Fig. 2.1
a Posterior–anterior view of tibial plateau with PCL attachment marked. Note how the attachment area passes ‘over the back. b Proximal-distal view of tibial plateau with PCL attachment marked [62]. PCL posterior cruciate ligament
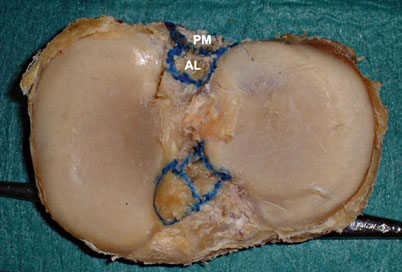
Fig. 2.2
Tibial footprints of the insertions of anterolateral (AL) and posteromedial (PM) bundles of the PCL [63]. PCL posterior cruciate ligament
The PCL has a wide variation in shape and size of its femoral attachments, whereas the tibial attachments size and shape are more consistent [5]. The substance of the ligament is made up of two distinct but inseparable bundles that allow for resistance of posterior translation in both extension and flexion. The bundles are named by their position within the femoral footprint/attachment: anterolateral bundle and posteromedial bundle (Fig. 2.3).To help identify these bundles during dissection or arthroscopy, other anatomical landmarks have been identified.
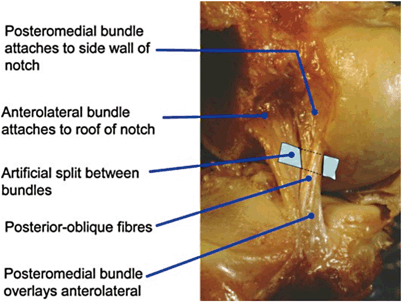
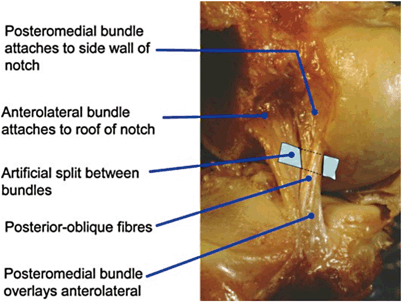
Fig. 2.3
The PCL fibers have been separated into the ALB and the PMB. Posterolateral view of left knee after removal of the lateral femoral condyle [62]. PCL posterior cruciate ligament, ALB anterolateral bundle, PMB posteromedial bundle
On the femoral side, the medial intercondylar ridge defines the proximal limit of the insertion of the PCL , whereas the medial bifurcate ridge separates the insertion sites of the two bundles (Figs. 2.4 and 2.5) [6]. There is a change in slope as each bundle approaches the femoral insertion site, putting the bundles in different planes when the knee is flexed. The PCL footprint on the femur is made up of approximately 55 % anterolateral bundle and 45 % posteromedial bundle. The mean distance between the centers of the anterolateral and posteromedial bundles on the femur is 12.1 mm. The distal margins of the anterolateral and posteromedial bundles are a mean 1.5 and 5.8 mm proximal to the notch articular cartilage, respectively [7]. While the femoral footprint size is nearly equal between the two bundles (Fig. 2.6), the anterolateral bundle’s crosssectional area is significantly larger than the posteromedial bundle. The anterolateral bundle is the major contributor to PCL strength.
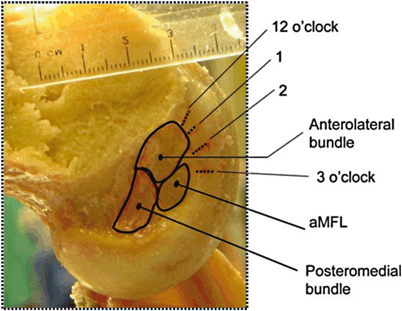
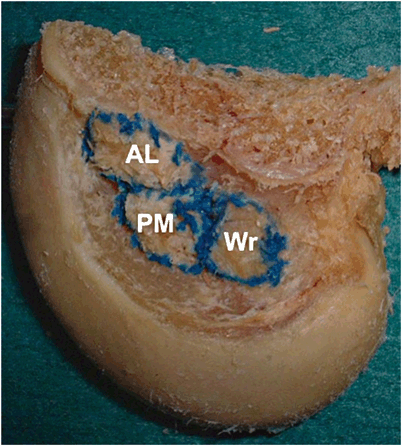
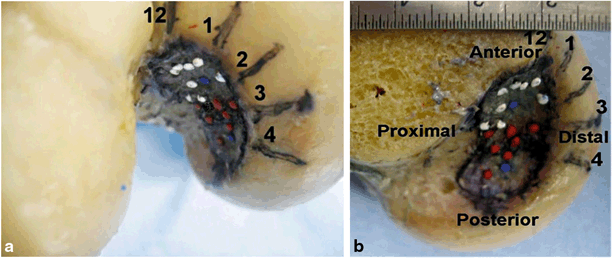
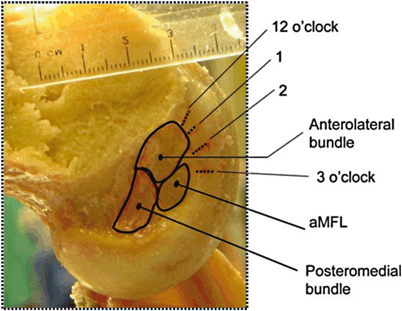
Fig. 2.4
The femoral attachment of the PCL. Lateral–medial view in a left knee after removal of the lateral femoral condyle. The anterolateral and posteromedial bundles of the PCL, plus anterior meniscofemoral ligament attachments are outlined [62]. PCL posterior cruciate ligament
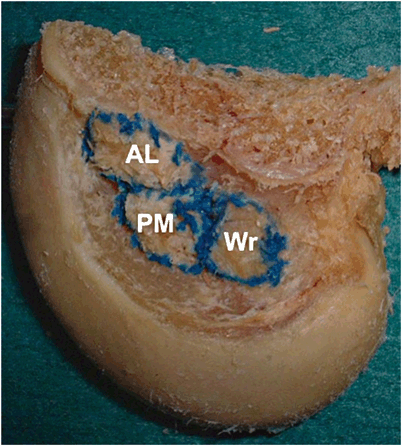
Fig. 2.5
Femoral footprints of the insertions of anterolateral (AL), posteromedial (PM) bundles of the PCL, and Wrisberg ligament (Wr) [63]. PCL posterior cruciate ligament
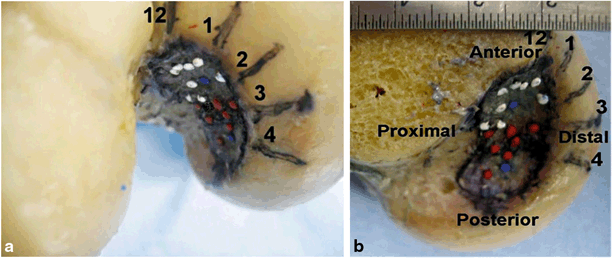
Fig. 2.6
a Positions of the anterolateral bundles (white) and the posteromedial bundles (red) and the anatomical centers of the two bundles of the PCL (blue) when viewed from anterior to lateral direction into the intercondylar notch in the knee flexed to 90°. b Positions of the anterolateral bundles (white) and the posteromedial bundles (red) and the anatomical centers of the two bundles of the PCL (blue) as seen in the sagittal section to view the medial femoral condyle [64]. PCL posterior cruciate ligament
The tibial insertions of the anterolateral and posteromedial bundle occur within the PCL fossa which is trapezoidal in shape and becomes wider inferiorly. The anterolateral bundle is attached at the superolateral aspect of the footprint and the posteromedial bundles are seen in the inferomedial portion of the fossa. The identification of each bundle is made easier with each bundle attachment having separate slopes. Across 21 knees, this change in slope angle was found to be an average of 14.5°. Also, an extensive portion of the posteromedial bundle is below the posterior part of the tibial rim, whereas none of the anterolateral bundle attachment is below the tibial rim. The superolateral and superomedial corners of the footprint were both represented by depressions and a reproducible ridge represented the inferior border, all of which could be identified with arthroscopy [8].
Johannsen et al. analyzed the posterior root attachments of the medial and lateral menisci, quantifying their position in relation to the PCL (Fig. 2.7). The lateral meniscus posterior root attachment center was 4.3 mm medial to the lateral tibial plateau articular cartilage edge and directly 12.7 mm to the most anterior edge of the PCL tibial attachment. The medial meniscus posterior root attachment center was 9.6 mm posterior and 0.7 mm lateral from the medial tibial eminence, and 8.2 mm anteromedial from the PCL. This anatomy is very important during PCL reconstruction as nonanatomic tunnel placement may compromise the meniscal root attachment.
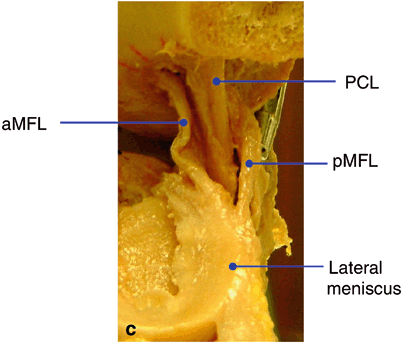
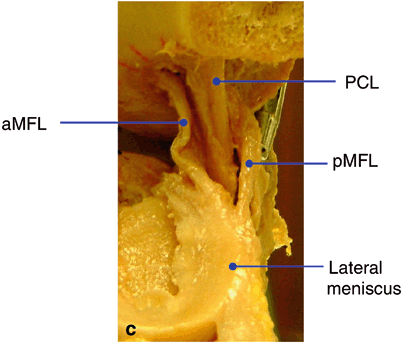
Fig. 2.7
Posterior view of knee showing the PCL attachment on the tibia and accessory ligaments located posteriorly [65]. PCL posterior cruciate ligament
The meniscofemoral ligaments are two distinct structures with variable incidence that run from the posterior horn of the lateral meniscus to the lateral aspect of the medial femoral condyle. The ligaments are named based on their location in relation to the PCL . The anterior meniscofemoral ligament is also known as the ligament of Humphrey, while the posterior meniscofemoral ligament is also known as the ligament of Wrisberg. The anterior meniscofemoral ligament is sometimes confused for the PCL during arthroscopy, albeit less than one-third the diameter of the PCL. The posterior meniscofemoral ligament can be nearly half the size of the PCL. Tugging on either of the meniscofemoral ligaments should reveal obvious motion of the lateral meniscus and thus will help you to identify it from the PCL. Multiple research studies looking at cadaver knees found the presence of either the anterior or posterior meniscofemoral ligaments in ~70 % of the time. Anderson et al. found that in those knees where both meniscofemoral ligaments were present, the posterior meniscofemoral ligament, posteromedial bundle, and anterior meniscofemoral ligament were aligned parallel to each other, proximally to distally [7]. The posterior meniscofemoral ligament is located directly proximal to the medial intercondylar ridge, proximal to the posteromedial bundle. There are no attachments from the PCL to the medial meniscus.
The posterior joint capsule runs in near continuation with the PCL . The posterior joint capsule originates above the femoral condyles and extends distally to the posterior margin of the tibial plateau. The posterior capsule is within 1–2 mm of the posterior aspect of the tibial attachment of the PCL. The anterior wall of the popliteal artery lies approximately 7–10 mm from the posterior border of the PCL at 90° of flexion [3]. Matava et al. found the distance between the PCL and popliteal artery was maximal at 100° of knee flexion , with measurements of 9.9 mm in the axial plane and 9.3 mm in the sagittal plane, using magnetic resonance imaging (MRI) [9]. There is an anterior septum between the capsule and PCL that is made up of fatty tissue wrapped in a thin synovial membrane which creases a triangular thickening. In the upper third of this tissue is the entry point for the bundle of the middle genicular artery, above the oblique popliteal ligament. Ahn et al. advocate for release of the posterior capsule. They believe it increases the distance between the insertion of the PCL and the popliteal artery, providing an increase in the volume of the posterior compartment during arthroscopy through expansion of this septal tissue. Greater posterior compartment volume enables better viewing of the insertion of the PCL and lowers the risk of neurovascular complication [10].
The blood supply to the PCL comes from the middle genicular artery, a branch of the popliteal artery. The middle genicular artery shows variations in its origin off the popliteal artery. The thin synovial sheath vessels that surround the cruciate ligaments are also seen in the fat pad have been found to be major contributors. These end arteries appear to branch from the middle genicular artery [11]. Capsular vessels supply distal portions of the PCL via branches from the inferior genicular and popliteal arteries [12].
Nerve fibers from the popliteal plexus supply the PCL and its synovial sleeve. The popliteal plexus is derived from the posterior articular nerve and the terminal branches of the obturator nerve [13]. The posterior articular nerve branches from the tibial nerve. A histologic study of mechanoreceptors performed by Katonis et al. observed three types of nerve endings in the PCL: Ruffini corpuscles (type I, pressure receptors), Vater–Pacini corpuscles (type II, velocity receptors), and free nerve endings (type IV, pain receptors) [14]. Golgi tendon organlike structures are found in the PCL origins beneath the synovial sheath and likely play a role in proprioceptive function in the knee [13]. Thus, injury to the PCL creates not only a mechanical disturbance but also a neurologic one by severing the afferent signals to the central nervous system [1].
Congenital deficiency of the PCL is described in association with various congenital longitudinal deficiencies of the lower limb. Albeit rare, PCL deficiency is often seen in conjunction with ACL deficiency. Congenital absence of the cruciate ligaments can result in anterior or posterior dislocation, specifically when undergoing surgery for leg length discrepancy. It is not uncommon for these patients to complain of retropatellar pain as they lock the knee in extension to help provide stability during gait increasing patellofemoral pressure during ambulation [15]. Arthroscopy of these patients often finds a shallow, hypoplastic intercondylar notch, poorly developed or absent tibial spines, and in some, fibular hypoplasia. There has not been good evidence to show that agenesis of the cruciate ligaments is associated with changes in the menisci (i.e., meniscal agenesis or discoid meniscus). Chomiak et al. reported there was little clinical significance to the cruciate deficiency in patients with proximal femoral focal deficiency (PFFD) as the majority of patients did not complain of knee instability during normal daily activities. Prevention of posterior dislocations of the knee is recommended in all lengthening procedures in patients with PFFD, as this complication can be anticipated [16].
Radiographic Anatomy
MRI is widely used to image internal derangements of the knee. To obtain good quality images, an appropriately sized field of view should be used to maximize the resolution. The knee should be imaged in three orthogonal planes: axial, coronal, and sagittal planes (Fig. 2.8). On sagittal T2-weighted images, the normal PCL appears as a well-defined uniform band of very low signal intensity. When the knee is in extension, the PCL is lax and it has a gentle posterior convex curvature (Fig. 2.9). The PCL should be found near the midline of the joint in at least 2–3 consecutive images. The meniscofemoral ligaments can often be seen as a small round or oval structure of low signal intensity just anterior or posterior to the PCL. On coronal images, the posterior vertical portion of the PCL is seen in the intercondylar notch, adjacent to the lateral aspect of the medial femoral condyle. The ligament curves forward anteriorly and the horizontal portion appears as a circular or ovoid area of low signal intensity within the intercondylar notch. Axial images are useful in visualizing the vertical portion of the PCL from its tibial insertion [15]. Coronal and axial images can be complementary in the evaluation of the femoral and tibial attachments of the PCL.
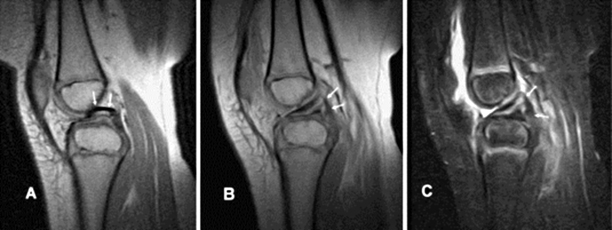
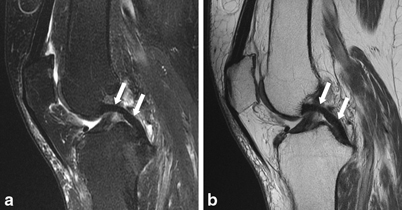
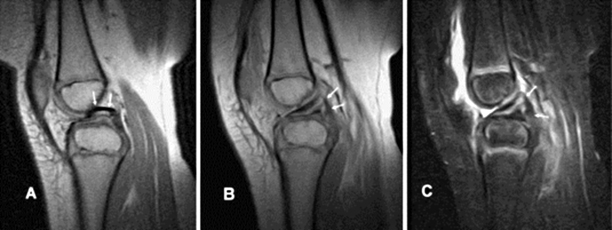
Fig. 2.8
a Normal PCL (arrows) in the left knee on TSE PD sagittal MR image (TR/TE 1840/21 ms). b TSE PD (TR/TE 1840/21 ms) and c fat-suppressed TSE T2-weighted sagittal MR images (TR/TE 4430/27, TI 90 ms) shows a thickened, poorly defined PCL with abnormal internal high signal intensity (arrows) [66]. PCL posterior cruciate ligament, TSE PD turbo spin-echo proton density
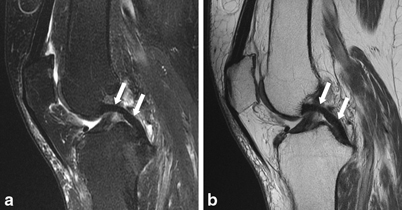
Fig. 2.9
a and b Demonstrate the normal appearance of the PCL (arrows) on T2 and PD images, respectively. Note the normal curved appearance and the homogeneously low signal on both sequences [67]. PCL posterior cruciate ligament, PD proton density
The majority of PCL tears are associated with other injuries due to the high level of force necessary to tear the strong PCL fibers. Tears occur most frequently at the middle portion of the PCL. Uncommonly, the PCL may avulse from its tibial attachment. Injuries are best evaluated using sagittal T2-weighted images. A normal intact PCL is a well-defined continuous band of low signal intensity in all pulse sequences. In a complete tear of the PCL, the fibers are discontinuous, with high-intensity fluid signal completely traversing the fibers (Fig. 2.10). Amorphous high signal intensity would also be seen on T1-weighted imaging. In a partial tear, the PCL is thickened, with an increased signal (Fig. 2.11). Avulsion fracture of the PCL is uncommonly seen but would reveal increased signal on T2-weighted images through the posterior portion of the tibia at the level of the PCL attachment. Common associated injuries with PCL tear include ACL tear, anterior bone contusion, collateral ligament injury, and menisci tears [17]. T1-weighted or short tau inversion recovery (STIR) images are valuable in the assessment of associated bone marrow injury [18]. The sensitivity and specificity for diagnosing a PCL tear by MRI approaches 100 %. MRI has proven to be an accurate modality for evaluating the integrity of the PCL [19].
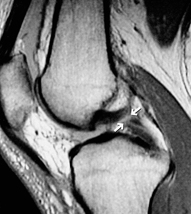
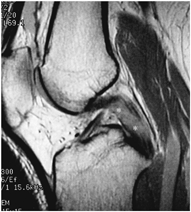
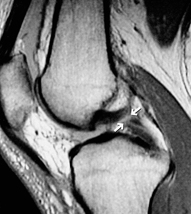
Fig. 2.10
Complete PCL tear. PDWI of a woman in her thirties shows complete tear of PCL at its mid-portion (arrow) [68]. PCL posterior cruciate ligament, PDWI proton-density-weighted image
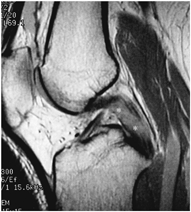
Fig. 2.11
Partial tear of PCL (intrasubstance injury) (1). PDWI of a man in his thirties shows partially torn PCL, which is swollen and shows hyperintensity (*) for the entire length, representing intrasubstance injury. Periphery of the PCL retains continuity of fibers [68]. PCL posterior cruciate ligament, PDWI proton-density-weighted image
Biomechanics
Over the past 30 years, the biomechanical role of the PCL has been investigated by many authors. Cadaveric sectioning studies of the PCL, comparison studies with PCL deficiency in one knee and a normal contralateral knee, as well as contact pressure studies and kinematic analysis have all been performed, yielding insight into the multitude of functions the PCL provides in the normal and pathologic knee. We have found that attempting to understand the literature available is best done by collating the studies based on their findings to specific questions. As such, this chapter has been subdivided into various subtopics of PCL biomechanics, each of which examines the present literature and based upon the best available evidence attempts to draw conclusions. The subdivisions are as follows: posterior tibial translation, rotational and medial/lateral stability, joint contact pressure studies, morphological/meniscal/chondral degeneration, strength, proprioception, and compensatory mechanisms: electromyography and muscle activation.
Posterior Tibial Translation
In vitro studies reaffirm the role the PCL has in preventing posterior tibial translation; however, controversy still exists as to the relative translational stability provided by the PCL at varying angles of knee flexion . Kumagai et al. conducted translational measurements in the anterior to posterior plane on five cadaveric specimens before and after PCL sectioning [20]. They found no difference in posterior translation below 25° of knee flexion, while displacement increased significantly between 25° and 90° of flexion, averaging > 10 mm of posterior translation at 90° of flexion [20]. In contrast, Li et al. examined eight cadaveric knees and found that in PCL-deficient knees, posterior tibial translation only occurred above 60°; this was reaffirmed by Pearsall et al. where eight cadaveric knees were examined with strain gauges in either meniscus to measure strain in the menisci in varying degrees of flexion in the PCL intact, ruptured, and reconstructive states [21, 22]. They found that in scenarios where the PCL was cut, the total anterior–posterior translation was > 18 mm and was statistically significant compared to the intact and reconstructive PCL states at knee flexion angles of 60° and 90° [22].
However, other authors have shown increased posterior tibial displacement in PCL- deficient knees throughout the arc of motion (0–120°) [23–28]. Gollehon et al. published their work in the Journal of Bone and Joint Surgery (JBJS) in 1987 where they tested the static stability of the PCL and the posterolateral corner structures in a sectioning study of 17 human cadaveric knees at knee flexion angles between 0° and 90° [23]. They found that at all angles of flexion, the PCL was the principle restraint to posterior translation and that at all angles tested, isolated sectioning of the PCL did not affect varus or external rotation stability [23]. Similarly, Li et al. examined 12 fresh-frozen cadaveric knees using a robotic testing system and applied a 130 N posteriorly directed load at 30° increments between 0° and 150°; they found statistically significant posterior tibial translation at all knee flexion angles except 150°, indicating the role of the PCL in posterior stability except for at extreme flexion angles [24]. Hagemeister et al. reaffirmed the importance of the PCL in providing posterior stability at low flexion angles when they looked at five pairs of fresh-frozen cadaveric knees with a mean age of 73.2 years and used electromagnetic sensors to effectively examine the translation caused by sectioning of the PCL and posterolateral corner structures [25]. Statistically significant differences in anterior–posterior translation existed at all 15° intervals measured from 0° to 75° [25]. Furthermore, Harner et al. examined the effect of the popliteus tendon in conjunction with the PCL in ten cadaveric specimens and found that with a simulated posterior drawer test of 110 N and a simulated popliteus contraction of 44 N in the PCL-intact knees did not result in increased posterior tibial translation; however, when the PCL was sectioned, not only did the posterior tibial translation increase at knee flexion angles of 0°/30°/60°/75°/90° but also a statistically significant decrease in posterior tibial translation was found with the addition of a simulated popliteus muscle contraction [26]. As such, they concluded that the popliteus muscle is an important stabilizer against posterior tibial translation in the PCL-deficient knee [26]. Grood et al. also looked at the effect of tibial translation at low flexion angles of the knee using 15 whole lower limb cadavera and found that sectioning of the PCL did produce posterior tibial sag even at full extension; however, the posterior translation was most apparent at 90° of flexion (the highest flexion angle they measured) where it averaged 11.4 mm of posterior displacement [27]. Finally, Hoher et al. examined eight cadaveric knees using a universal force-moment sensor (UFS) testing system to apply a 110-N load to the knee at 0°/30°/60°/75°/90° [28]. They found that with the application of the load, even at full extension there was a difference in posterior tibial translation of 3.0 mm, which progressively increased to 14.1 mm at 90° of knee flexion [28].
In addition, in vivo studies examining the posterior tibial translation of PCL -deficient knees also exist. Castle et al. examined posterior tibial translation at varying degrees of knee flexion in ten patients with unilateral PCL deficiency using lateral radiographs [29]. They found that at knee flexion angles between 70 ° and 90°, the mean displacement of the tibia posteriorly was 7.4 mm, while knee flexion angles between 30 ° and 50 ° resulted in a mean displacement of just 2.1 mm [29]. Furthermore, displacement at lower flexion angles was not uniform. Logan et al. also looked at in vivo analysis of the PCL in their series of six patients with unilateral PCL deficiency who underwent MRI evaluation while squatting as well as while a posterior drawer test was performed [30]. Their findings were suggestive of altered kinematics in the medial compartment, with approximately 5 mm of posterior tibial translation measured on the sagittal image throughout all weight-bearing flexion angles [30]. Additionally, in the stress sagittal images obtained at 90° of flexion, a mean of 10.1 mm of posterior tibial translation occurred [30].
Assimilation of the data available in all of these studies would suggest that the PCL is the primary restraint to posterior translation of the knee and that although it likely has an effect throughout the functional arc of motion, its effect is likely greatest at high angles of knee flexion (60–120°). Additionally, the popliteus muscle may act as a restraint to posterior translation in the PCL-deficient knee.
Rotational and Medial/Lateral Stability
The role that the PCL plays in providing rotational stability to the knee is still unclear. Many reports exist throughout the literature that show isolated sectioning of the PCL does not significantly affect tibial external rotation. Gollehon et al. sectioned the PCL in 17 cadaveric specimens and showed that external rotation or varus stress did not increase at any position of knee flexion [23]. Grood et al. performed sectioning of the PCL and posterolateral structures in 15 cadaveric specimens and measured displacement in varying degrees of knee flexion with a electrogoniometer [27]. Their results showed no increased tibial external rotation or varus/valgus angulation compared to the intact state [27]. Nielsen et al. evaluated 25 osteoligamentous-intact cadaveric knees and performed sectioning of the PCL along with the medial and lateral structures [31]. They found that axial rotatory instability was only detectable when the PCL lesion was combined with either a medial or lateral side ligamentous injury; furthermore, a reverse pivot shift was only elicited when the PCL/lateral collateral ligament (LCL) /popliteus tendon were sectioned [31]. Finally, Kaneda et al. looked at 15 cadaveric fresh-frozen knees and performed sequential sectioning of the PCL, LCL, and posterolateral structures and found that in isolated sectioning of the PCL, there was no increase in tibial external rotation; however, they did find that isolated sectioning of just the anterolateral bundle of the PCL shifted the axis of external rotation of the knee [32].
This is in contrast to four other studies, all which showed that isolated sectioning of the PCL does lead to increased tibial rotation. Li et al. examined eight cadaveric knees using a robotic testing system with applied simulated quadriceps and hamstring loads (400 and 200 N, respectively) at knee flexion angles from 0° to 120° and found that at angles above 60° significant external tibial rotation occurred [21]. Harner et al. performed an investigation using ten cadaveric knee specimens where simulated popliteus muscle contraction was performed in both the presence and absence of the PCL [26]. They found that simulated popliteus muscle contraction resulted in an internal tibial rotation of 2° and 4° at 60° and 90° of knee flexion, regardless of whether or not the PCL was intact [26]. Gupte et al. used eight cadaveric knees to evaluate the role of the meniscofemoral ligaments in providing sagittal plane and rotational stability in the posterior cruciate ligament-deficient knee [33]. They found that although isolated sectioning of the PCL does increase rotational instability between 60° and 90°, further sectioning of the meniscofemoral ligaments still does not lead to increased rotational instability [33]. Finally, Ogata et al. performed sequential sectioning of the PCL and collateral ligaments and found increasing posterior sag and internal rotation of the tibia with increasing degrees of knee flexion in specimens in which only the PCL was deficient [34].
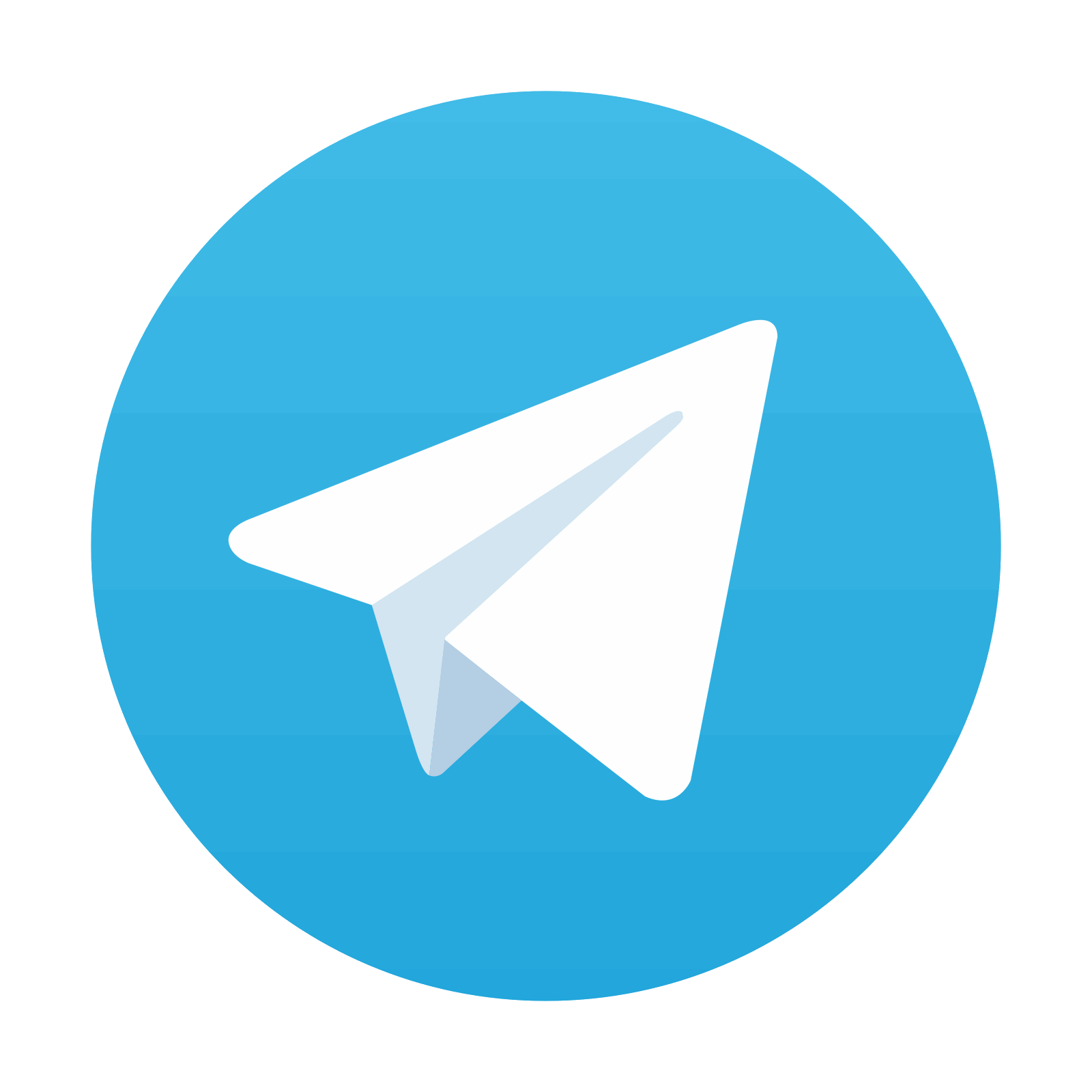
Stay updated, free articles. Join our Telegram channel

Full access? Get Clinical Tree
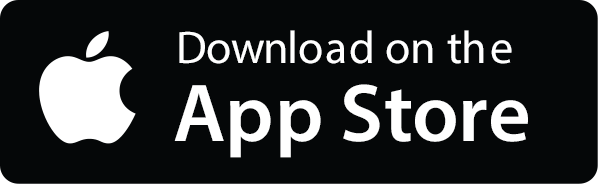
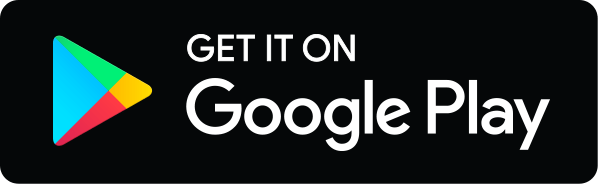