in 90° of abduction and external rotation.28 This was corroborated by the strain measurement in the anterior band, which was tight in abduction and external rotation, whereas the posterior band was tight in abduction and internal rotation.29 The anterior band also was reported to be the primary anterior stabilizer when the arm was in abduction in the scapular plane, and the posterior band was the primary posterior stabilizer when the arm was horizontally adducted.30
Anatomically, the IGHL is attached to the glenoid in the 7-to-9 or 3-to-5 clock-face positions. The collagen fibers are directly affixed to the rigid fibrocartilaginous labrum and more medially to the periosteum along the glenoid neck. The anterior band is an anterior thickening of the IGHL. A posterior capsular thickening or band is present in 63% of specimens.31 The IGHL attachment on the glenoid side has been reported to have two types of insertions32 (Fig. 1-13). In this study of 10 shoulders, 2 out of 10 specimens had labral dominant insertion where the IGHL is directly attached to the labrum, and 8 out of 10 specimens had glenoid neck dominant insertion where the IGHL is attached to both the labrum and the glenoid neck. These two insertion types may influence arthroscopic repair of Bankart lesion as the lesion on the glenoid neck cannot be visualized during an arthroscopic repair.
The IGHL attachment on the humeral side has two attachment geometries: the split type (58%) and the broad type (42%).33 Both extend inferiorly 2 cm from the articular surface at approximately the 4 o’clock and 8 o’clock positions. The split type is a capsular bifurcation having internal and external folds with an interposed loose connective tissue. The broad type does not have separate folds. Failure to release the external fold may tether the capsular pouch in a patient with multidirectional instability. Releasing the entire capsule from the humeral attachment, particularly posteriorly, may be necessary during a capsulorrhaphy.
The biomechanical characteristics of the three IGHLC anatomic regions (superior band, anterior axillary pouch, and posterior axillary pouch) were quantified by tensile testing of bone-ligament-bone complexes highlighting the significance of permanent stretching of the capsule.34 However, in an isolated Bankart lesion, only minor humeral head translation was observed,35 suggesting that IGHLC plastic deformations exist for pathologic recurrent instability. Another study reported unrecoverable IGHLC strain as a result of cyclic loading and thereby supported the hypothesis that repeated subfailure strain results in an overuse injury.36 Testing of IGHLC in the apprehension position (abduction and external rotation) showed that unrecoverable capsular elongation was greatest in the ligament midsubstance (mean, 0.53 mm) and smallest at the humeral insertion (mean, 0.04 mm).37 The low absolute strain values suggest that only slight plication of the anteroinferior capsulolabral structures may be necessary to restore capsular anatomy after a primary instability injury (Figs. 1-14 and 1-15)
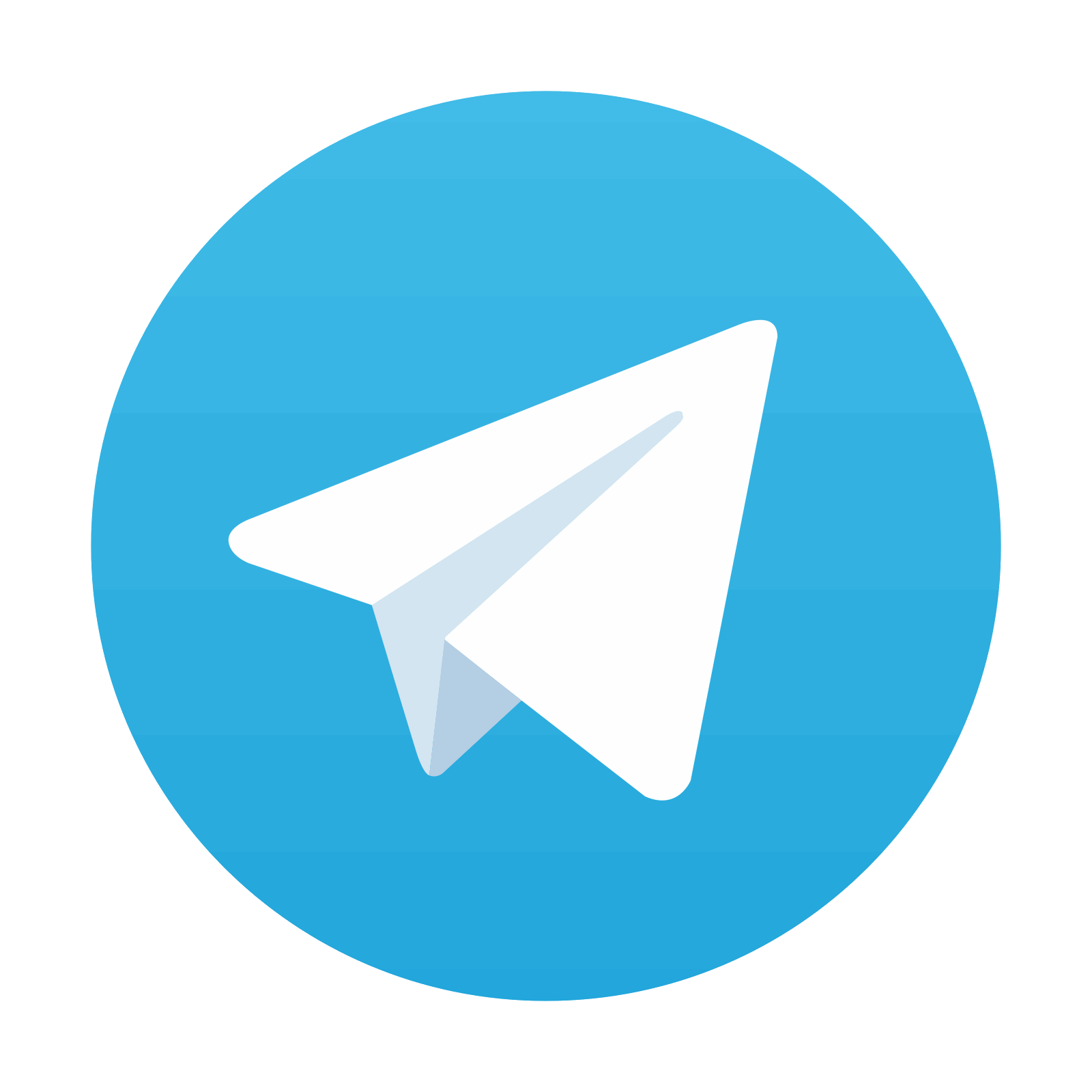
Stay updated, free articles. Join our Telegram channel

Full access? Get Clinical Tree
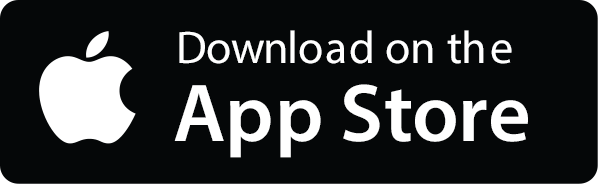
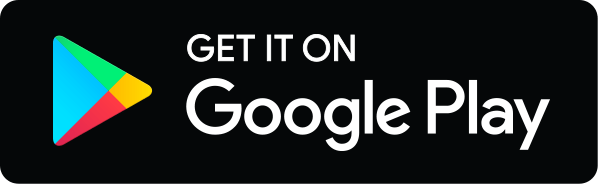