Fig. 18.1
Microscopic appearance of cancellous bone and bone marrow. BM bone marrow, CB cancellous bone
Cancellous Bone
Bone tissue is formed in a place which does not contain any prior bone tissues. Embryos, bone growth, fracture callus, heterotopic ossification, Paget’s bone, and the bone which is generated by tumors can be given as an example. Collagen and minerals are located randomly. Osteocyte organization is not clear. Cancellous bone remodels with removal of primary bone tissue and replacement with secondary bone formation. This formed new bone tissue is named as lamellar bone [5].
Lamellar Bone
This tissue is well organized with lamellar layers in contrast to irregular cancellous bone tissue. Lamellas are 3–7 μ thick layer that can be seen with polarized light microscopy. The layered appearance is not understood exactly; it is thought to be related to configuration of collagen fibers and extracellular matrix. Lamellas are formed after the elimination of an existing bone and as a result of remodeling process. In other words, the secondary bone tissue is such always in lamellar form. On the other hand, primary bone tissue is usually cancellous, but sometimes, it can be in lamellar bone form. For example, during environmental bone growth, environmental lamellas can be created by periosteal cells. Cancellous bone is formed faster than lamellar bone and contains less mineralized smaller crystals than lamellar bone, thereby more easily removed during remodeling. Lamellar bone consists of a process slower and more regular than cancellous bone, and mechanical properties are very important for mature bone. Lamellar tissue varies in cortical and cancellous bone.
Lamellar Cortical Bone
Cortical bone is organized into dense cylindrical lamellar structures – osteons which are also referred to as the Haversian system. Osteons are usually 3–6 mm in length and in diameter of 150–300 μ. In the center of osteons, there is a cylindrical cavity called the Haversian canal which vessels and poor myelinized nerve fibers pass through. Haversian canals are connected to each other or to surface of the bone by the Volkmann channels. The Haversian and Volkmann channels contribute a small amount to the porosity of cortical bone tissue. Each osteon is surrounded by cement lines (Figs. 18.2 and 18.3).
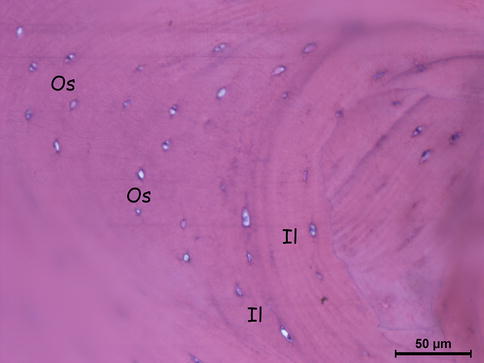
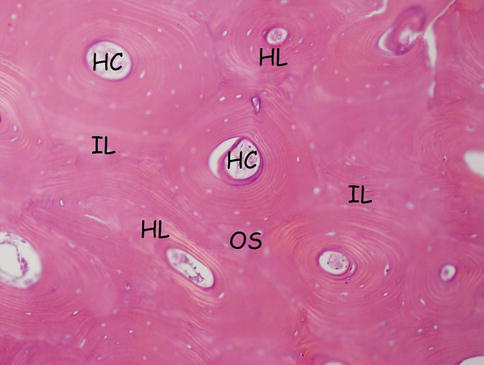
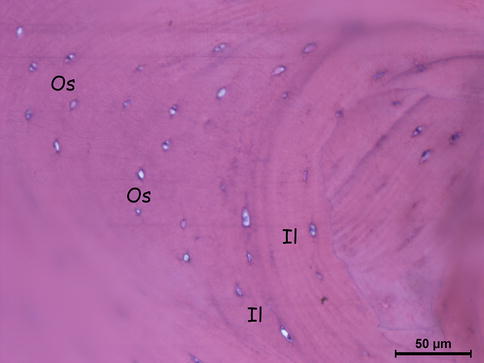
Fig. 18.2
Lamellar cortical bone’s cross section (osteocytes, interstitial lamellae). Os osteocytes, IL interstitial (intermediate) lamellae
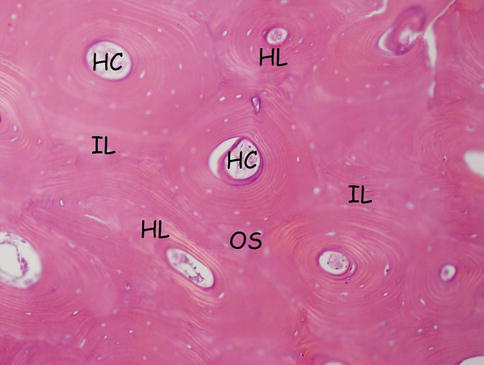
Fig. 18.3
Haversian system: osteon, Haversian canal, and Haversian and interstitial lamellae. HC Haversian canal, HL Haversian lamella, OS osteon (Haversian system), IL interstitial (intermediate) lamella
Lamellar Cancellous Bone
As in cortical bone, spongiosa is also subject to be organized into lamellae surrounded by cement lines [7].
Lacunae/Canaliculi
Gaps in the bone tissue are important for the mechanical properties and behavior. Cancellous or cortical bone tissue all contain osteocytes buried in the mineralized matrix. Osteocytes are present in the ellipsoidal cavity which is called lacunae. There are approximately 15,000 units of lacunae in each 1 mm bone tissue, and they constitute of 1 % of the bone. Recent studies report that the number and size of the lacunae can vary depending on bone health and the anatomical location. Most of the crack ends in lacuna, and starting from this point, it can be thought that lacunae stop the cracks and inhibit the progress of the cracks. Lacunae connect with each other, Haversian system, and bone surface by means of the canaliculi. Osteocyte cell extensions pass through in the canaliculi to connect with other osteocytes and bone cells on the surface. This network allows osteocytes for nutrients and oxygen, and again, this network removes waste. This network is very important that the mineralized matrix is not permeable to nutrients (Fig. 18.4).
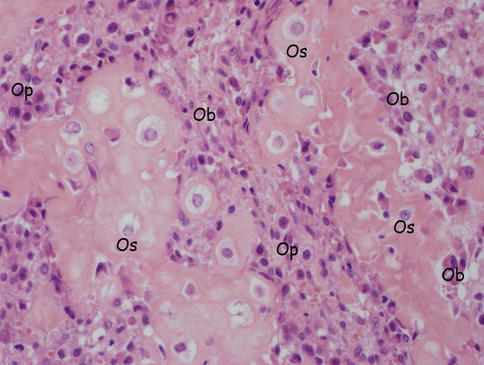
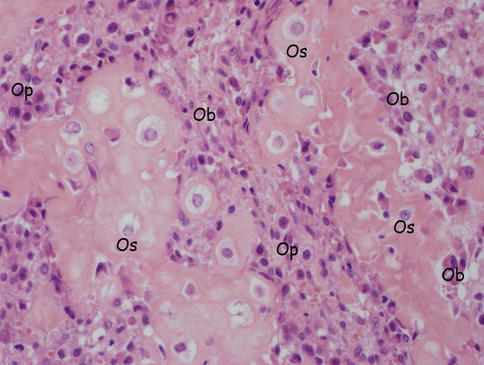
Fig. 18.4
Bone cells (osteocytes, osteoblasts, osteoprogenitor cells). Os osteocytes, Ob osteoblasts, Op osteoprogenitor cells
Cement Lines
Cement lines are located in both cortical and secondary cancellous bones. Their thickness is 1–2 μ, and they contain small amount of collagen than the surrounding bone tissue. Cement lines form the boundaries of the reformed bone. Although their formation objectives are not clear, they are considered to be effective in stopping the progression of cracks as lacunae do. Osteocyte extensions rarely exceed the cement lines. This means that nutrients and chemical signals cannot pass effectively to osteon to another or to a trabecular package to another [5, 7].
Cellular Biology
Osteoblasts
They are tasked with bone formation. Overall, they are rounded and are arranged on the bone surface. Cellular structures according to the secretory structure expanded cisternal to include a well-developed Golgi apparatus. Newly formed bone is not mineralized, and this bone is called osteoid. Osteoid’s quality is closely related to bone-forming osteoblasts. Some of the osteoblasts switch into osteocytes by embedding into matrix after active bone production. Others come to align to the bone surface. These arrays of cells are flat-shaped surface and comprise a small amount of organelles [6].
Osteocytes are believed to be the last step in osteoblast differentiation. These cells are found buried in the osteocytic lacunae. Osteocytic lacunae are connected with each other by canaliculi with cytoplasmic continuation, and these canaliculi are important in the food and oxygen uptake from the capillary circulation. Surface area of osteocytes is quite large because of multiple cytoplasmic processes. In addition, these cytoplasmic bundles contain well-developed actin filament to detect mechanical stress. Given these features, osteocytes are believed to play a role in bone metabolism with mechanical stress detection and transmission. Recent studies of osteocytes showed that they release the channel that is activated by stress and shear stress-responsive elements [9].
Osteoblasts produce collagen, non-collagenous proteins (osteocalcin, bone sialoprotein, osteopontin, osteonectin), and several proteoglycans (decorin and the biglycan). Osteoblasts can also produce cytokines like insulin-like growth factor 1–2 (IGF), transforming growth factor (TGF), and bone morphogenetic protein 3 (BMP). These growth factors are important in the differentiation and function of osteoblasts and are stored in calcified bone matrix. Bone matrix is a storage location not only for calcium and phosphate but also for growth factors [8]. Significant alkaline phosphatase activity is available in plasma membranes of osteoblasts. More recently, experimental studies of mouse with defective tissue nonspecific alkaline phosphatase (TNAP) showed that TNAP’s behavior was like pyrophosphatase hydrolyzing pyrophosphate which acts as a calcification inhibitor and was found to increase the inorganic phosphate required for calcification. Although alkaline phosphatase activity in the plasma membrane of osteoblast is quite effective, it has little effect at the membrane which faces the osteoid tissue and at osteocytes’ membrane [10]. This histochemical evidence shows that the distribution of alkaline phosphatase is not always associated with calcification regions. Osteoblasts and bone matrix proteins’ interaction is very important in osteoblast differentiation and function.
Osteoblast cells are also important in osteoclast differentiation and activation, as they are effective in bone formation. Macrophage colony-stimulating factor (M-CSF) and nuclear factor kappa-B ligand (RANKL) which are essential in osteoclastogenesis are created by osteoblasts. In addition, osteoprotegerin (OPG) and trap receptor for RANKL are also synthesized by osteoblasts. Bone resorption formed by osteoclasts has to be connected to balance in RANKL and OPG which are released by osteoblasts [6]. On the other hand, the destructive agent, collagen matrix metalloproteinase 13 (MMP-13), is secreted by osteoblasts and osteocyte cells [9]. MMP-13 activity is stimulated by activator protein 1 and RUNX2 which are activated by parathyroid hormone (PTH) in osteoblasts [11].
Osteoblasts are formed from mesenchymal stem cells. These stem cells can return to chondrocytes, osteoblasts, myoblast, and adipocytes. This transformation is regulated by specific transcription factors. Transformation of chondrocytes is regulated by SOX 5, 6, and 9; PP and MyoD regulate transformation to adipocytes and myoblasts, whereas RUNX2/CBFA and osterix/Sp 7 activate the conversion of osteoblasts. In low-stress and high-oxygen-pressure condition, conversion to osteoblasts does occur, while in medium-stress and low-oxygen environment, conversion to chondroblasts does occur, and finally, in high-stress environments, differentiation is mainly to fibrous tissue. RUNX2 participates in the gene expression of osteopontin, bone sialoprotein, dentin sialoprotein, and TGF receptor 1, and RUNX2 is a transcriptional factor which connects to the structure that supports the formation of osteocalcin [11].
Osteoblast differentiation and function is regulated by 1,25-(OH)2D3, hormones like PTH and estrogen, and cytokines such as bone morphogenetic proteins (BMP).
Receptors found in osteoblasts and functions of them are summarized below [6, 11]:
1.
Parathormone: Osteoclastic activity with continuous stimulation and increased osteoblastic activity in intermittent stimulation. Activates adenylate cyclase.
2.
1,25-(OH)2 vitamin D3: Activates matrix, alkaline phosphatase, and osteocalcin synthesis.
3.
Glucocorticoids: Inhibit DNA synthesis, collagen production, and protein production.
4.
Prostaglandines: Activate adenylate cyclase formation and bone resorption.
5.
Estrogens: Activate bone formation and inhibit bone resorption. Increase mRNA levels for alkaline phosphatase synthesis. Inhibit activation of adenylate cyclase.
Osteocytes
They constitute 90 % of the mature skeleton. They are seen as the producing osteoblasts surrounded by newly formed osteoid. They are less effective in production of the bone than active osteoblasts. They are important for extracellular concentrations of calcium and phosphorus. They are stimulated by calcitonin and directly inhibited by PTH [6].
Osteoclasts
Osteoclasts, which are responsible for bone resorption, are multicellular giant cells. They are found in Howship’s lacunae which are formed by their resorptive activity. Active ones are in contact with calcified bone surface. They are separated from other bone cells by their size and being multinucleated cells (Fig. 18.5) [12].
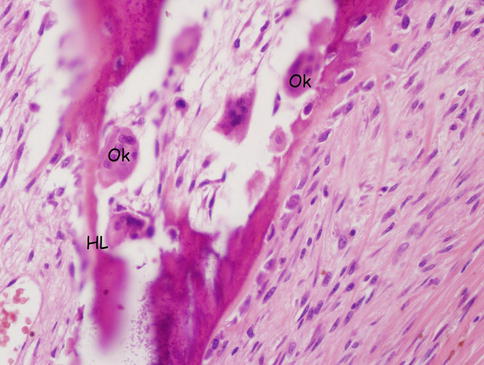
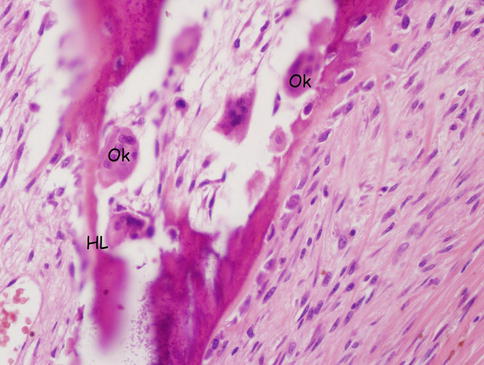
Fig. 18.5
Osteoclast and Howship’s lacuna. Ok osteoclasts, HL Howship’s lacuna
They have many mitochondria around the nucleus, endoplasmic retinaculum, and well-developed Golgi apparatus. In addition, they also contain the vesicles, lysosomes, tubular lysosomes, and vacuoles. The presence of these structures demonstrates that osteoclasts are important for energy generation, protein synthesis, and production of lysosomal enzymes [13].
Plasma membrane of active bone-resorbing osteoclasts is divided into three regions as clear zone, indented area, and basolateral membrane [14]. Clear region functions in attachment of osteoclast to the bone matrix and separates bone-resorbing compartment and extracellular fluid of cells from each other. This compartment provides effective situation for bone resorption [15]. The attachment of osteoclasts to bone matrix is provided with the vitronectin and integrin receptors. Another ligand is considered to be osteopontin. The cell matrix interaction activates c-Src, a non-receptor-type tyrosine kinase. Absence of c-Src activity generates a phenotype of osteopetrosis. There are many osteoclasts with non-recessed surface in this type of osteopetrosis. The face of the plasma membrane that is toward to osteoclast bone matrix is a recessed portion. Bone resorption is associated closely with the recessed surface.
Bone resorption would be the dissolution of degradation products such as hydroxyapatite and organic components of bone matrix. Carbonic anhydrase which converts CO2 and H20 to H+ and HCO3 − in the cytoplasm and vacuolar H+-ATPase in indented surface is involved in acidification in Howship’s lacuna. H+ ATPase is abnormal in osteopetrosis. Mitochondria in osteoclasts are believed to provide ATP for the transport of H+ [14]. The balance of ions in the cytoplasm is provided with chloride channels. Defect of these channels has been shown to cause osteopetrosis in rat experiments. Extracellular digestion of the organic components is provided by lysosomal enzymes such as cathepsin K. Cathepsin K can break down collagen fibers in an acidic environment. Tartrate-resistant acid phosphatase is released in Howship’s lacuna, and these enzymes are used as markers for osteoclasts. Basolateral plasma membrane is believed to be important for receiving calcitonin and cytokine stimulation. This area is also important in the cell-cell stimulation with osteoblast cells.
Calcitonin is a hormone whose receptor is on osteoclasts and that inhibits osteoclasts. This hormone breaks down actin filaments, eliminates the clear zone, and separates osteoclasts from the bone by retracting them [14, 15].
Osteoclasts are derived from precursor monocyte/macrophage cells and hematopoietic stem cells [16]. Macrophage colony-stimulating factor (M-CSF) is important for third derivation. Morphological findings support that osteoclasts and preosteoclasts are always in relationship with ALP-positive osteoblasts, and therefore, cell-cell relationship with osteoblasts is important for osteoclast differentiation. One of the most interesting findings in osteoclast differentiation is the effect of RANK/RANKL system. RANKL which is a member of the tumor necrosis factor family is produced by activated T cells and osteoblasts. RANKL takes a role in differentiation by binding to RANK on osteoclast precursors and osteoclasts. 1,25-(OH)2D3, prostaglandin E2, interleukin 1, PTH, and PTH-related protein, which are the stimulators of osteoclastogenesis, promote the formation of RANKL on osteoblasts. Conversely, osteoprotegerin (OPG), the soluble receptor form of TNF, inhibits RANKL. Estrogen, TGF, and BMP increase the formation of OPG. RANKL/RANK signaling is regulated by the TNF receptor-associated factor on osteoclasts [16].
Parathyroid hormone-related peptide which is secreted by the tumor cells forms RANKL. RANKL binds to specific receptors on osteoclasts, and then mature osteoclast formation occurs, and this process leads to the activation of bone resorption [14].
Extracellular matrix containing heparan sulfate and fibronectin is important for the connection between osteoclast and osteoblast cells. This connection is important for cell stimulation [10].
Bisphosphonate group of drugs is classified as nitrogen containing and nonnitrogen containing. Antiresorptive effects of nitrogen-containing bisphosphonates are 1000 times more than the nonnitrogen-containing bisphosphonates. Nonnitrogen-containing bisphosphonates create apoptosis by nonfunctional adenosine triphosphate (ATP), whereas nitrogen-containing bisphosphonates (zoledronate, alendronate, etc.) inhibit farnesyl pyrophosphate synthetase enzyme which is on mevalonate pathway [6, 16].
Molecular Structure
Matrix
Most of the bone consists of a matrix. Matrix has two components, including organic and inorganic.
Inorganic Matrix
Inorganic matrix is the mineral part of the bone and formed by calcium hydroxyapatite and calcium phosphate. It constitutes 60 % of the dry weight of the bone [6].
Organic Matrix
Organic matrix constitutes 40 % of the dry weight of the bone. Ninety percent of organic matrix consists of collagen and the very large amount of collagen is type 1. Other components are proteoglycans, non-collagenous matrix proteins (osteocalcin), growth factors, and cytokines.
The following section will provide more detailed information on the matrix.
Extracellular Matrix
Bone tissue is relatively less cellular than the other tissues. Extracellular matrix (ECM) comprises the majority of bone tissue. ECM is composed of mineral, protein, water, salts, lipids, glycoproteins, and proteoglycans and primarily consist of osteoblasts, the cells that form the bone. The bone is composed of different types of ECM, and these types are mineralized ECM, osteoid (non-mineralized) ECM, and lacunar ECM (matrix which surrounds osteocytes). Lacunar matrix is probably moving in the canalicular network of osteocytes [18].
Osteoid is a temporary matrix which forms mineralized bone. A small percentage of the volume of the bone is osteoid except for mineralization disorder diseases such as osteoporosis and osteomalacia. Although osteoid is generally present in the areas of formation of new bones, it can be seen as a thin layer on mature bone surface which assumed a critical role in the regulation of remodeling.
Mineralized Bone Extracellular Matrix Components
Mineralized bone matrix defines bone tissue material properties and accounts for the majority of bone matrix. Mature bone matrix contains significant inorganic components in addition to the organic components unlike lacunar and osteoid matrix. Mineral constitutes 60–70 % of the tissue, primarily calcium, phosphate, sodium, and other appropriate ions such as magnesium carbonate. The organic matrix constitutes 20–25 % of the tissue, and 90 % of organic matrix is type 1 collagen, whereas 10 % is non-collagenous proteins. Types 3, 5 and small amounts of other types of collagen may also be present. Bone morphogenetic proteins (BMPs) and other growth factors are also found in the mineralized matrix. The remaining tissue volume is filled with water. All mineralized bone surfaces are covered with a thin layer of non-mineralized osteoid.
< div class='tao-gold-member'>
Only gold members can continue reading. Log In or Register a > to continue
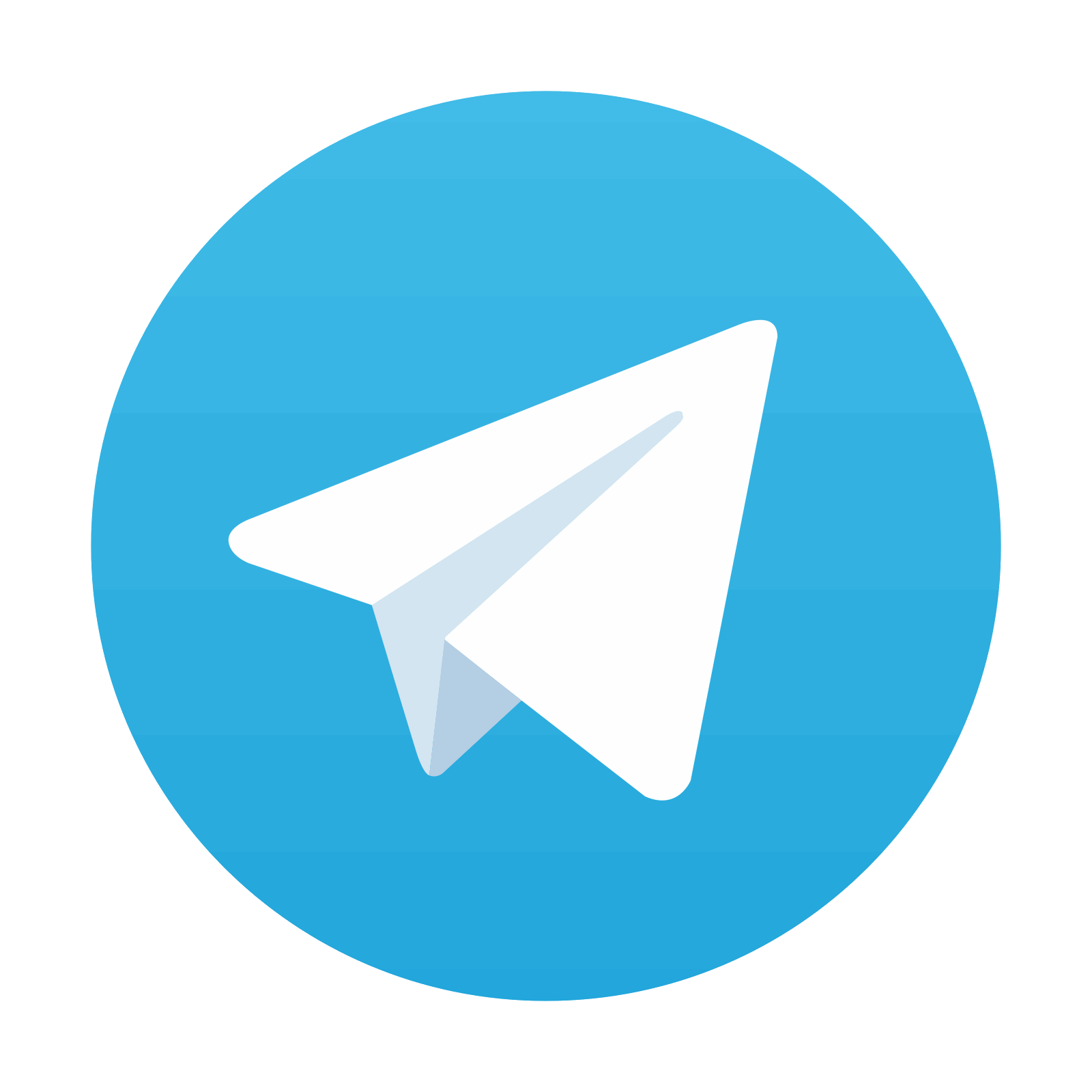
Stay updated, free articles. Join our Telegram channel

Full access? Get Clinical Tree
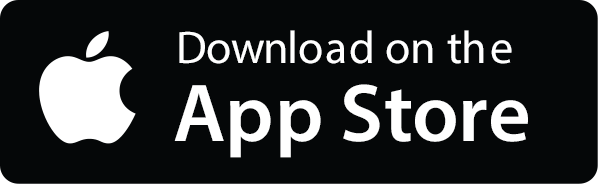
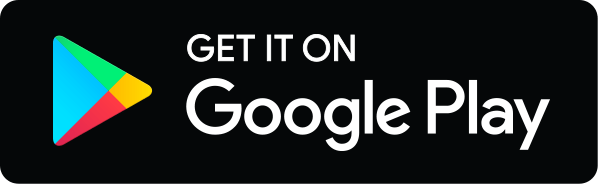