Formulation
Type
Volume (mL)
Platelet concentration over plasma
WBC content
Cascade (MTF)
Platelet-rich fibrin
4.5
1.3×–1.7×
Low
ACP (Arthrex)
Platelet-rich plasma
4–7
2×–3×
Low
GPS III (Biomet)
Leukocyte-rich plasma
6
2×–8×
High
Symphony II (DePuy)
Leukocyte-rich plasma
7–10
3×–7×
High
PRP is typically prepared through a centrifugation or filtration process that separates anticoagulated whole blood into liquid and solid components. A potential disadvantage of centrifugation is that it may cause fragmentation of the platelets that may cause early release of growth factors and cytokines. Newer, cell-sensitive filtration systems are available that have the potential to mitigate this variable in an operating room or clinical setting [20]. There are more than 40 commercial systems available that purport to convert blood into PRP. Final concentrations of all of the aforementioned variables may be secondary to both the system chosen in addition to the inherent variability and technique employed within each system [21, 22]. Patient-specific factors such as age, medical comorbidities, and circulation may also lead to differences in growth factors and platelet concentrate [23]. Current attempts to create classification systems for PRP so that results can be standardized and compared among studies are under way and will be important when comparing the results from different studies [24]. In a recent Level V paper, DeLong and colleagues devised the PAW classification system in an attempt to compare publications based on three variables of PRP: the absolute number of platelets in the preparation, the manner in which platelet activation occurs, and the presence or absence of white blood cells [19]. Platelet concentration is graded on a scale from P1 to P4, with P4 being concentrations greater than 1,250,000 platelets/μL. White blood cell content of the preparation is graded as either A (above baseline values) or B (below/equal to baseline levels). Neutrophils are graded separately in this system to be either α (above baseline) or β (below baseline). Method of activation is categorized as either endogenous or exogenous (x) in the scheme. The authors cite an example of their system by classifying a PRP preparation with 900,000 platelets/μL with a total WBC and neutrophil content above baseline levels as being a P3-Aα. Classification systems such as these are paramount for researchers to standardize their reporting of the preparation used so that reproducibility of results by other investigators can be documented.
Current Clinical Applications
PRP has gained greater acceptance in orthopedic practice and more specifically, sports medicine. Initially, PRP was used clinically in wound healing, dermatology, and oromaxillofacial conditions [15, 17]. It has demonstrated osteogenic properties in preclinical and in vitro studies; however, clinical outcome studies have not been as promising [25]. In the realm of sports medicine, PRP has been studied for potential positive effects in cartilage healing and chronic tendinopathy. TGF-β1, thrombospondin-1, and IGF have all been found within PRP or upregulated after application of PRP, all of which have the capability of promoting joint repair. In a level I randomized study evaluating the efficacy of PRP (WBC filtered) for bilateral knee osteoarthritis, patients treated with PRP were reported to have better outcomes than controls at 6 months [26]. Despite several other level I evidence studies citing the therapeutic benefit of PRP for the treatment of osteoarthritis of the knee, the current recommendation of the American Academy of Orthopaedic Surgeons based on their released Clinical Practice Guideline, however, is that they were “unable to recommend for or against growth factor injections …for patients with symptomatic osteoarthritis of the knee” [27]. While reversing the course of degenerative arthritis of the knee may be a significant feat for an orthobiologic application, tendinopathy offers perhaps a more feasible goal to harness the body’s own capability to promote healing where a halted biologic response has occurred. With regard to lateral epicondylitis, it has been demonstrated that PRP formulations containing white blood cells improve patient outcomes when compared with nonoperative treatment or injections of local anesthetic, whole blood, or corticosteroid [28, 29]. The results of PRP treatment for other tendinopathies (Achilles, patellar) have not been as significant as that for lateral epicondylitis; however, a recent investigation by Bouyer demonstrated excellent restoration of function and architecture of the patellar tendon by magnetic resonance imaging (MRI) after three consecutive injections of ultrasound-guided PRP [30–32]. Further study is needed to determine what dosage and frequency of administration may be able to offer patients with these maladies the most therapeutic benefit.
While PRP has the potential to augment a biologic response in the context of nonoperative treatment, it has also been employed as an adjunct treatment for patients undergoing surgery of the anterior cruciate ligament (ACL), rotator cuff tendon, and Achilles tendon. Successful ACL reconstruction is contingent upon graft incorporation into the femoral and tibial bone tunnels through a biologic response. It has been postulated that graft remodeling may be enhanced by PDGF, TGF-β1, and IGF-1. In a recent investigation, the use of PRP-gel was found to significantly affect the maturation of ACL grafts by MRI at 1-year follow-up [33]. In a similar study using semitendinosus grafts in a randomized controlled trial design, similar findings were found at 6 months in the group that was augmented with platelet concentrate [34]. A systematic review of the literature on this topic demonstrated a potential positive effect on ACL surgery by enhancing graft maturation by 20–30 % [35]. For patients undergoing rotator cuff repair, the task of healing is even more daunting as the rotator cuff tendon is often torn in a degenerative mechanism. In a systematic review evaluating the effect of PRP on rotator cuff healing after surgery, it was found that PRP demonstrated no beneficial effect with regard to re-tear rates or clinical outcomes [36]. In one investigation, platelet-rich fibrin matrix therapy was found to negatively affect repair integrity at 3-month follow-up [37]. The results of studies on Achilles tendon repair have been rather equivocal, similar to those findings seen in rotator cuff surgery, and to date no study has demonstrated a decreased risk of re-rupture with biologic augmentation utilizing PRP.
Effects of PRP on Muscle Tissue
While PRP has shown some benefit in the setting of chronic tendon inflammation, it is unclear what effects PRP demonstrates on muscle tissue. A recent investigation evaluated the effects of PRP on myoblasts in culture. This study demonstrated that an early differentiation marker (MyoD) was upregulated after 7 days, indicating a potential biologic effect on muscle tissue [38]. In a muscle injury model, PRP was applied to muscles that were injured in experimental rats. It was found that the muscle tissue treated with PRP demonstrated greater leukocyte infiltration and contralateral muscle also demonstrated an increase in protein expression of the involved leukocytes, indicating a systemic response that magnifies the early inflammatory response after muscle injury [39]. Platelet-rich fibrin matrix has also been shown to improve muscle regeneration, promote neovascularization, and inhibit fibrosis in treated muscle tissue [40]. In a small animal injury model evaluating the effects of PRP on muscle strains, local delivery of PRP into a multiple strain muscle injury demonstrated improvement in recovery time and restoration of full contractility [41]. In an attempt to determine the optimum PRP application for muscle tissue, Mazzocca and colleagues [42] exposed muscle tissue to three different PRP preparations (lower platelet concentration, high platelet and white blood cell concentration, high platelet and low white blood cell concentration). The results of this study demonstrated that myocytes treated with a low platelet preparation demonstrated significantly more proliferation than controls and the other preparations studied. Studies such as these can further define the optimum concentration of platelets required for the specific clinical setting. For example, this same study demonstrated that tenocytes are also positively affected by PRP with a low platelet concentration; however, tenocytes also favorably responded to the high platelet concentrations. There is promise that, in the future, specific preparations for the tissue being treated will be available and may further calibrate the biologic response intended.
Results of PRP for Hamstring Injury
The senior author (JPB) has reported on his experience of autologous conditioned plasma (ACP) injections given within 2 days of acute hamstring injuries in NFL football players [43]. These results demonstrated an earlier return to play of 3 days for grade I injuries and 5 days for grade II injuries. There was a one-game overall difference in return to play and 0 % risk of recurrence of injury in this patient cohort. In another review of 15 patients with 17 proximal hamstring injuries, 12 patients underwent local injection of PRP for insertional injuries that failed conservative treatment. While all the patients in this series returned to their desired activity level, the PRP-treated group demonstrated a significant reduction in visual analog scale scores and Nirschl Pain Phase Scale scores [44]. In a multicenter review of patients receiving ultrasound-guided PRP injections for chronic tendinopathy, 17 patients underwent treatment for a hamstring muscle/tendon injury [45]. The authors of this study demonstrated that greater than 80 % of patients reported complete resolution of their symptoms at final follow-up. While the majority (60 %) of patients only received one injection, a significant number of patients did require three or more injections (10 %) during their treatment course. In a pilot study evaluating the effects of PRP on muscle injuries, Wehling and colleagues [46] demonstrated an improvement in the recovery time of nearly 1 week for treated muscles in addition to accelerated healing on high-resolution (MRI) imaging. In this study, 6 of the 18 patients studied had hamstring muscle strains. To date, no level I studies have been completed that demonstrate efficacy of PRP in the treatment of hamstring muscle injury. Recently, a randomized controlled trial protocol was introduced in an effort to study the effects of PRP on grade II hamstring muscle injury [47]. There is hope that this investigation can more definitively answer the question of whether biologic therapy for hamstring strains improves recovery, reduces the risk of reinjury, and is clinically safe for practice.
Author’s Preferred Technique for PRP Administration
Patients who sustain an injury to the hamstring muscle are first graded based on MRI criteria (grades I–III) as previously defined [48]. In this grading system, the score is based on patient age, number of muscles involved, location of injury, presence of insertional damage, percentage of cross-sectional muscle involvement, length of muscle retraction, long axis T2 sagittal plane signal abnormalities, and the presence of chronic changes. Patients are initially rested for 24–48 h to allow for clotting at the site of injury and endogenous platelet activation to occur. After this time, using either ultrasound guidance or fluoroscopy with correlation to MRI, injection of a leukocyte-poor PRP preparation (ACP—Arthrex, Naples, FL) is placed within the zone of injury. Patients then receive routine post-injury care by athletic training staff with standard rehabilitation protocols and minimal stretching. The patient is then seen a week later for a repeat injection at the same site as the prior inoculation. The patient is then seen a third week for a repeat clinical evaluation. If the patient is still symptomatic at this visit, a third injection is performed. If the patient is asymptomatic at this time, they are released from care. Patients are allowed return to play when they demonstrate near full strength and flexibility, with minimal to no pain at the site of injury.
Growth Factors and Stem Cell Therapy
There are several potential therapies that may enhance the biologic healing for hamstring injuries, although little data exists at this stage. These include stem cells and delivery of specific growth factors, many of which are contained within the α-granules of platelets. Stem cells have the potential to regenerate tissue by direct expression of cell types that enable healing and repair. For example, animal studies have shown that in models of muscle injury, muscle-derived stem cells (MDSCs) improve both muscular structure and muscle regeneration [49, 50]. In vitro tissue engineering allows either stem cells or growth factors to be placed on a scaffold for direct delivery into damaged tissue. The hope is that ultimately, isolated MDSCs could be seeded onto a scaffold carrier and placed within a specific zone of injury, such as a hamstring muscle, providing the necessary biomaterials for complete repair and recovery of injured tissue [51]. While all of the recent advances in laboratory and bench research are promising, no clinical applications of stem cells have been introduced for the repair and healing of hamstring injuries.
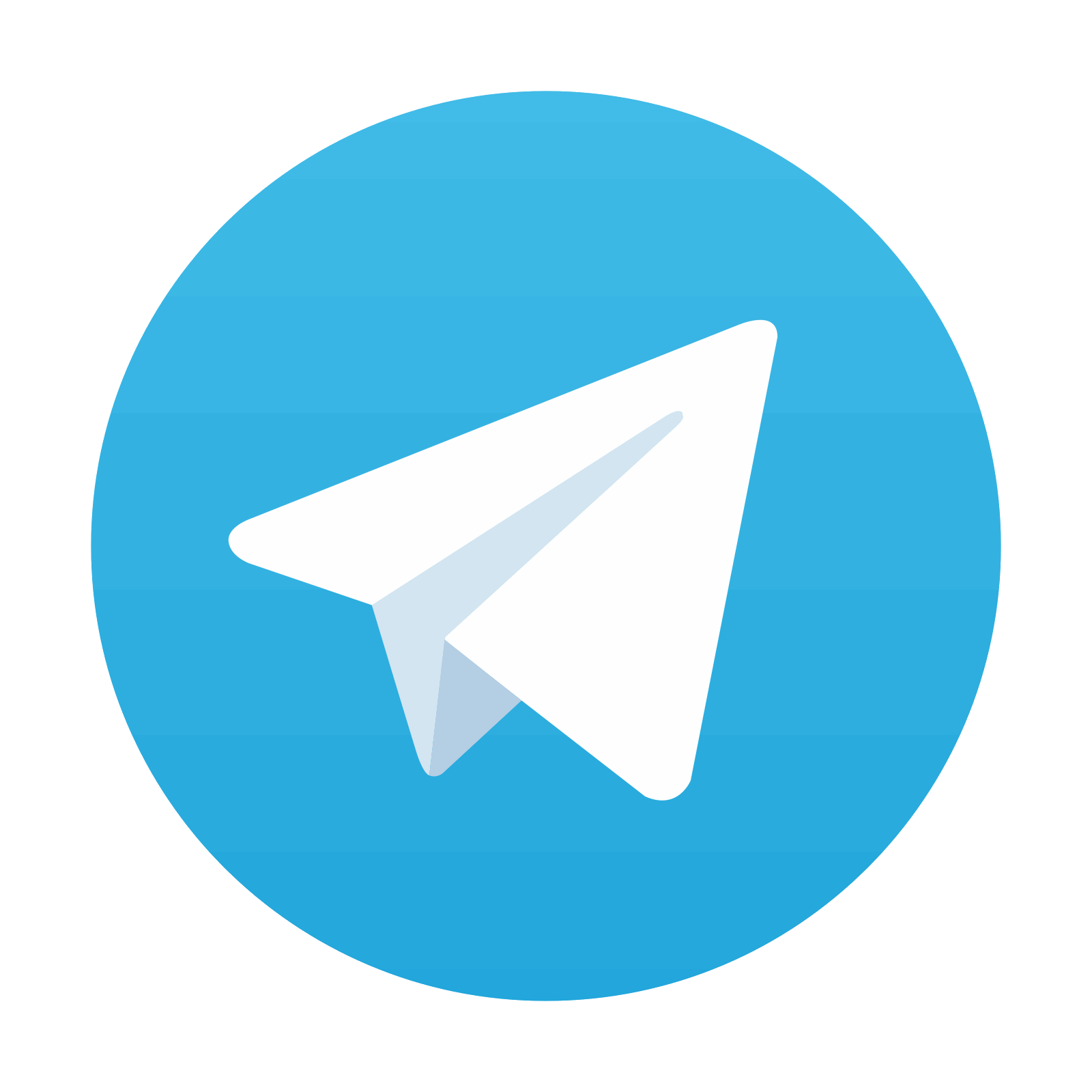
Stay updated, free articles. Join our Telegram channel

Full access? Get Clinical Tree
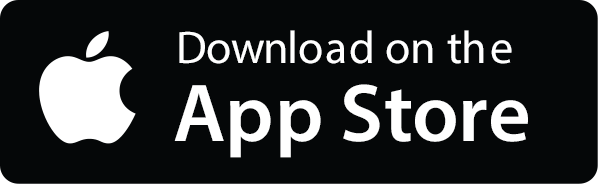
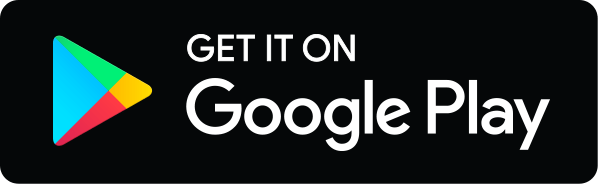