Arthroscopic Treatment of Degenerative Arthritis of The Knee
Jack M. Bert
Significant controversy exists regarding the arthroscopic treatment of osteoarthritis (OA) of the knee. The indications for arthroscopic treatment of OA of the knee alone and in conjunction with other arthroscopic procedures will be reviewed.
HISTORICAL PERSPECTIVE
Arthroscopic debridement for OA of the knee was initially reported by Burman et al. (1) in 1934. The authors reviewed the first 30 cases where knee arthroscopy was used to diagnose a “possible meniscal injury, arthritis in the knee, or suspected tumor (1, 2 and 3)”. In the group of arthritic cases, they had “the pleasant surprise of seeing a marked improvement in the joint following arthroscopy.” The authors stated that “arthroscopy involves only minimal risk, and in some cases has actually had a beneficial therapeutic effect, probably due to the thorough flushing and distention of the joint, which it necessitated.” (1) In 1941, Magnuson introduced the term “joint debridement” to describe an operation of the knee in which “all the accessible synovial membrane, osteophytes, diseased cartilage, and normal soft tissues were removed in an effort to relieve the symptoms of OA.” This was performed as an open procedure in which he stated that “complete recovery of symptoms” occurred in 60 of 62 patients (4).
During and after World War II, arthroscopy waned, and the open Magnuson procedure consisting of total synovectomy, osteophyte resection, cruciate ligament excision (if torn), as well as patellectomy was performed in most cases with reported symptomatic improvement in 66% of patients. This procedure became widely accepted as the treatment of choice for OA of the knee as published by Haggart (5) in 1947 and Isserlin (6) in 1950. These open debridement procedures, therefore, became the treatment of choice for arthritis of the knee until the resurgence of arthroscopy in the early 1970s.
CARTILAGE REPAIR
In 1743, William Hunter (7) stated, “From Hippocrates to the present age, it is universally allowed that ulcerated cartilage is a troublesome thing and that once destroyed it is not repaired.” In 1849, Leidy (8) confirmed this principle stating that “a rupture of cartilage fragments is never united and that articular cartilage lacks regenerative power and fracture gaps extending into the joint become filled with tough fibrous tissue (9).”
Redfern (10), in 1851, described the histology of induced wounds of the articular cartilage of dog joints and stated that the wound “healed perfectly by the ingrowth of fibrous tissue,” which he believed arose from the intercellular substance of the chondrocytes of the articular cartilage. However, as Mankin concluded in 1952, superficial lacerations of cartilage “neither heal nor progress to more serious disorders if they are small lesions.” On the basis of multiple animal studies, these superficial lacerations, therefore, are generally limited in progression and do not lead to clinical OA (11). He furthermore noted that deep lacerations may be clearly visible years after injury (12, 13 and 14). When the subchondral bone is thus disrupted, interosseous blood vessels expose bone matrix growth factors, causing fibrin clot formation. Inflammation introduces new cells into the cartilage defect and these cells proliferate and begin matrix repair (15). The matrix of articular cartilage has extraordinary biochemical characteristics. It is a hyperhydrated tissue, with estimates of water content ranging as high as 80%. It contains type I collagen consisting of two α and one α2 chains. The collagen of cartilage contains three α1 (type II) chains. Furthermore, the α1 (type II) chains of type II collagen have a different structure from those of type I. It is this type I collagen that is formed when fibrous tissue regenerates in attempts at forming normal hyaline articular cartilage (11, 12, 13 and 14, 16, 17, 18, 19, 20, 21 and 22). Furthermore, mature repair tissue has a relatively low proteoglycan concentration, and the proteoglycans do not resemble the large
elaborate molecules found in the articular cartilage. These reparative cells, therefore, do not produce tissue with the unique composition, structure, and biochemical properties of normal articular cartilage (15, 18, 23). After cartilage injury or during the progression of OA, some chondrocytes do proliferate but do not migrate through the matrix to enter the site of tissue injury. The repair tissue matrix, which is usually formed by undifferentiated cells containing primarily type I collagen, thus cannot restore normal articular cartilage properties. These reparative cells fail to organize the molecules they produce to create a strong cohesive structure like that of articular cartilage and they produce other types of molecules that may interfere with the assembly of the cartilage matrix. This abnormal matrix with its different composition and structure, therefore, adversely alters the material properties of the tissue (24, 25 and 26). These alterations compromise the ability of cartilage to survive and function in the highly stressed mechanical environment found in load-bearing joints and may lead to further cartilage degeneration and OA. Disruption of collagen cross-linking causes cartilage to lose its intrinsic tensile stiffness, strength, and shear stiffness, and this loss of proteoglycans and increased water content compromise its compressive and permeability properties (27, 28 and 29).
elaborate molecules found in the articular cartilage. These reparative cells, therefore, do not produce tissue with the unique composition, structure, and biochemical properties of normal articular cartilage (15, 18, 23). After cartilage injury or during the progression of OA, some chondrocytes do proliferate but do not migrate through the matrix to enter the site of tissue injury. The repair tissue matrix, which is usually formed by undifferentiated cells containing primarily type I collagen, thus cannot restore normal articular cartilage properties. These reparative cells fail to organize the molecules they produce to create a strong cohesive structure like that of articular cartilage and they produce other types of molecules that may interfere with the assembly of the cartilage matrix. This abnormal matrix with its different composition and structure, therefore, adversely alters the material properties of the tissue (24, 25 and 26). These alterations compromise the ability of cartilage to survive and function in the highly stressed mechanical environment found in load-bearing joints and may lead to further cartilage degeneration and OA. Disruption of collagen cross-linking causes cartilage to lose its intrinsic tensile stiffness, strength, and shear stiffness, and this loss of proteoglycans and increased water content compromise its compressive and permeability properties (27, 28 and 29).
Multiple treatments have been attempted to stimulate repair or reformation of the articular surface of the knee joint. Arthroscopically, these treatments include marrow stimulation procedures, debridement and shaving of fibrillated cartilage, and joint lavage. Other arthroscopic biologic articular cartilage treatment including osteochondral autografting or allografting will be described by other authors.
MARROW-STIMULATION PROCEDURES
The concept of drilling through eburnated bone to stimulate reparative cartilage formation was originally described by Pridie in 1959 (Fig. 67.1). Seventy-four percent of 62 patients believed their operation was a success and stated they would “have the operation again under similar circumstances (30).” To reconfirm the findings of Pridie, Akeson surgically removed the articular cartilage of the femoral heads of dogs and drilled the subchondral bone. He noted that after 1-year at the time of retrieval, “excessive loading destroyed the initial repair tissue or prevented formation of repair tissue.” The results also indicated that 1 year after surgery, the concentration of proteoglycans in the reparative cartilage was less than half of that found in normal cartilage (31). Mitchell and Shepard found that multiple small drill holes made in the subchondral bone of rabbit-knee-joints-stimulated repair from large areas of the articular surface. They found that repair tissue grew from the drill holes and spread over the exposed bone. However, large areas of repair tissue that had the appearance of hyaline cartilage began to fibrillate and deteriorate within 1 year. These experiments were the first that showed that abrasion or perforation of subchondral bone could stimulate repair of large areas of joint surface with fibrocartilaginous tissue, but the retrieved repair tissue lacked the proteoglycan concentration found in previously published studies of normal hyaline cartilage (32, 33).
![]() FIGURE 67.1. Picture of patient S/P Pridie procedure illustrating fibrocartilage formation in medial femoral condylar drill holes. |
Abrasion arthroplasty of grade 4 eburnated chondral lesions using motorized instrumentation was introduced by Johnson in 1981. This procedure is essentially an extension of the Pridie procedure except that in abrasion arthroplasty a superficial layer of subchondral bone, approximately 1 to 3 mm thick, is removed to expose interosseous vessels (Fig. 67.2). Theoretically, the resulting hemorrhagic exudate forms a fibrin clot and allows for formation of fibrous repair tissue over the eburnated bone (Fig. 67.3). In some patients, this fibrocartilaginous tissue
lasted up to 4 years but in Johnson’s (34) series, only one of eight biopsy specimens showed any type II collagen typical of hyaline cartilage at the time of arthroscopic review and biopsy, and the rest had types I and III collagen. In a series of patients at our institution who had abrasion arthroplasty, at 5-year follow-up exams, 15 had been converted to total knee replacement (TKA) and biopsies were obtained at the time of TKA. All patients had fibrocartilage and type I collagen on their biopsy specimens (Fig. 67.4A,B). In our series of 126 patients who had treatment of unicompartmental gonarthrosis with either abrasion arthroplasty or arthroscopic debridement alone, at 5-year follow-up examinations, 51% had good to excellent results with abrasion arthroplasty. Sixty-six percent had good to excellent results with arthroscopic debridement alone. However, all of these patients had complete obliteration of the medial joint space preoperatively. The results in our series were unrelated to age, presence of previous surgery, weight, extent of unicompartmental disease, presence or absence of joint space widening after surgery, and extent of residual varus or valgus deformity (35). Coventry and Bowman (36) noted that formation of hyaline-like cartilage occurred in the unloaded medial compartment of several patients after valgus upper tibial osteotomy (Fig. 67.5). This finding was confirmed arthroscopically by Fujisawa et al. (37) 12 to 18 months after upper tibial osteotomies, which implies that regeneration of reparative cartilage can occur secondary to unloading of bone alone without additional surgery.
lasted up to 4 years but in Johnson’s (34) series, only one of eight biopsy specimens showed any type II collagen typical of hyaline cartilage at the time of arthroscopic review and biopsy, and the rest had types I and III collagen. In a series of patients at our institution who had abrasion arthroplasty, at 5-year follow-up exams, 15 had been converted to total knee replacement (TKA) and biopsies were obtained at the time of TKA. All patients had fibrocartilage and type I collagen on their biopsy specimens (Fig. 67.4A,B). In our series of 126 patients who had treatment of unicompartmental gonarthrosis with either abrasion arthroplasty or arthroscopic debridement alone, at 5-year follow-up examinations, 51% had good to excellent results with abrasion arthroplasty. Sixty-six percent had good to excellent results with arthroscopic debridement alone. However, all of these patients had complete obliteration of the medial joint space preoperatively. The results in our series were unrelated to age, presence of previous surgery, weight, extent of unicompartmental disease, presence or absence of joint space widening after surgery, and extent of residual varus or valgus deformity (35). Coventry and Bowman (36) noted that formation of hyaline-like cartilage occurred in the unloaded medial compartment of several patients after valgus upper tibial osteotomy (Fig. 67.5). This finding was confirmed arthroscopically by Fujisawa et al. (37) 12 to 18 months after upper tibial osteotomies, which implies that regeneration of reparative cartilage can occur secondary to unloading of bone alone without additional surgery.
MICROFRACTURE
Blevens et al. (38) recommended a “microfracture” technique in which they used an arthroscopic awl to create multiple perforations into the subchondral bone arthroscopically. They reported 266 patients between 1985 and 1990, with 3.7-year follow-up using a similar grading system to the Outerbridge classification (39, 40). The indications for the microfracture technique includes a full-thickness, well-circumscribed cartilage defect on a weight-bearing surface of the knee with exposed subchondral bone (i.e., grade 4 lesions). After chondral surface debridement, the bone is perforated to a depth of 3 to 4 mm using an awl and the holes placed approximately 4 to 5 mm apart (Fig. 67.6). Blood should be seen emanating from the microfracture holes after perforation is complete. A postoperative rehabilitation program was used to provide motion without applying high-load stress to the treated chondral defect. Repeat arthroscopies were performed in 80 patients. In the “majority” of chondral defects, subchondral bone was covered with cartilage of “varying quality” and the term “hyaline-like” was introduced to describe the fibrocartilage surface. There was absolutely no evidence that hyaline cartilage was present
at the second-look arthroscopy, and the authors confirmed that the only type of tissue that has been seen to regenerate over these surfaces was fibrocartilaginous repair tissue. The authors furthermore stated that the “biochemical composition and durability of the presumed fibrocartilage repair tissue is unanswered.” Clearly, there is no evidence that hyaline cartilage is regenerated by marrow stimulation.
at the second-look arthroscopy, and the authors confirmed that the only type of tissue that has been seen to regenerate over these surfaces was fibrocartilaginous repair tissue. The authors furthermore stated that the “biochemical composition and durability of the presumed fibrocartilage repair tissue is unanswered.” Clearly, there is no evidence that hyaline cartilage is regenerated by marrow stimulation.
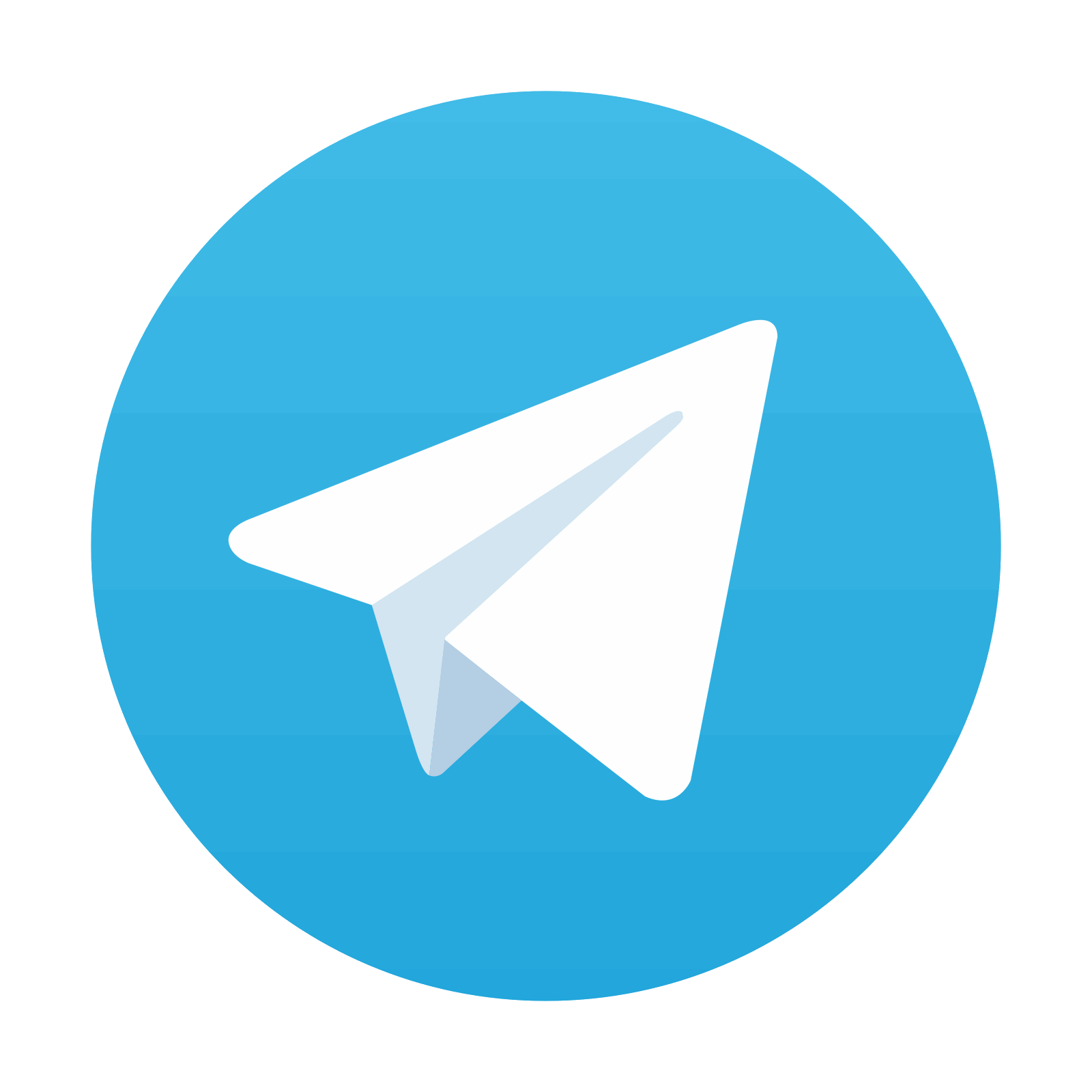
Stay updated, free articles. Join our Telegram channel

Full access? Get Clinical Tree
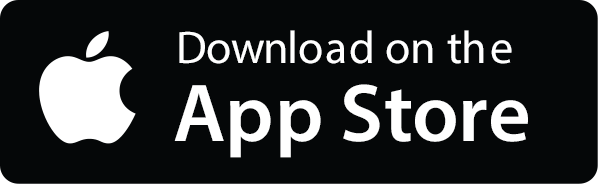
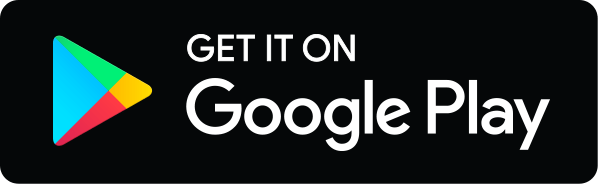