Simplified diagram of the vertebral system: 1. Environment, 2. Inside the body. I inputs, O outputs, SS sensory sensors, IC intrinsic control, MC medullary control, BC brain control, Ins instruction, VS vertebral structure, f feedback

Representation of the depreciating stabilization of a static equilibrium with respect to an instruction and the instability arising from fatigue

The precision is expressed by the difference between the instruction and the response. For static equilibrium (left) and dynamic equilibrium (right): 1. nominal trajectory; 2. disturbed trajectory
The vertebral structure includes vertebrae, discs, fibrous elements all composed of highly differentiated connective tissues, and muscles. It is also worth mentioning a host of sensory receptors (measurement of acceleration, force, displacements, etc.).
a tissue module that immediately responds to the constraints in compression, tension or torsion thanks to the mechanical properties of its connective and muscular constituents (elasticity, rigidity, and contractility) without any latency time.
a cellular module which, by mechanobiological effect, adapts in a delayed manner to the same constraints by synthesizing new protein constituents (various proteoglycans and collagens) which guarantee the homeostasis of the extracellular matrix. We are talking about adaptive remodeling.
the third functional control module of the column is a neural module that is both medullary and cerebral.
The motricity (motor function) of the vertebral structure is totally dependent on the spinal and cerebral nervous control centers where intangible instructions are developed for turning, until the action stops, in feedback loops where they undergo incessant adjustments (positive or negative gains) to match the results obtained (outputs) to the task required to control spinal stability. Any state of static or dynamic equilibrium is ultimately dependent on these feedbacks.
The first constituents (the structural elements) are material, and the second (the instructions and the feedbacks) are virtual.
The constitutive elements of any dynamic system are, therefore, interdependent and interact in a circular process of causality [2–7].
one is analytic that can identify its components and their connections, then evaluate their properties.
the other is synthetic, focused on the dynamic effects of their interactions. This is the systematic approach to the problem. Both methods are completely complementary.
This holistic method of approaching the complexity of the living resonates with any doctor. Medical diagnosis requires, firstly, a detailed and comprehensive assessment of structural and functional unit failures (symptomatology), then a second approach, this time synthetic, which consists of grouping the pathological data in a proper nosological setting and then establish a prognosis as relevant as possible.
- 1.
complete, for the structural analysis of “mechanical” vertebral pathology, the imaging data, as efficient as it is, by those coming from functional exploration techniques, which are already available, which should be done among the routine preoperative investigations (quantitative functional assessments, kinematic analyzes, numerical simulations);
- 2.
adopt methods of analysis of automation engineers, which have already become essential to progress in the understanding and management of complex pathologies in general. While the collaboration of the mechanics and the spinologist works perfectly, it is critical to develop it with the automation engineers. The analysis of the vertebral system according to the laws of systems engineering should cause an important strategic inflection in the field of spinal pathology.
Organization of a System
(Functional diagram, state variables, complexity, hypercomplexity, …)
The functional diagram of a system is a simplified graphic representation called a functional diagram, describing the hardware components and virtual links that convey information. It can be used in two ways: (1) for a qualitative analysis and (2) for a quantitative analysis if we reliably know the laws of each component and if the tools to do so exist [2, 3].
This is usually the case for common industrial servo systems whose components have well-defined properties and perfectly defined functional parameters: actuators (motors, cylinders), transformation and adaptation devices (drives, reducers), sensors and correctors (control elements for modulating the behavior of the server system). In these cases, the operating state of the system can, in principle, be known at any time thanks to the mathematical formulation of the functional parameters of each component and links (state variables ). This is the basic functional scheme of automobiles, planes as well as an industrial chocolate production line [2–7].
In these cases, the functional diagram provides an overall qualitative and quantitative representation of the system concerned.
We speak of linear systems when the effects are proportional to the causes. Basic knowledge of matrix theory and linear algebra is sufficient to understand and quantify them.
Nonlinear systems are more difficult to study. In these systems, the effects are not proportional to the causes. They cannot be described by linear differential equations with constant coefficients. Most physical systems are nonlinear.
Over the last decade, there have been important conceptual changes in the formulation of complex systems. New mathematical techniques have introduced some uncertainty in the modeling of controls with, as a consequence, a multivariable feedback. The integration of this type of uncertainty is seen as a progress, decisive in the treatment of complex systems because the group processing of certain multivariable parameters makes it possible to increase the robustness of these systems (robust control theory) [8, 9].
Living systems are immeasurably more complex than industrial systems. We can speak of hypercomplexity . These are open systems in constant interaction with the environment. They are maintained through variable flows of energy and information. Nonlinearity and variability of controls are the rule. The order does not exist a priori, it is created and maintained by the action (dynamic systems).
- 1.
The storage of energy material is limited so that their performance periodically collapses (fatigue) until the recovery of reserves after a shorter or longer period (recovery). In living beings, endurance thus becomes an essential parameter for maintaining performance [10, 11].
- 2.
The controls are not only electric but also biochemical.
- 3.
A living organism manufactures, maintains, transforms, and repairs (up to a certain point) its own components through a permanent process of genetically programmed production.
- 4.
Their performances vary spontaneously throughout their life (growth/learning, fullness, aging).
- 5.
Living systems can replicate.
Finally, the only invariant of living systems lies in their organization which ensures the durability of the action parameters (homeostasis) [8, 9].
Qualitative Analysis of the Vertebral System (Fig. 1)
Like all systems, the vertebral system is defined by a boundary that separates all its constituents from the environment. In the case of the vertebral system, it is the body skin that is the frame of the system.
The Entries
Solicitations from the outside environment are not information but physical interactions. They can, without transformation, directly reach the vertebral structure and trigger a passive instant mechanical response.
Another mode of entry follows the path of sensory transducers. It is these signals that trigger the activation of the system (the task at hand). To be integrated under the form of information that can be used by a living organism, these exogenous signals must be encoded [12] by transducers (sensors).
The task to be accomplished can also be generated within the system itself (endogenous signals). The order then comes directly from a nerve center. In these cases, no conversion is necessary.
Transducers (Receivers or Sensors)
Sensorial receptors (visual, vestibular, auditory, olfactory, or cutaneous) encode information from the environment for the brain.
Sensitive receptors encode endogenous information. They feed the interactions and continuously monitor a host of data including position, velocity, and even some parameters of tissue metabolism. They abound in the vertebral structure (mechanoreceptors, proprioceptors, chemoreceptors). There are intramuscular sensors (neuromuscular spindles) and sensors located in periarticular fibrous structures (free ending fibers, Ruffini and Pacinian corpuscles, Golgi tendinous bodies, etc.) [14]. At least seven types of myelinated afferent nerve fibers (I, II, alpha, gamma, Ib, III, etc.) emerge.
At this stage, the information is already desynchronized because each type of nerve fiber has a different conduction velocity depending on the thickness of the myelin sheath.
Mechanoreceptors combine to construct the kinesthetic sense.
It is of course necessary to add to this flow of circulating information which come from the sensory sensors of the pain.
All transducers and afferent fibers deteriorate progressively with age, but sometimes also because of neurological pathologies (degenerative or specific peripheral neuropathies). These abnormalities often escape the medical examination. They result in a loss of information or a delay in the transmission of signals which, by themselves, can compromise the equilibrium states of the spinal system by altering its performance [12, 13].
Controls
Intrinsic or tissue-based , organizes the expression of the mechanical and biological properties of the vertebral connective tissues.
Neurological , they receive the relative encoded information, integrate them, and develop an action program (motor program or instruction). For the vertebral column, there are two different but interconnected neurological controls: spinal cord control and cerebral control.
Cellular .
Tissue-Based Control
The mechanical properties of the connective tissue: bone, fibrous, or muscle of the vertebral column allow an instantaneous to mechanical stresses.
The components of the vertebral structure (discs, vertebrae, ligaments, and muscles) resist compressive or stretching forces by deforming. It is the collagen fibers, the elastic fibers, and the extracellular proteins that are responsible for this. Passive stretching of the tendons is used to store potential energy and then return it as kinetic energy, provided that the reaction is almost immediate to avoid dissipation in the form of heat. This phenomenon, called energy restitution storage, leads to a significant metabolic energy saving, for example in humans (up to 50% according to Linsted, 2002). It also intervenes at the spinal column level where it increases endurance in dynamic stabilization efforts. Thanks to their mechanical properties, the connective structures of the column thus participate totally in the control of vertebral stability , as well as the neurological activation of the musculature.
Cyclic mechanical stress plays a very important role in the movement of water and metabolites in the disc and articular cartilages. The hydration of the disc, for example, is by percolation through the epiphyseal plates largely under the effect of mechanical stresses.
Cellular Control
The mechanical constraints , moreover, regulate a good part of the cellular and extracellular metabolism of the components of the vertebral system through a network of macromolecules, a group of surface proteins, the integrins connected to the collagen fibers. They function as real mechanoreceptors. Prolonged mechanical stresses trigger an adaptive remodeling process of bone, fibrous, or muscular components. Genetically programmed, it involves an impressive number of intra and extracellular proteins (hormones), growth factors, enzymatic cascade, cell activity regulating factors such as MAP kinase (mitogen-activated protein), and also mobilizes stromal cells (i.e., strain). This mechanobiological control makes it possible to temporarily adapt the resistance of vertebral materials to the mechanical requirements of vertebral stability.
Neurological Checks
Spinal cord control is an automatic neurological control (reflex). The afferent signals from the sensors converge to the motor neurons located in the anterior horns of the cord at variable speeds depending on the number of synaptic relays (mono or polysynaptic reflexes). The operating time varies on average from 0.5 ms to 20 ms. It gets longer with age.
Pain signals are normally scrambled to a certain threshold by proprioceptive signals at the level of the posterior horns (gate control), but any peripheral demyelination may disturb this protective mechanism by improving nerve conduction gaps.
Cerebral control is more complex and slower (100–1000 ms).
The brain response is developed through neural modules often spatially and temporally remote.
Very schematically, the so-called voluntary cortical response begins with a process of identifying the task. A mental image of the body and its relationship to the environment is progressively elaborated by diffusion of the signals from the perception areas to the associative areas of integration. For vision, for example, information progresses from the primary visual cortex to the posterior parietal area, and then to the prefrontal cortex.
The next step is the motor programming. It obligatorily refers to the memory of sensorial and sensitive information relating to the experience of previous movements of the column (procedural memory). The memory then publishes tangible recipes of governance.
This predictive programming integrates the optimal tension of each muscle involved in the desired balance, corresponding to a specific posture or displacement. It must also determine the type (slow I, fast IIa or IIb) and number of muscle fibers to recruit in each muscle, and decide on the need for coactivation of other peri-vertebral muscle groups or even located at a distance from the column. If it is a question of maintaining a posture, the muscular contractions will be isometric, but most often it is a question of programming displacements which require dynamic contractions [10].
Cerebral control offers a great deal of flexibility in the choice of the most efficient and/or the least expensive energy control strategy (set shifting or task switching). The running of the motor protocol can even be pre-programmed, as for example in certain sports exercises or for any repetitive activity in general [15].
Motor programming is under the permanent control of the basal ganglia. It can, however, be interrupted, delayed, or modified at any moment under the effect of emotions. In this context, the motor response can be both shifted in time (1–2 s) and lose in precision with respect to the reality according to the degree of attention of the subject. The level of psychological “motivation” of the subject is also an important parameter for demanding efforts.
Finally, the decision to execute the motor program (the instruction) is formed at the level of the supplementary motor area, then reaches the actual motor area from which the axons of the pyramidal pathway emerge.
But programming and controlling muscles is not enough. The cortical signals that descend toward the medullary motor neurons are permanently controlled and filtered by the cerebellum which, consistent with the pyramidal pathway, has the peculiarity to represent only 11% of the cerebral volume but to possess by contrast 50% of the neurons of the brain human.
Finally, the action sequences (cerebral function) reach, via the spinal cord, the selected muscles whose finely tuned contractions are supposed to provide an optimal response.
Outputs
A system is essentially a variable transformer . The input variables (task to be accomplished) are transformed into output variables that are supposed to represent the task ideally accomplished.
In fact, the output data are not, in most cases, immediately optimal. In addition, the delay introduced by the process of setting up the motor program necessitates adjustments at the entry level.
Feedback
They ensure the adjustment of output variables to input variables. This device returns to the controls the output data that represents the action of the system on the environment. It is fundamental to the dynamics of change within the system.
This device is referred to feedback loops.
Positive feedback loops that amplify the behavior of the system to optimize the adjustment of the control.
Negative feedback loops , on the contrary, tend to stabilize the system when the action appears finalized.
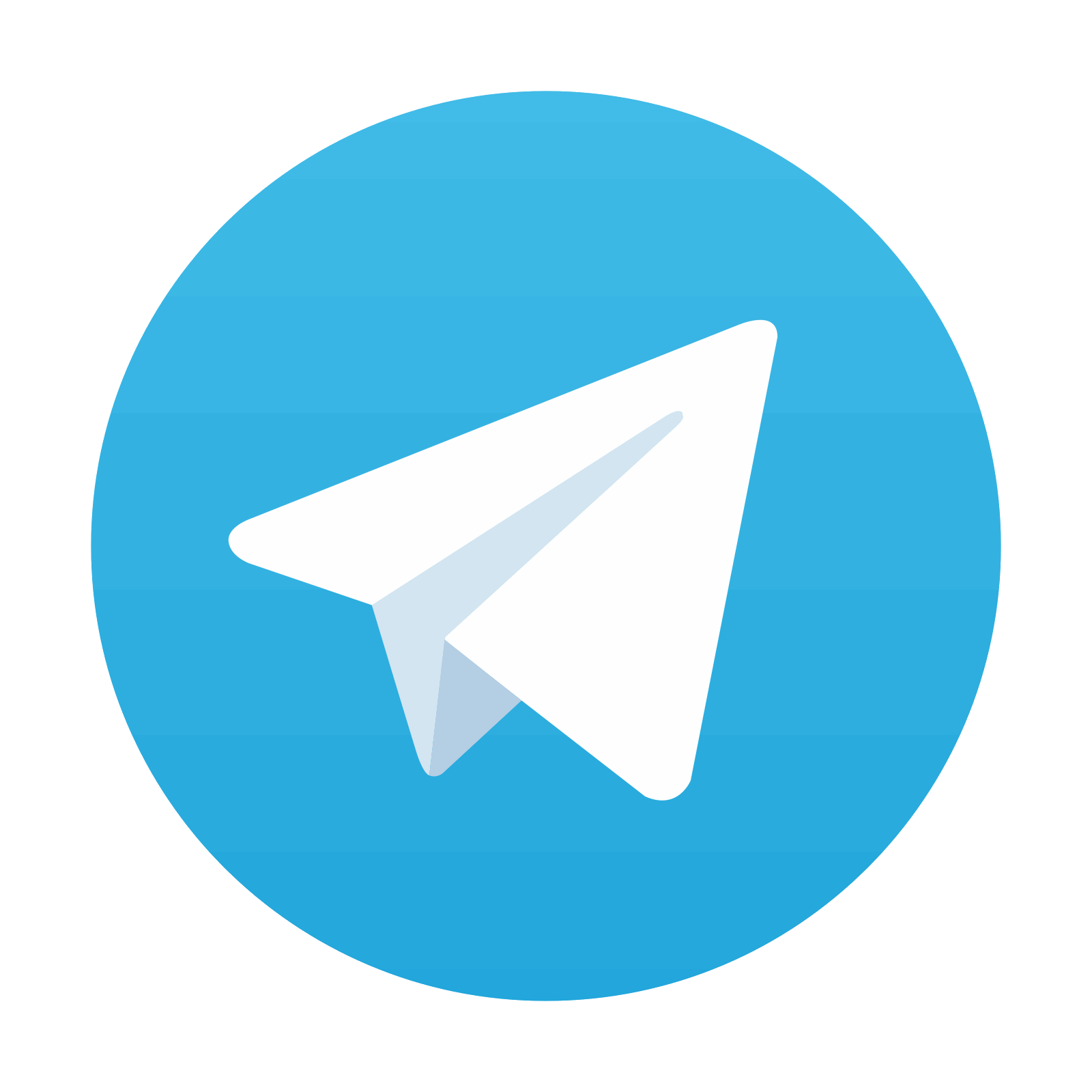
Stay updated, free articles. Join our Telegram channel

Full access? Get Clinical Tree
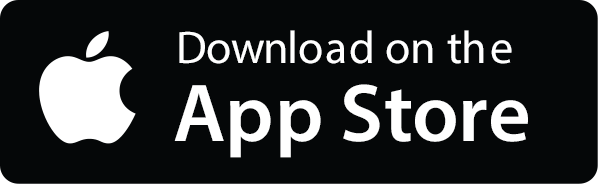
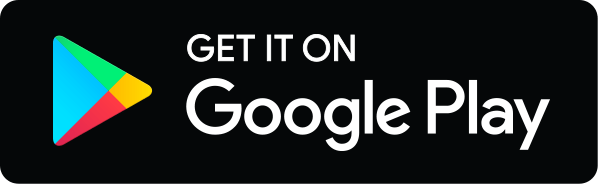