CHAPTER 38 Bone Biology
GENERAL PRINCIPLES
Organization of bone
Macroscopically, the skeleton is composed of flat bones (e.g. bones of the skull) and long bones (e.g. tibia), based on development and growth occurring through an intramembraneous process or endochondral and intramembraneous processes, respectively.1 From a gross anatomical perspective, individual bones are made up of varying proportions of cortical (compact) and trabecular (cancellous) tissue, depending on location, and reflecting specific structural and functional differences. Compact bone is 80–90% mineralized tissue by volume, whereas trabecular bone is only 15–25% mineralized. Thus, trabecular bone has a much larger interface with soft tissue such as bone marrow, vascular, and connective tissue. In general terms, skeletal specialization is dictated by the relative abundance of cortical and trabecular tissue within specific bones; that is, cortical tissue accounts mainly for mechanical and protective functions while metabolic and cellular renewal functions are attributed mostly to trabecular tissue.
Cellular elements
Osteoclasts are giant multinucleated cells whose precursors are bone marrow monocytes/macrophages derived from hematopoietic stem cells and whose sole function is bone resorption.2 They are typically located in contact with a calcified bone surface within Howship’s lacunae. Their so-called zone of contact with bone is a ruffled membrane bounded circumferentially by an actin ring that serves to seal off the underlying bone as a discrete resorbing compartment. Specific attachment of osteoclasts to matrix components occurs through αvβ3, αvβ5, and α2β1 integrin receptors. The ruffled membrane contains both a proton ATPase and coupled chloride channel that acidifies the sealed-off bone resorbing compartment while maintaining electrical neutrality across the membrane. This is made possible by carbonic anhydrase within the cell and a HCO3−/Cl− exchanger on the basolateral membrane. RANK, the receptor for RANKL, and the macrophage-colony stimulating factor receptor are also located on the basolateral membrane, both being required for osteoclast differentiation.
Ossification in the spine
As in long bones, ossification in the spine occurs through an endochondral process.3 Mesenchymal cells give rise to prechondroblasts which then become progressively embedded within their cartilaginous matrix in lacunae. Now designated as chondrocytes, they continue to proliferate and then enlarge progressively to become hypertrophic, eventually undergoing apoptotic cell death. As cartilage cells die, and presumably under the influence of vascular endothelial growth factor (VEGF), the area is invaded by blood vessels. Osteoblasts move onto the cartilage surface and deposit bone in this area of primary spongiosa.
VERTEBRAL MORPHOGENESIS AND EMBRYOLOGICAL DEVELOPMENT
Cartilage models
Neural crest cells contribute to the branchial arch derivatives of the craniofacial skeleton, and the lateral plate mesoderm gives rise to the limbs. However, it is the paraxial mesoderm that gives rise to most of the axial skeleton, including the vertebral bodies, as well as the nonbranchial arch derivatives of the craniofacial skeleton. Mesenchymal cells condense to form regions with positional identity, which ultimately represent the future skeletal outline. The fate of mesenchymal cells within condensations dictates whether ossification occurs via an intramembranous or endochondral process. The former occurs as the result of osteoblast differentiation in the calvarium, maxilla, mandible, and subperiosteal bone-forming region of long bones. It is mediated by the transcription factors CBFA1/RUNX2 and osterix (OSX).4–7 Endochondral ossification requires differentiation of mesenchymal cells into chondrocytes, creating cartilage models of the remaining skeleton that serve as templates for bony replacement. Chondrocyte differentiation is influenced by members of the SOX-family of transcription factors.5–8
Cartilage models develop by interstitial and appositional growth until they take on the characteristic shapes and sizes of those intended bones. Patterning of the endochronal skeleton occurs even in the absence of eventual bone formation. Regulation of cartilage differentiation and growth in proper spatial sequence is essentially sufficient to complete a correctly shaped skeleton and this concept is supported by the phenotype of mice harboring two null alleles for the gene encoding CBFA1/RUNX2.5,6 Despite the lack of osteoblast differentiation in these mice, a nearly intact skeleton is formed with accurately placed and correctly shaped skeletal elements, albeit consisting totally of cartilage.
MESENCHYMAL CONDENSATION AND CHONDROCYTE DIFFERENTIATION
The regulation of events leading up to the formation of somites is thought to be tightly controlled by cell–cell interactions involving the Notch1 receptor and its ligands, which are also transmembrane molecules.9,10 Vertebral defects in mice can be seen as the result of somite condensation and patterning errors when the gene for Notch1 or one of its ligands is inactivated.8,11–13 Mutations in specific Notch1 ligands also occur in humans and are associated with the vertebral abnormalities found in the recessive form of spondylocostal dysostosis (semivertebrae) and Alagille syndrome (butterfly vertebrae).14–16
Disruption of sclerotome cell differentiation, and thus chondrocyte differentiation, also has profound effects on vertebral column formation. The notochord-secreted cytokine, Sonic hedgehog, is an important regulator of sclerotome cell differentiation. Its inactivation in mice leads to skeletal development without a vertebral column and posterior rib regions.17,18 Spinal deformities in mice and humans have also been reported to occur with mutations in PAX1, a transcription factor thought to be at least partly inducible by Sonic hedgehog.19,20
OSTEOBLAST DIFFERENTIATION AND BONE FORMATION
Regulatory pathways
Transcriptional control mechanisms regulate osteoblastogenesis. Examples of early transcriptional regulators include the homeodomain proteins (e.g. Msx-2, Dlx-2, Dlx-5, BAPX1), steroid receptors, as well as the helix-loop-helix (HLH) proteins Id, Twist, and Dermo. The HLH proteins play important roles in the proliferation of osteoprogenitor cells, but repress osteoblast differentiation and must be down-regulated before a mature bone cell phenotype can be expressed.21 The activating protein cFOS is also expressed in osteoprogenitor cells, resulting in osteosarcomas if overproduced, but is not detected in mature osteoblasts.22 A similar theme is true for some of the homeodomain transcription factors, where mesenchymal precursors abundantly express them, but mature bone-forming cells do not.23,24
In contrast, the runt homology domain proteins (e.g. Runx2/Cbfa1) and the zinc finger protein Osterix and others activate genes expressed in the mature osteoblast, such as osteocalcin and osteopontin (Fig. 38.1).6,7,25–27 Null mouse mutants of Runx2 and Osterix result in inhibition of bone formation and perinatal mortality, demonstrating their requirement in osteogenesis.6,7 Runx2 also plays important roles in the maturation of chondrocytes, cartilage mineralization, and endothelial cell migration necessary for vascular invasion.28–30 Runx2 mediates its plethora of effects by interaction with diverse coregulatory proteins, including CBFβ1, Wnt pathway regulator LEF-1, and TGF-β/BMP responsive SMAD proteins, to name a few.31–38 Temporal expression of Runx2 followed by Osterix is thought to be essential for the later stages of osteoblast differentiation.7
Hormonal regulation of osteoblast differentiation includes steroid as well as polypeptide signaling molecules. Glucocorticoids enhance the differentiation of bone marrow stromal cells to osteoblasts in vitro, but in vivo have negative effects on bone that contribute to osteoporosis by inducing apoptotic cell death.39–41 Vitamin D3, sex steroids, adrenomedullin, and leptin all have osteogenic or anabolic effects on bone.42–50 Retinoic acid contributes to skeletal development in the embryo and also plays a role in postnatal bone formation.51–53 Among the polypeptide hormones, PTH and PTHrP have stimulatory effects on osteoprogenitor cells and regulatory effects on differentiation, both mediated by coupled G protein interactions with their receptors.54–61
Osteoprogenitor cells
Osteoprogenitor cells can arise from stem cells in a variety of tissues. There are as yet no unique identifying markers for the mesenchymal stem cell (MSC) that gives rise to bone, fat, cartilage, and muscle. Bone marrow stroma contains highly proliferative cells that will form single colonies or colony-forming units-fibroblasts (CFU-Fs) and are thought to contain mesenchymal stem cells that can be distinguished from early hematopoietic precursors.62–65 Stromal cells grown in vitro are heterogeneous with respect to the capacity for differentiation, and only a low percentage of all CFU-Fs have stem cell properties.62,63,66,67 Further, only a small fraction of CFU-Fs are bone-forming cells.68,69 Although a specific osteoprogenitor marker has not been identified, antibodies have been developed (e.g. STRO-1, SP-10, SH2, HOP-26) that recognize some subsets of precursor cells.70–77
Mesenchymal differentiation may proceed by a multistep hierarchical process with greater cell lineage restriction to terminal cell types at each subsequent step.78 This idea is supported by identification of bipotential adipocyte-osteoblast precursors, and has suggested that there may be an inverse relationship between adipocyte and osteoblast differentiation.79,80 A related observation is the transdifferentiation of bone to fat cells, or fat to bone cells.81–84 Depending on the local cellular environment, ‘committed’ MSC progeny may also dedifferentiate into another lineage.85 Thus, plasticity may be a common phenomenon; however, at least some reports suggest that cell fusion events may confound this paradigm.86–90 Figure 38.1 shows a simplified scheme for osteoblast differentiation.
MSCs similar to those found in bone marrow have been found in adult peripheral blood, fetal cord blood, fetal liver, tooth pulp, muscle satellite cells, and extramedullary adipose tissue and have osteogenic potential.91–100 Multipotent adult progenitor cells (MAPCs) derived from bone marrow can contribute to most somatic cell types, including skeletal tissue.101,102 Pericytes can also be induced toward the osteogenic lineage.103
Osteogenic growth factors
Bone morphogenetic proteins (BMPs), members of the transforming growth factor β (TGF-β) superfamily, are produced by skeletal and extraskeletal tissues. BMP-2, −4, and −6 are secreted by osteoblasts and play an autocrine role by enhancing the differentiated function of bone-forming cells.104 BMPs induce the differentiation of cells toward the osteoblast lineage, increase the terminally differentiated pool of osteoblasts, and induce endochondral ossification and cartilage formation in concert with Indian/Sonic hedgehog.105–107 BMPs interact with three distinct serine/threonine kinase receptors which, after dimerization, can signal through at least eight different Smads that become phosphorylated and/or heterodimerize to regulate transcription.108–110 Smads have activating and inhibitory functions, and also mediate signals initiated through TGF-β and activin receptors. BMPs and TGF-β signaling may also use Smad-independent pathways that rely on Ras or MAP kinase.111 Suppression of BMP activity occurs at several levels, including nonsignaling pseudoreceptors, inhibitory Smads, Smurf-mediated ubiquitination and degradation of signaling Smads, intracellular Smad binding proteins, and extracellular BMP antagonists such as noggin, chordin, follistatin, and twisted gastrulation.112
TGF-β1, -β2, and -β3 are polypeptides synthesized by bone cells and function as mitogens for preosteoblasts, stimulatory factors for collagen synthesis, and inducers of osteoclast apoptosis.113 The effect of BMPs on osteoblast differentiation is inhibited by TGF-β, and transgenic mice overproducing TGF-β are, in fact, osteopenic.114,115 Like BMPs, TGF-β signals through distinct serine-threonine kinase receptors that activate specific Smads to regulate transcription.110,111
Insulin-like growth factors (IGFs) are produced in a variety of tissues and have been characterized as IGF-I and IGF-II, both sharing similar properties but the former being more potent. IGFs116 stimulate proliferation of the osteoblastic lineage, prevent apoptosis of mature osteoblasts, and enhance net type I collagen abundance by stimulation of transcription and inhibition of collagen protein degradation. IGF-I knockout mice have decreased bone formation while those overexpressing IGF-I have enhanced bone formation.117,118 In humans, IGF-I has a generalized anabolic effect on musculoskeletal tissue with stimulation of bone remodeling.119 Parathyroid hormone (PTH) induces IGF-I synthesis in osteoblasts, and the anabolic effects of PTH on bone is diminished in the absence of IGF-I.117 Steroids decrease IGF-I expression and this may, in part, explain glucocorticoid-induced bone loss.120
β-catenin in association with specific transcription factors can regulate BMP and TGF-β signaling.121 The so-called Wnts prevent the degradation of β-catenin. Low-density lipoprotein receptor-related protein 5 (LPR5) is a coreceptor for Wnt that is required for optimal signaling with resultant accumulation of β-catenin.122 BMP-stimulated osteoblasts and stromal cells express LRP5 and knockout mice lacking LRP5 are osteopenic.123 In humans, inactivating mutations in LRP5 cause decreased bone mass, while other mutations in LRP5 cause a high bone mass phenotype.124,125
Fibroblast growth factor (FGF) 1 and 2 stimulate osteoblast proliferation and in vivo are important in the maintenance of bone mass. FGF null mice display low numbers of osteoblasts and decreased bone formation.126 Conversely, systemic FGF2 increases the preosteoblast pool that ultimately forms mature bone.127 FGF receptor signaling is essential for chondrogenesis and normal skeletal and limb development, with an activating mutation in FGF receptor-3 causing achondroplasia associated with dwarfism and early closure of cranial sutures.128 Platelet-derived growth factor (PDGF) has activities similar to those of FGF.129
Synthesis of bony matrix
In early bone formation, a chondroitin sulfate proteoglycan (versican) and the glycosaminoglycan hyaluronan are secreted and may delineate areas of future bone deposition.130 Versican is eventually replaced by two chondroitin sulfate proteoglycans, decorin and biglycan, each containing leucine-rich tandem repeat sequences. One possible function for these leucine-rich repeat proteins is their ability to bind and regulate TGF-β family members in the extracellular milieu.130 Biglycan-deficient transgenic mice have underdeveloped trabecular bone.131
The most abundant noncollagenous glycoprotein secreted by bone cells is osteonectin, with putative functions in osteoblast proliferation and matrix mineralization. Other glycoproteins mediate cell attachment and have been designated members of the SIBLING family (e.g. fibronectin, osteopontin, bone sialoprotein).132 All members contain the cell consensus sequence RGD (Arg-Gly-Asn) that binds to integrin cell surface molecules. Although most members of the SIBLING family are found in other tissues, bone sialoprotein is specific to mineralized tissue.133
The bone-specific gla-containing protein osteocalcin may function as an inhibitor of mineral deposition, as judged by osteocalcin-deficient mice who have increased bone mineral density.134 Similarly, mice deficient in the matrix gla protein (MGP), found in many connective tissues, develop extracellular sites of calcification.135
Bone mineral contains numerous impurities and is a carbonate-substituted hydroxyapatite with solubility properties that enable its constituent imperfect crystals to serve as a reservoir for calcium, phosphate, and magnesium ions.135 While the mineral content provides mechanical rigidity and load-bearing strength, the organic matrix provides elasticity, flexibility, and microstructural organization largely secondary to the presence of type I collagen.135
Extracellular matrix vesicles released from chondrocytes and osteoblasts accumulate calcium and phosphate ions, enzymes that degrade inhibitors of mineralization, and components of a nucleation core that induce apatite formation.133 It is unclear if there is an association of vesicle mineral with mineral in the collagen matrix or if the matrix vesicle directly provides a calcium and phosphate reservoir that supports mineralization initiated in collagen fibrils. In either case, the first stable crystal formed is followed by the addition of ions and ion clusters that allows for the expansion of crystal structure with addition of secondary nucleation sites.136 Removal of noncollagenous protein from matrix, particularly phosphoprotein, inhibits crystal nucleation and apatite formation.137 Alkaline phosphatase may contribute to enhanced mineralization by increasing the local phosphate concentration, removing phosphate-containing inhibitors of apatite growth, or modifying phosphoproteins to control their function as potential nucleators.133
Growth of mineral crystals is primarily dictated by the collagen matrix template. Noncollagenous proteins that bind crystals can also influence the extent and direction of mineral deposition.133 The presence of ions other than calcium and phosphate may influence bone apatite crystal growth, size, and solubility, and they include magnesium, strontium, cadmium, carbonate, and fluoride.133
MECHANISMS OF BONE RESORPTION
Osteoclast differentiation
Cells of the mononuclear/phagocytic lineage give rise to osteoclasts. Early precursors are committed to the myeloid series by the action of transcription factors PU-1 and MiTf. The lineage is further narrowed to monocytic cells by macrophage-colony stimulating factor (M-CSF).2,138 Proliferation and survival of monocytic cells, with concomitant RANK receptor expression, is mediated by M-CSF. The presence of RANKL is then required for commitment to the osteoclast differentiation program. Osteoclastogenesis depends on the association of M-CSF and RANKL, found on stromal cells and osteoblasts, with their receptors on monocytes/macrophage cells.2,138 Subsequent fusions of preosteoclasts result in the formation of giant multinucleated cells containing 4–20 nuclei and expressing characteristic markers of mature osteoclasts.
The osteoclast membrane has lost several macrophage markers and is lacking Fc and C3 receptors, but retains phagocytic non-specific esterases, lysozyme synthetic function, and CSF-1 receptors.2,138 Osteoclasts are distinguishable from macrophages by the abundance of RANK, vitronectin (integrin αvβ3) receptors, and calcitonin receptors. Osteoclasts undergo an estrogen-promoted apoptotic cell death after a cycle of resorption.2,138
Regulation of osteoclast activity
The key regulators of osteoclastic bone resorption are RANK ligand and its two confirmed receptors, RANK and osteoprotegerin (OPG). OPG is a member of the TNF receptor superfamily that lacks a transmembrane domain and, as such, is a secreted molecule. OPG regulates osteoclast activity by serving as a decoy receptor for RANKL.138–141 The only other known ligand for OPG is TRAIL which, like RANKL, is also a type II membrane-bound TNF homolog. The significance of OPG–TRAIL interaction is unknown. Deficiency of OPG in mice causes increased osteoclast formation, activity, and survival that results in destruction of growth plates, loss of trabeculae, decreased bone strength, and reduced bone mineral density (BMD).142,143 Parenteral administration of OPG causes a decrease in the number of active osteoclasts with a concomitant increase in BMD.144 In vitro, OPG abrogates osteoclast formation mediated by M-CSF and RANKL, in the absence of osteoblasts or stromal cells.139,140 OPG also inhibits osteoclastogenesis induced by a diverse group of osteotropic hormones.138–141 Finally, there is a direct effect of OPG on isolated mature osteoclasts due, in part, to limiting osteoclast survival.
RANKL expression is obligatory for osteoclastic resorption and physiologic bone remodeling (Fig. 38.2). Membrane-bound RANKL is more potent than soluble forms and mature osteoblasts express RANKL constitutively.145–147 Knockout mice lacking RANKL are devoid of osteoclasts and exhibit osteopetrosis with occlusion of the marrow cavity.148 The bone-resorbing activity and survival of mature osteoclasts is greatly increased by exposure to recombinant RANKL in the absence of stromal cells or osteoblasts.138–141
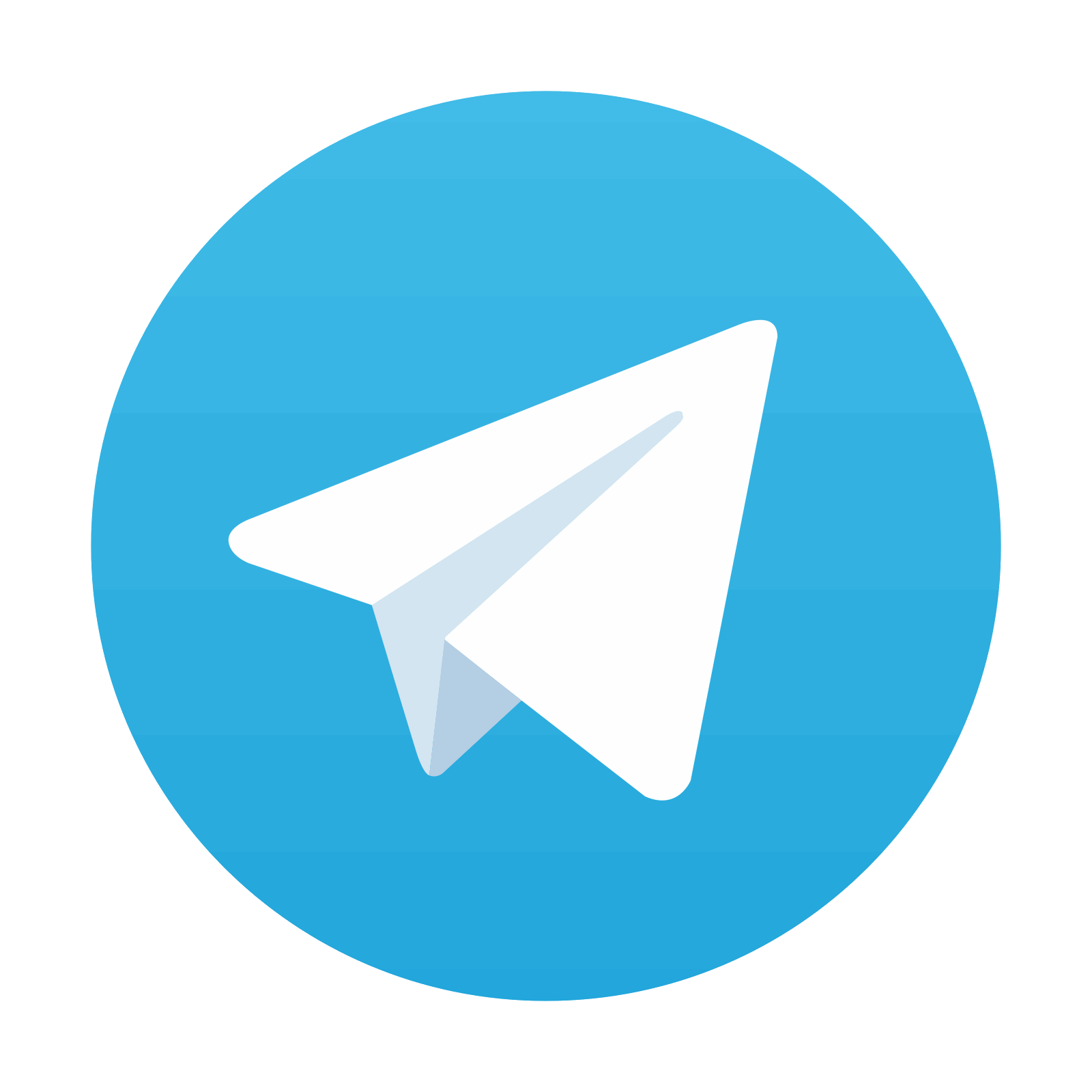
Stay updated, free articles. Join our Telegram channel

Full access? Get Clinical Tree
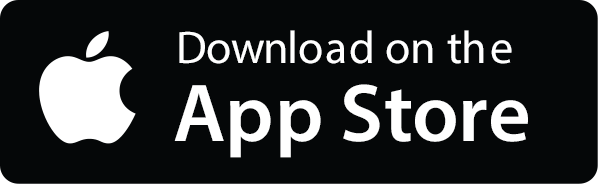
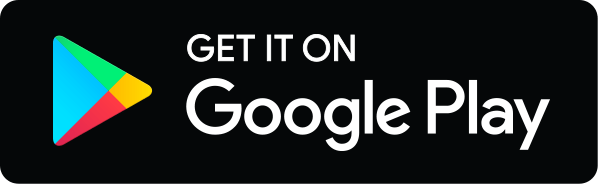