Chapter 12
Cardiovascular fitness training
Poor cardiovascular fitness is an impairment which commonly prevents patients with spinal cord injury from performing motor tasks.1–4 The performance of motor tasks with paralysis is often an inefficient way of moving5 and is physically demanding.6,7 For example, ambulating with partial paralysis of the lower limbs is associated with a greater oxygen cost than walking as an able-bodied individual.8 Similarly, pushing a wheelchair up a slope is physically strenuous. The added physical stress of moving with paralysis is particularly pronounced in the early days after injury when patients have not yet mastered efficient ways of moving.1 At this time, patients may also be deconditioned from extended periods of bedrest9 and may still be recovering from the effects of associated chest or lung injuries.
Cardiovascular fitness is also important for good long-term health and quality of life.2,10–12 Poor cardiovascular fitness predisposes patients to cardiovascular disease, a leading cause of death in patients with spinal cord injury.13–16 Seventeen per cent of patients with established spinal cord injury develop ischaemic heart disease (compared to 7% in the general community)13 and 50% die of cardiovascular-related diseases.17 The high rate of cardiovascular-related disease is due to sedentary lifestyles18 and the high incidence of obesity,19,20 glucose intolerance,21 diabetes22 and smoking.10,23–26 It is also due to deleterious changes in lipid profiles including decreases in the concentration of high density lipoprotein-cholesterol (this is the type of cholesterol which helps prevent cardiovascular disease).19,22,27 Regular and ongoing exercise decreases the incidence of cardiovascular-related disease in the able-bodied population and is believed to be equally important for patients with spinal cord injury.5,23 Patients are probably most likely to regularly exercise in the community if fitness-training programmes are an integral part of the rehabilitation process and if patients have access to appropriate opportunities and facilities.
Fitness-training programmes need to be based on appropriate exercise testing and prescription. Both pose unique problems in people with spinal cord injury, especially those with high thoracic and cervical lesions. These patients have marked or complete loss of supraspinal sympathetic control (see Chapter 1). The sympathetic nerves innervate the smooth muscles of arteries, veins and airways, as well as the heart and adrenal medullae.4 They are important for cardiac, respiratory, thermoregulatory and metabolic responses to exercise.20 Circulating catecholamines can mimic sympathetic activity but their effects are delayed and often less pronounced.4,28
There are still many unanswered questions about different aspects of cardiovascular fitness training and testing for patients with spinal cord injury24,39 and few randomized controlled trials to provide definitive answers.12,29–32 Most of what is known comes from quasi-experimental trials,33,34 cross-sectional studies,35–37 knowledge about the short-term effects of exercise in patients with spinal cord injury, and the generic benefits of exercise for other patient and able-bodied populations.6,11,35–39 The important trial examining the effectiveness of cardiovascular fitness training for preventing cardiovascular-related disease in patients with spinal cord injury is yet to be done. With these limitations in mind, the purpose of this chapter is to outline the effects of spinal cord injury on patients’ responses to exercise and to provide guidelines for exercise testing and prescription. This chapter focuses on patients who are wheelchair-dependent with sufficient upper limb strength to actively exercise (i.e. patients with lesions between C5 and T12). However, regular exercise is also important for ambulating patients and the underlying principles of exercise testing and training are the same.
Assessment of cardiovascular fitness
There are three main ways to assess cardiovascular fitness.40 Each is summarized below.
Peak oxygen consumption tests
The most accurate way to assess cardiovascular fitness is with a peak oxygen consumption () test.8,20,41 The
test measures the maximal capacity of the body to deliver oxygen from the lungs to the mitochondria of exercising muscles.42 The test can be performed with any type of exercise, although ideally with exercise incorporating as much available muscle mass as possible. It is typically performed with patients rotating arm ergometers, or propelling wheelchairs on treadmills or ergometers.20,40,43,44 Expired gases are collected during the test through a mouthpiece connected to a gas analysis system.45,46 Results are expressed as either the greatest absolute (l.min‒1) or relative (ml.kg‒1.min‒1) rate of oxygen consumption.46
The test requires patients to exercise at gradually increasing intensities until exhaustion. Short rests of between 20 and 30 seconds are sometimes provided between each increment.11,47,48 For example, an arm ergometer test in a patient with paraplegia might start at 30 watts (W) and then increase by 10–15W every 2 minutes.11,49,50 The maximal power output these patients are likely to achieve is between 50W and 100W. The equivalent test for a patient with tetraplegia might start at 5W or less, and increase by between 2.5W and 10W, depending on the level of fitness and spinal cord injury. The maximal power output a patient with tetraplegia is likely to attain ranges from 10W to 50W.45 The power output of an arm ergometer can be adjusted by changing the externally applied resistance and cranking velocity. Cranking velocities between 30 and 90rpm are commonly used.
The test is equivalent to the
test in able-bodied people. The different terminology is used to reflect the lower maximal rate of oxygen consumption with arm versus leg exercise.11 Arm exercise is associated with a lower maximal rate of oxygen consumption because of the lower demand for oxygen from the smaller exercising upper limb muscles and the circulatory implications of arm exercise (see pp. 231–234 for details).
Submaximal exercise tests
Cardiovascular fitness is most commonly assessed in wheelchair-dependent patients with submaximal arm tests. Expired gases can be collected with portable and easy-to-use expired gas analysis systems although it is more common in spinal cord injury units to just measure heart rate.41 Submaximal arm tests are performed in a similar way to maximal arm tests but are terminated before exhaustion. Different testing protocols are used. A commonly used protocol includes three 7-minute bouts of exercise at 40%, 60% and 80% of predicted maximal exercise capacity. For example, a patient with paraplegia and a high level of fitness might exercise at 40W, 60W and 80W. Patients with lower levels of cardiovascular fitness and patients with tetraplegia would exercise at three lower power outputs (e.g. 20W, 30W and 40W).
In able-bodied individuals, submaximal tests with expired gas analysis are used to estimate . Estimations are based on the assumption that there is a linear relationship between oxygen consumption and heart rate.46,55,56 Oxygen consumption data are extrapolated to the point which corresponds with predicted maximal heart rate. The same process can be used to predict maximal power output because there is also a linear relationship between oxygen consumption and power output.45 It is, however, more difficulty to estimate
from submaximal arms tests, especially in patients with spinal cord injury and loss of supraspinal sympathetic control.55 Formulae for predicting
from the results of submaximal tests have been proposed but are yet to be validated.11,43,57,58
The results of submaximal tests which solely rely on heart rate are primarily used to monitor the response of patients to training.51 For example, improvements in cardiovascular fitness are indicated by a decrease in heart rate at the same power output with training. It is also possible to gauge improvements in fitness by patients’ perceptions of exertion. The Borg exertion scale is widely used for this purpose (see Table 12.1).41,52–54 Improvements in fitness are indicated by lower levels of perceived exertion with exercise at the same power output.
Table 12.1
6 | no exertion at all |
7 | extremely light |
8 | |
9 | very light |
10 | |
11 | light |
12 | |
13 | somewhat hard |
14 | |
15 | hard (heavy) |
16 | |
17 | very hard |
18 | |
19 | extremely hard |
20 | maximal exertion |
After References 52 and 53 with permission of Borg Products USA, Inc.
Field exercise tests
Cardiovascular fitness can also be assessed by measuring the distance walked, pushed, cycled or swam over a set time period.51 Alternatively, instead of measuring the distance covered in a set time, the distance can be standardized and the time taken to cover the distance measured. The more standardized tests include the 6- and 12-minute wheelchair propulsion tests.3,59,60 In these tests, patients are required to push their wheelchairs as far and as fast as possible in 6 or 12 minutes over flat ground. Variations can be used where the speed of pushing and/or incline are gradually increased.61 can be estimated from the 12-minute wheelchair propulsion test (see Table 12.2).59,60
Table 12.2
Guidelines to estimating fitness from distance pushed in a manual wheelchair over 12 minutes
Fitness level | Distance (km) | ![]() |
Poor | < 1 | < 7.7 |
Below average | 1–1.39 | 7.7–4.5 |
Fair | 1.4–2.1 | 14.6–29.1 |
Good | 2.2–2.5 | 29.2–36.2 |
Excellent | > 2.5 | > 36.3 |
Reprinted from Archives of Physical Medicine and Rehabilitation, Vol 71, Franklin BA, Swaantek KI, Grais SL et al, Field test estimation of maximal oxygen consumption in wheelchair users, pp 574–578. Copyright 1990, with permission from the American Congress of Rehabilitation Medicine and the American Academy of Physical Medicine and Rehabilitation.
The response of people with spinal cord injury to exercise
The response of patients with spinal cord injury to exercise is influenced by the extent and level of neurological involvement. Patients with the ability to ambulate respond to exercise in a similar way as their able-bodied counterparts. In contrast, the response to exercise of wheelchair-dependent patients with complete upper thoracic paraplegia or tetraplegia is quite different. Their exercise response is adversely affected by three main factors, namely, reliance on arm exercise, lower limb paralysis and loss of supraspinal sympathetic nervous control.4,20 Each of these three factors adversely effect cardiac output and arterio-venous oxygen difference (a- difference): the two determinants of
(see Table 12.3).
Table 12.3
Key determinants of heart rate, stroke volume and a- difference
Heart rate | Stroke volume | a-![]() |
Sympathetic nervous system Parasympathetic nervous system Circulating noradrenalin Intrinsic heart rhythm | Venous return After-load Contractility Blood volume | Size of exercising muscle mass Ability of muscles to extract oxygen: |

where: cardiac output = heart rate × stroke volume.
Cardiac output
Maximal cardiac output is reduced in patients with spinal cord injury. This is predominantly due to a decrease in maximal stroke volume but also due to a decrease in maximal heart rate.14,48
Fitness training typically increases maximal cardiac output in able-bodied individuals and patients with lower levels of spinal cord injury capable of exercising with larger muscle masses. However, in wheelchair-dependent patients with spinal cord injury, and especially in those with tetraplegia, arm exercise does not commonly place a sufficient demand on the heart to prompt a central training effect on cardiac output unless patients are very deconditioned.48 The exercising muscles are too small and the body’s demand for oxygen too low to stress and hence train the heart.4,5,62,63
Heart rate
The balance between sympathetic and parasympathetic activity determines heart rate. Sympathetic control to the heart is via T1–T4 nerve roots, while parasympathetic control is via the vagal nerve.20,48 Sympathetic activity increases and parasympathetic activity decreases heart rate.4,20,48 Without input from either source, the heart will beat at approximately 70–80 beats.min21. This is due to the intrinsic firing rate of the sinoatrial node in the heart.
Patients with lesions above T1 have complete loss of supraspinal sympathetic control to the heart. Consequently, heart rate is primarily increased by the withdrawal of excitatory input from the vagal nerve.4,20,64 Circulating humoral factors such as catecholamines can further increase heart rate65,66 but there is a time lag between their release and effect. The maximal heart rate of patients with lesions above T1 can be as low as 110–130 beats.min‒1.4,49 In contrast, the maximal heart rate of able-bodied individuals is approximately 200 beats.min‒1 depending on age.
Unlike able-bodied individuals, fitness training may increase maximal heart rate in patients with spinal cord injury.41 The mechanisms underlying this possible training effect are not well understood, but may be due to changes in the body’s ability to release and respond to circulating humoral factors. Alternatively, it may be due to the local effects of training on the arm muscles. Training delays the onset of muscle fatigue during maximal exercise testing. Consequently, trained patients can exert themselves more than untrained patients placing a greater demand on the body for oxygen. This demand is met by an increase in heart rate.41
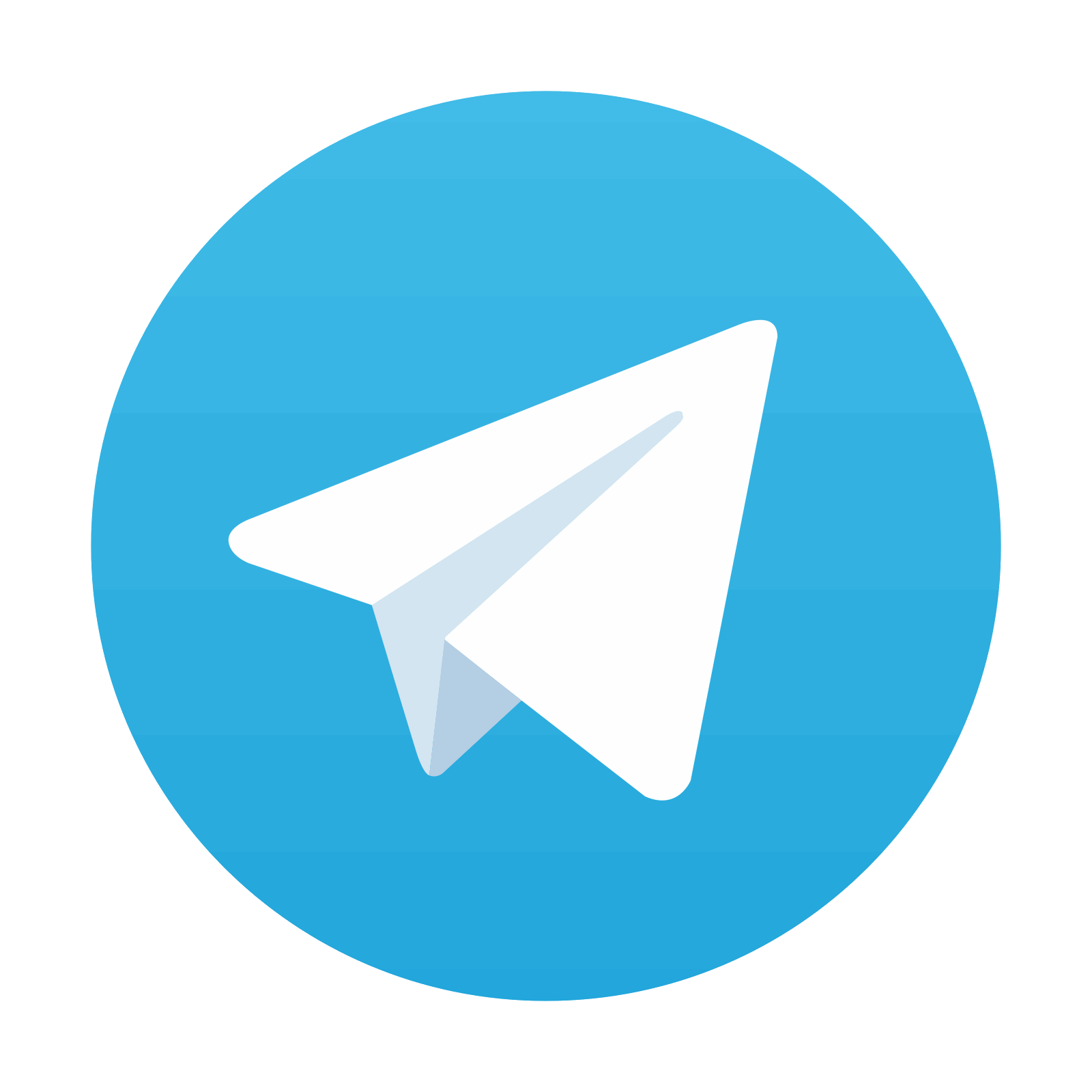
Stay updated, free articles. Join our Telegram channel

Full access? Get Clinical Tree
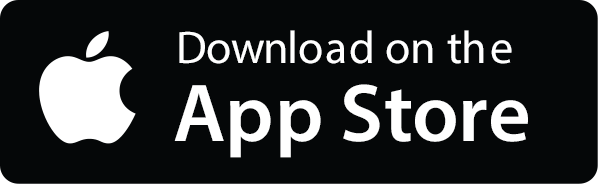
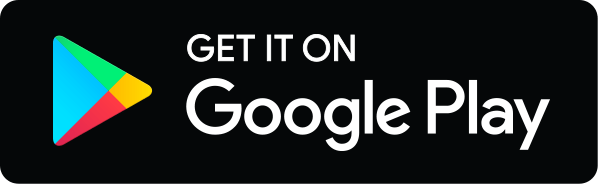