The body of the vertebrates is characterized by the existence of an anteroposterior corporeal axis and a cephalocaudal bipolarization. Head and neck complex—support of the CNS and sensory organs for the analysis of environment and nutrition. Postcranial complex—protection of vital organs and locomotion
Adaptive Constraints of the Living Environment
Constraints of the Aquatic Environment
It is thought that the aquatic environment is the original environment. Oxygen is relatively rare (7.2 ml/l vs 209.5 ml/l in air) and diffuses more slowly. Oxygen is extracted by gills, extensions of the splanchnocranium. In the branchial system of fish, the partial pressure difference of oxygen between water and blood remains constant.

(a) In the aquatic environment, the buoyancy depends on the hydrostatic weight/pressure ratio (Archimedes’ thrust). (b) In fish the body uses six degrees of freedom. The linear displacements are carried out by axial translation. Buoyancy—Hydrostatic thrust—Gravity
Constraints of the Terrestrial Air Environment
The terrestrialisation of tetrapods involves the extraction of oxygen from the air using lungs developed from the digestive tract. This active extraction requires the individualisation of a specific respiratory musculature whose action is reinforced by that of the appendicular musculature when the consumption of oxygen increases, especially in rapid displacements.
For the support of the body and terrestrial locomotion, the primordial adaptive stress is gravity (9.81 m/s−2). It must be balanced by the resistance of the locomotor apparatus to ensure body retention. On land, it is the limbs which take on most propulsion forces. The axial system loses its flexibility, especially in large mammals, but it nevertheless contributes significantly to rapid propulsion through deformations in the sagittal plane which modify the orientation of the pelvis and thus the axis of thrust of the limbs. This intervention of the axial skeleton is essential in fast paces. The vertebral column also plays a pivotal role in postural flexibility through rotation.
Finally, to survive, an animal must be able to attack and defend itself. For this purpose, the axial skeleton of the tetrapod allows the rapid projection and retraction of the mouth, armed with teeth, towards the prey or the aggressor.
Fish
(Approximately 25,000 Species)
The axial skeleton of primitive fish such as cyclostomes (e.g. lamprey) is a flexible rod, the notochord which is formed of vacuolated chondrocytes, surrounded by a fibrous sheath. The stiffness of this hydrostatic skeleton depends on the osmotic pressure of the colloid in the vacuoles of the chondrocytes. Its mechanical properties can be compared to those of an elastic rod combining longitudinal compressive stiffness and lateral flexibility. With this configuration, the propulsion of these species is the axial wave mode contraction of muscles composed of periaxial myotomes in sequence which generate a succession of undulating waves propagating from the head to the tail, while the animal moves forward [10].

The displacements of anguilliform fish (a) occur in an axial wave mode (the whole body is flexible and undulating). Most pelagic fish (b) move in an axial oscillatory mode (the propelling force originates mainly from the caudal fin). Certain fish with rigid bodies (e.g. balistidae, mormyridae and electric fish) move in a caudal oscillatory mode (the tail alone allows the propulsion)
In fish, the perivertebral musculature is organized into two groups, the dorsal epiaxial and ventral hypoaxial, separated by a horizontal septum. The myofibrils do not, however, attach directly into the vertebral parts. For pelagic fish (living neither close to the shore nor to the bottom), many myomeres regroup as large longitudinal fibre endings that resemble the organization of long muscles of tetrapods [15]. Propulsion of these species then takes place in the axial oscillatory mode, the lateral deformation of the body intensifying towards the caudal segment carrying a rigid fin.
The feeding of the fish is done either by suction/aspiration or by predators, by rapid acceleration of the mouth towards the prey. This gesture of rapid protraction of the head by impulse of the body involves the whole axial skeleton. This “push-forward-with-the-head” ability is evident with all tetrapods.
Tetrapodomorphic fish (actinistians such as coelacanth and rhipidistians like lungfish) have fleshy fins that participate in locomotion. Their pectorals have well-defined bony pieces prefiguring the anterior well-defined chiridian limbs. Tetrapod fish are characterized by the appearance of a cervical vertebra at the craniovertebral junction and chiridian limbs with three distinctly individual segments: stylopod, zeugopod and autopod. Thus, contrary to popular belief, the chiridian limbs first appeared in aquatic and non-terrestrial organisms. This is the principle of exaptation. At this stage, the functional coupling of the vertebral column of the appendicular skeleton for open-air locomotion is already in place.
A flexible cartilaginous or bony axial skeleton without vertebral differentiation but with truncal and caudal regionalization
The presence of an epiaxial and hypoaxial musculature
Only a lateral (in a horizontal plane) deformation of the column that ensures all locomotion with attack/defence behaviours.
Terrestrial Vertebrates
During life in the open air, the weight of the body is no longer balanced by hydrostatic thrust. It is the appendicular skeleton which counteracts gravity for the maintenance of the body and its displacements.
There are two spatial reference systems of terrestrial vertebrates:
- 1.
The external (exocentric) reference frame is fixed, locked on the gravitational vertical force from which the coordinates of the centre of mass of the animal are defined.
- 2.
The general internal frame of reference (egocentric) corresponding to the three axes of polarity, which is an expression of the animal’s body pattern (topological properties of the species).

Representation of the orthonormal reference formed by the three axes of polarity of the animal from the cephalocaudal axis of symmetry

In quadrupeds , the angular variations coupled between the thoracolumbar column and ilium can be measured by the spinopelvic angle. It is formed by the intersection of a line from T1 and the sacroiliac joint (the upper edge of the sacrum is very hard to visualize in animals) and the centre line between both the femoral heads (the bicoxo-femoral axis) and the sacroiliac joint. This is an adaptation of the spinopelvic angle described in humans
To analyse the relative movements of the thoracolumbar spine and pelvic girdle, we propose to use the angle formed by the spinopelvic line from T1 to the centre of the sacroiliac joint (easier to locate in tetrapods than the sacral end plate), and the axis of the ilium represented by the line from the acetabulum to the same point of the sacroiliac joint.
The angle of incidence formed by the axis of the ilium and that of the sacrum is not a reliable topological data in animals because, contrary to popular belief, the sacroiliac joint is mobile, varying within and between individual cases. In man also this mobility can vary from one subject to another.
The external and internal reference systems coincide only under certain conditions (as in the strict vertical position in human bipedalism). In all other cases, data switching is required to change the repository. In animals, this switching is performed automatically by the central nervous system, which reconstructs a global pattern of data from the two references, so that the animal knows the position, speed and acceleration of the elements, its environment, its body and its own components.
Amphibians (About 7000 Species)
In amphibians , the final regionalization of the vertebral column is in place. Although reduced to a single vertebra, the cervical segment articulates with two trochoid surfaces homologous to the occiput. This is the individualized subcranial junction.
In anurans (frogs), the trunk segment is limited to 4–8 elongated vertebrae connected to the pelvis by a longitudinal urostyle. Frogs have no ribs. The truncocaudal junction is the most mobile of the column. At rest, the column is flexed at this joint, while it extends during jumping.
In the urodeles (salamanders), the elongated truncal segment comprises 12–63 vertebrae with ribs connected to the sternum.
In amphibians, the posterior vertebral arch is complemented by three types of apophyses: diaphysis (transverse), zygapophysis (articular) and neurapophysis (spinous). The first segment of the upper limb (stylopod) is horizontal, characteristic of the transverse chiridium (buttressed limbs). In amphibians, the scapular girdle is independent of the vertebral column while the pelvic girdle is secured to the sacrum through the transverse sacral processes. The ilium houses the proximal end of the femur. The pubic and ischial parts play the role of levers for the proximal muscles of posterior limbs. The axial skeleton of amphibians shows no curvature in the sagittal plane (the functional sagittal curvature of the anurans corresponds to the truncopelvic junction). The axis of the ilium is vertical (sacropelvic angle = 90°). The lumbosacral angle is approximately 0°.

(a) In Anurans (frogs) the craniovertebral junction is functional. The truncal segment is short and prolonged by the urostyle. (b) In the jump, the column is extended to the level of the truncosacral junction. (c) For amphibians and certain reptiles (geckos, iguanas, and chameleons), the projection of the tongue is more economical than that of the whole body for the capture of prey (sit-and-wait predators)

Walking in Urodeles (salamanders) is done by side-to-side oscillations of the column causing an inverse rotation of the pelvis. The essential role of the limbs consists of lifting the body, while lateral deformations of the column pull the limbs forward
The reduction of the cervical segment to a single vertebra does not make it possible to carry out a rapid protraction/retraction of the cephalic end of the animal for the capture of prey. The low mobility of the occipitocervical junction allows it only to bite and swallow. For reasons of saving energy that propulsion of the whole body would require, most of these species are “sit-and-wait predators” [6]. The solution of an extensible tongue projected rapidly and powerfully towards the prey was selected by evolution in many amphibians.
Respiration of amphibians is ensured by the contraction of the buccal floor, without any involvement of the appendicular musculature as seen in reptiles and mammals.
Reptiles (Approximately 8950 Species)
- 1.
a semi-independent craniocervical junction with significant mobility;
- 2.
an epiaxial musculature differentiated into independent muscle groups;
- 3.
and the strengthening of ties between the sacrum and the pelvis.
The Cervical Spine
The atlas articulates with a single occipital condyle. The testudines (turtles) have up to eight cervical vertebrae, the caudal aspect of which articulates with the first truncal vertebra which itself is fixed to the shell.
In reptiles, epiaxial cervical muscles become inserted on the occiput, differentiating into three groups (vertebrocapitis superficialis and profundus, and intertransversalis capitis). These extensor muscles of the craniocervical junction have an antigravity action which is essential for maintaining the head, which is sometimes very heavy (e.g. crocodiles).
The movements of protraction/retraction are particularly pronounced in turtles, whereas in crocodiles and most squamates (snakes), it is still the propulsion of the body that ensures the projection of the head forward. In geckos, iguanas and chameleons, the “sit-and-wait attitude” prevails, with the projection of the tongue towards the prey.
The thoracic column of the crocodiles comprises 10–11 vertebrae, 5 lumbar and 2 sacral, the transverse processes of which have a close connection with the ilium.
Like the cervical system, the muscular masses are segregated into organized bundles (m. longissimus, m transverseospinalis and ilio costalis), which generate the lateral movements of the column when they contract asymmetrically, but induce an extension of the column during a symmetrical contraction [15]. Crocodilian limbs have a first horizontal segment (buttressed limbs). It is the muscles of the pelvis that raise the body of the animal (sprawling posture).
The amplitude of mobility of the hip joint is low (less than 45°). The axis of the ilium is vertical, perpendicular to the vertebral axis at rest (pelvic version = 90°). The spinopelvic angle varies from 80 to 100° in crocodiles.

In crocodiles , the axis of the ilium is perpendicular to the column. The posterior spinopelvic angle and the pelvic version approximate 90°
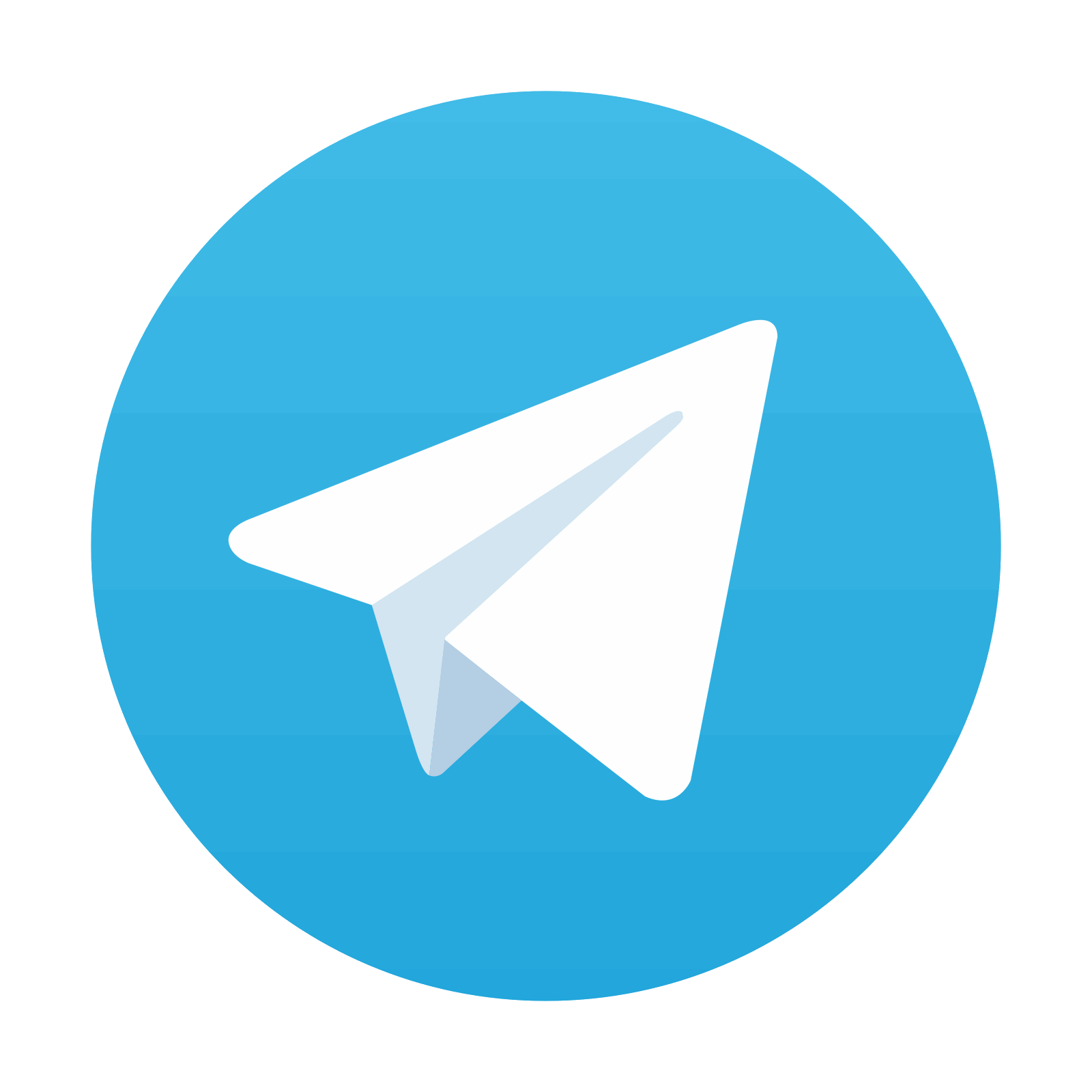
Stay updated, free articles. Join our Telegram channel

Full access? Get Clinical Tree
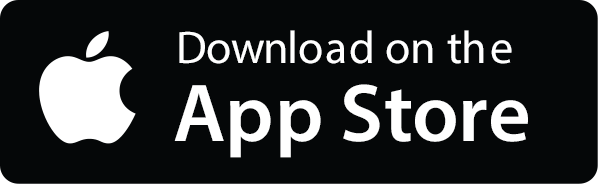
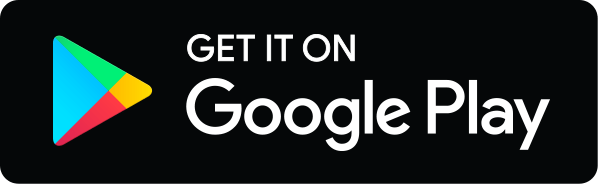