Chapter 1 Anatomy
The anatomy of the knee can be examined on a number of levels from microscopic to gross and with a variety of techniques, including physical examination, anatomic dissection, radiographic and cross-sectional imaging, and arthroscopic examination. Any practitioner interested in diagnosing and treating disorders of the knee must have a detailed understanding of both normal and abnormal regional anatomy. Furthermore, the ability to interpret and correlate information obtained from different sources is highly beneficial. However, it is also paramount that the clinician gain the knowledge required to be able to interpret the significance of an identifiable anatomic abnormality within the context of a patient’s complaints. It is the goal of this chapter to present a thorough review of knee anatomy to help the reader successfully assimilate the material presented in subsequent chapters. To provide a comprehensive description of pertinent anatomic details, text, illustrations, arthroscopic photographs, radiographs, and pictures from cross-sectional imaging studies are used. In addition, in many situations, the same structures are presented from different perspectives. Rather than being redundant, we hope that this approach will facilitate the development of a more complete appreciation of the anatomy about the knee. The descriptions that follow are taken in part from standard anatomic texts.3,10,73,120
Normal Skeletal Structures
Bone Physiology
Bone is composed of mineral crystals embedded in an organic matrix. Of the dry weight of bone (about 10% of the actual weight in situ), approximately 70% is due to mineral content and 30% is organic matter. The mineral consists of primarily calcium and phosphorus in a ratio of 2 : 1. The organic matter is composed of collagen, noncollagenized matrix, and proteins. Collagen is the major extracellular component of bone and is composed of fibrils. Collagen fibrils, which form a parallel, highly organized arrangement, are known as intrinsic fibers, whereas those that tend to anchor ligaments and tendons at attachment sites and often insert in a perpendicular manner are extrinsic fibers. The matrix is populated by mesenchymal cells, which differentiate into osteocytes, osteoblasts, and osteoclasts. These cells perform key functions in the turnover and remodeling of bone in response to both physical and metabolic stimuli. Osteoblasts are cuboid in nature and have abundant cytoplasm. The main function of osteoblasts is to produce osteoid, a collagenized protein that mineralizes at the tidemark zone as hydroxyapatite crystals are incorporated (Fig. 1-1). As the matrix becomes mineralized bone, these cells become embedded and are transformed into osteocytes. The osteocyte is in contact with the osteoblast through the cannular system. Osteoclasts are multinucleated macrophage-like cells that perform bone resorption at mineralized bone surfaces (Fig. 1-2). Other associated tissues such as periosteum (Fig. 1-3), fatty and hematopoietic marrow elements, and tendon and ligament attachments create a complex system with mechanical, metabolic, and hematopoietic functions.
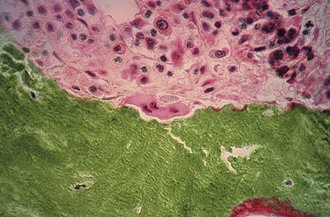
Figure 1-2 Osteoclast. A multinucleated osteoclast is resorbing bone at a crenated surface, Howship’s lacuna.
Bony Architecture
Patella
The patella, the largest sesamoid bone in the body, sits in the femoral trochlea. It is an asymmetrical oval with its apex directed distally. The fibers of the quadriceps tendon envelop it anteriorly and blend with the patellar ligament distally. The articulation between the patella and the femoral trochlea forms the anterior or patellofemoral compartment (Fig. 1-4).
The posterior aspect of the patella is described as possessing seven facets. The medial and lateral facets are divided vertically into approximately equal thirds, whereas the seventh or odd facet lies along the extreme medial border of the patella. Overall, the medial facet is smaller and slightly convex; the lateral facet, which consists of roughly two thirds of the patella, has both a sagittal convexity and a coronal concavity (Fig. 1-5). Six morphologic variants of the patella have been described (Fig. 1-6). Types I and II are stable, whereas the other variants are more likely to give rise to lateral subluxation as a result of unbalanced forces.12,119 The facets are covered by the thickest hyaline cartilage in the body, which may measure up to 6.5 mm in thickness.119 The relationship between surface degeneration of this articular surface, or chondromalacia, seen arthroscopically in adolescents and young adults, and pain is unclear.
The femoral trochlea is separated from the medial and lateral femoral condyles by indistinct ridges; the lateral ridge is more prominent. The patella fits into the trochlea of the femur imperfectly, and the contact patch between the patella and the femur varies with position as the patella sweeps across the femoral surface. The contact patch has been investigated by dye39 and casting techniques.2 Both methods produce very similar results and indicate that the area of contact never exceeds about one third of the total patellar articular surface. At 10 to 20 degrees of flexion, the distal pole of the patella first contacts the trochlea in a narrow band across the medial and lateral facets (Fig. 1-7).39,54 As flexion increases, the contact area moves proximally and laterally. The most extensive contact is made at approximately 45 degrees, where the contact area is an ellipse in continuity across the central portion of the medial and lateral facets. By 90 degrees, the contact area has shifted to the upper part of the medial and lateral patellar facets. With further flexion, the contact area separates into distinct medial and lateral patches.2,39,54 Because the odd facet makes contact with the femur only in extreme flexion (such as in the act of squatting), this facet is habitually a noncontact zone in humans in Western cultures—a fact that is thought to have some pathologic significance.
The main biomechanical function of the patella is to increase the moment arm of the quadriceps mechanism.62 The load across the joint rises as flexion increases, but because the contact area also increases, the higher force is dissipated over a larger area. However, if extension against resistance is performed, the force increases while the contact area shrinks, and this may exacerbate pain from the patellofemoral region. Straight-leg raises eliminate force transmission across the patellofemoral joint because in full extension, the patella has not yet engaged the trochlea.54
Femur
The architecture of the distal end of the femur is complex. Furthermore, this area serves as the attachment site of numerous ligaments and tendons (Fig. 1-8). In shape and dimensions, the femoral condyles are asymmetrical; the larger medial condyle has a more symmetrical curvature. The lateral condyle viewed from the side has a sharply increasing radius of curvature posteriorly. The femoral condyles viewed from the surface, articulating with the tibia, show that the lateral condyle is slightly shorter than the medial. The long axis of the lateral condyle is slightly longer and is placed in a more sagittal plane than the long axis of the medial condyle, which is oriented at a mean angle of about 22 degrees and opened posteriorly.61 The lateral condyle is slightly wider than the medial condyle at the center of the intercondylar notch. Anteriorly, the condyles are separated by a groove known as the femoral trochlea (Fig. 1-9). The sulcus represents the deepest point in the trochlea. Relative to the midplane between the condyles, the sulcus lies slightly laterally.28 Reproducing this anatomic relationship is important for accurate patellofemoral mechanics after total knee replacement.
The intercondylar notch separates the two condyles distally and posteriorly. The lateral wall of the notch has a flat impression, where the proximal origin of the anterior cruciate ligament (ACL) arises. On the medial wall of the notch is a larger site, where the posterior cruciate ligament (PCL) originates. The mean width of the notch is narrowest at the distal end and widens proximally (1.8 to 2.3 cm); in contrast, the height of the notch is greatest at the midportion (2.4 cm) and decreases proximally (1.3 cm) and distally (1.8 cm).68 The dimensions of the notch have become an important topic because of the association between narrow notch width and increased risk for ACL tear. This risk does not seem to be related to the intrinsic characteristics of the ACL because normal-size ligaments have been identified in specimens with narrow notches.87 Therefore, the increased risk of ACL failure is probably due to impingement on the ligament.38,70,87 Notchplasty or sculpting of the intercondylar notch to increase the dimensions has become an integral part of ACL reconstruction.
The lateral condyle has a short groove just proximal to the articular margin, in which lies the tendinous origin of the popliteus muscle. This groove separates the lateral epicondyle from the joint line. The lateral epicondyle is a small but distinct prominence to which the lateral (fibular) collateral ligament (LCL) is attached. On the medial condyle, the prominent adductor tubercle is the insertion site of the adductor magnus. The medial epicondyle lies anterior and distal to the adductor tubercle and is a C-shaped ridge with a central depression or sulcus (Fig. 1-10). Rather than originating from the ridge, the medial collateral ligament (MCL) originates from the sulcus. The epicondylar axis passes through the center of the sulcus of the medial epicondyle and the prominence of the lateral epicondyle (Fig. 1-11). This line serves as an important reference line in total knee replacement. In relation to a line tangent to the posterior femoral condyles, the epicondylar axis is externally rotated about 3.5 degrees in males and 1 degree in females with normal knees.13 In patients with osteoarthritis and valgus knee alignment, the transepicondylar axis has been shown to be externally rotated up to 10 degrees relative to the posterior condylar line.41
In recent years, important anatomic variations in the morphology of the distal femur have been identified in males and females and in different racial groups. Measurements of the distal femur in both Asian and Caucasian populations suggest that women have narrower femurs in the medial-lateral dimension than males, for any given anterior-posterior dimension.14,19,21,48,77 This concept has been defined in terms of the aspect ratio of the distal femur, where the medial-lateral width is divided by the anterior-posterior dimension × 100.19,48 In females, the aspect ratio tends to be smaller than in males in both Asian and white populations.19,48 However, this aspect ratio is also affected by race, with Japanese females displaying a greater medial-lateral width than white females for any given anterior-posterior dimension.110 In addition to these findings, racial differences appear to exist in the rotational anatomy of the distal femur, with more natural external rotation of the transepicondylar axis versus the posterior condylar line in Asian populations.122 These racial differences and sexual dimorphism among humans may have significant implications for both prosthesis development and surgical technique in total knee arthroplasty.94 This information has stimulated vigorous debate regarding whether gender-specific femoral components and knee prostheses designed for different racial groups are needed.* In particular, implants with a narrower medial-lateral geometry for a given specific anterior-posterior dimension have been advocated by some for use in females.40,48,77 A similar prosthesis has been suggested for use in Indian populations, among which greater variability in medial-lateral width for any given anterior-posterior dimension has been noted.112 Although a gender bias has been demonstrated for some contemporary knee prostheses, it is unclear whether this bias has adversely affected the outcomes of total knee arthroplasty in females versus males.29,48,84,85
Tibia
In a macerated skeleton, inspection of the tibial plateau suggests that the femoral and tibial surfaces do not conform at all. The larger medial tibial plateau is nearly flat and has a squared-off posterior aspect that is distinct on a lateral radiograph.25 In distinction, the articular surface of the narrower lateral plateau borders on convexity. Both surfaces have a posterior inclination of approximately 10 degrees with respect to the shaft of the tibia. However, lack of conformity between the femoral and tibial articular surfaces is more apparent than real. In an intact knee, the menisci enlarge the contact area considerably and increase the conformity of the joint surfaces. As previously noted for the femur, gender and race appear to affect the morphology of the proximal tibia.48,69 Again, this may have implications for prosthesis design in total knee arthroplasty.48,69 However, because flexibility in coverage and position of the tibial component is generally greater on the prepared surface of the tibial plateau in total knee arthroplasty, the implications of this variability have not been well researched at this time.
The median portion of the tibia between the plateaus is occupied by an eminence: the spine of the tibia. Anteriorly a depression is seen—the anterior intercondylar fossa—to which, from anterior to posterior, the anterior horn of the medial meniscus, the ACL, and the anterior horn of the lateral meniscus are attached. Behind this region are two elevations: the medial and lateral tubercles. They are divided by a gutter-like depression: the intertubercular sulcus. On an anteroposterior radiograph, the medial tubercle usually projects more superiorly than the lateral tubercle; on a lateral radiograph, the medial tubercle is located anterior to the lateral tubercle (Fig. 1-12). The tubercles do not function as attachment sites for the cruciate ligaments or menisci but may act as side-to-side stabilizers by projecting toward the inner sides of the femoral condyles. In concert with the menisci, the tibial spine enhances the impression of cupping seen in intact specimens. In the posterior intercondylar fossa, behind the tubercles, the lateral and then the medial menisci are attached anteriorly to posteriorly. Most posteriorly, the PCL inserts on the margin of the tibia between the condyles. On the anterior aspect of the tibia, the tuberosity is the most prominent feature and is the attachment site of the patellar tendon. Approximately 2 to 3 cm lateral to the tibial tubercles is Gerdy’s tubercle, which is the insertion site of the iliotibial band (ITB).
Hyaline/Articular Cartilage
Articular cartilage is a specialized connective tissue composed of hydrated proteoglycans within a matrix of collagen fibrils. Proteoglycans are complex glycoproteins consisting of a central protein core to which glycosaminoglycan chains are attached. The structure of hyaline cartilage is not uniform, but rather can be divided into distinct zones based on the arrangement of the collagen fibrils and the distribution of chondrocytes. The density of chondrocytes is highest close to subchondral bone and decreases toward the articular surface (Fig. 1-13). Calcification occurs in a distinct basophilic zone at the deepest level of chondrocyte proliferation termed the tidemark. Beneath this region is a zone of calcified cartilage that anchors the cartilage to the subchondral plate. Cartilage is avascular, and chondrocytes in the superficial zones are believed to derive nutrition from synovial fluid. Deeper zones probably obtain nutrition from subchondral bone.
Examination of gross specimens or arthroscopic visualization reveals normal cartilage to consist of a white, smooth, firm material. Articular cartilage damage or degeneration, termed chondromalacia, can be readily identified (Fig. 1-14). These characteristic changes seen during arthroscopic examination have been classified by Outerbridge90: Grade 0 is normal, white-appearing cartilage; grade I is swelling or softening of an intact cartilage surface; grade II is represented by fissuring and fibrillation over a small area (<0.5 inch); grade III includes the same pathologic changes over a larger area (>0.5 inch); and grade IV changes represent erosion to subchondral bone and are indistinguishable from osteoarthritis. Chondral flap tears caused by delamination of the articular cartilage may also be encountered (Fig. 1-15). These changes in articular cartilage cannot be directly visualized on conventional radiographs but may be seen on magnetic resonance imaging (MRI) studies. However, even MRI is unreliable for detecting early stages of chondromalacia, which may appear as foci or areas of diffuse abnormal signal with a normal surface. Grade III or IV chondromalacia is visible as thinning, irregularity, and fissuring of cartilage (Fig. 1-16).
Damage to the articular cartilage and joint surface may result indirectly from pathologic changes in subchondral bone. Both osteonecrosis and osteochondritis dissecans (OCD) may lead to destruction of the articular surface. In the knee, OCD tends to occur on the intercondylar aspect of the medial femoral condyle in young people. These lesions may separate from the surface and form a loose body. The base of these lesions, if débrided, will reveal vascular subchondral bone (Fig. 1-17). Classic radiographic findings include a lucent osseous defect that may have a fragmented or corticated osseous density within the lucency (Fig. 1-18). On MRI studies, increased signal about the defect on T2-weighted images represents joint fluid surrounding the lesion; irregularity of the articular surface may also be noted (Fig. 1-19). Osteonecrosis results in a similar osteochondral fragment but tends to occur in elderly patients on the weight-bearing aspect of the medial femoral condyle (Fig. 1-20). In distinction to the lesions in OCD, fragments in osteonecrosis separate from a bed of avascular bone (Fig. 1-21). Again, radiographs may reveal a lucent defect at the involved site, but MRI is more reliable for evaluation of these defects (Fig. 1-22). A curvilinear area of low signal with variable bone edema is characteristic. Although the articular cartilage is initially normal, both processes may lead to detachment of osteochondral loose bodies, fragmentation, and collapse of the articular surface with resultant degenerative changes.
Menisci
The menisci are two crescentic fibrocartilage structures that serve to deepen the articular surfaces of the tibia for reception of the femoral condyles (Fig. 1-23). The most abundant components of the menisci include collagen (75%) and noncollagenized proteins (8% to 13%). Glycosaminoglycans and glycoproteins are also key constituents. Although four main types of collagen are present in the menisci, type I collagen is the predominant component and accounts for about 90% of the total collagen. Histologic examination reveals a population of fibroblasts and fibrocartilaginous cells dispersed in an organized matrix of eosinophilic collagen fibrils. The collagen bundles are arranged in a circumferential pattern that is optimal for absorption of compressive loads (Fig. 1-24). Radial fibers found at the surface and in the midsubstance parallel to the plateau may act to increase structural rigidity and help prevent longitudinal splitting.96 Elastin fibers, which constitute approximately 0.6% of the dry weight of the meniscus, seem to help in recoil to the original shape after deformation.113 In degenerative menisci, metaplasia of the cell population occurs with a trend toward chondroid cell appearance (Fig. 1-25).
Each meniscus covers approximately the peripheral two thirds of the corresponding articular surface of the tibia. The peripheral border of each meniscus is thick, convex, and attached to the capsule of the joint; the opposite border tapers to a thin, free edge. The proximal surfaces of the menisci are concave and in contact with the femoral condyles; the distal surfaces are flat and rest on the tibial plateau. On MRI studies, normal menisci are best seen on sagittal views and have low-signal characteristics with no or little internal signal. The posterior horn of the medial meniscus is larger than the anterior horn, whereas the anterior and posterior horns of the lateral menisci are typically of similar size (Fig. 1-26). Increased signal within the menisci may be noted and classified on a scale ranging from I to III. Patchy areas of increased signal that do not touch the inferior and superior borders of the menisci represent grade I changes. Grade II changes typically have a linear configuration, but again they do not touch the superior and inferior surfaces. These signal changes probably represent the normal aging process in the menisci. Increased signal with a linear appearance that contacts one of the articular surfaces of the menisci is classified as grade III change and represents a true meniscal tear (Fig. 1-27).78,105 A variety of meniscal tears may be identified on MRI but are best delineated by arthroscopic examination (Fig. 1-28). Patterns include vertical and horizontal cleavage tears, radial tears, bucket handle tears (detachment of the body of the menisci at the periphery with intact anterior and posterior horn attachments), and complex degenerative tears (Fig. 1-29). The technique of arthroscopic repair and partial meniscectomy has superseded open meniscectomy; therefore, examination of intact resected specimens is rarely possible (Fig. 1-30).
Calcification may occur within the fibrocartilage of the menisci and is referred to as chondrocalcinosis. This abnormality has classically been described in association with calcium pyrophosphate dihydrate deposition disease. However, chondrocalcinosis may be noted incidentally on radiographs or during arthroscopic examination (Fig. 1-31).
The menisci perform several important functions, including (1) load transmission across the joint, (2) enhancement of articular conformity, (3) distribution of synovial fluid across the articular surface, and (4) prevention of soft tissue impingement during joint motion. The medial meniscus also confers some stability to the joint in the presence of ACL insufficiency, in that the posterior horn acts as a wedge to help reduce anterior tibial translation.76 However, the lateral meniscus does not perform a similar function.75 The rapid progression of degenerative changes, first observed by Fairbank, that occur as a result of complete meniscectomy have been well documented.30 These changes include (1) osteophyte formation on the femoral condyle projecting over the site of meniscectomy, (2) flattening of the femoral condyle, and (3) narrowing of joint space in the involved compartment.
Medial Meniscus
The medial meniscus is nearly semicircular in form and measures about 3.5 cm in length. It has a triangular cross-section and is asymmetrical, with a considerably wider posterior than anterior horn. The attachment of the posterior horn, the so-called meniscal root, is firmly attached to the posterior portion of the intercondylar fossa of the tibia, directly anterior to the PCL insertion (Fig. 1-32). The functional importance of the meniscal root has become better appreciated over the past 5 years. Tears of the meniscal root destabilize the meniscus and are associated with meniscal extrusion on MRI.74 As a result, it has been theorized that root tears appear to produce the same functional changes as total medial meniscectomy.4,46,74,82 This is believed to be a significant risk factor in the development of early osteoarthritic changes.4,74 In biomechanical studies, evidence to support the MRI findings has been forthcoming, with data showing increased joint contact pressures and altered knee kinematics; indeed, these changes are similar to those seen after total meniscectomy that have been associated with subsequent articular cartilage damage and osteoarthritic changes.4,82 It is important to note that repair of a root tear appears to improve the function of the meniscus and may reduce the risk of early osteoarthritic changes.4,46,82
The anterior attachment of the meniscus is more variable; usually, it is firmly attached to the anterior intercondylar fossa approximately 7 mm anterior to the anterior margin of the ACL insertion, in line with the medial tibial tubercle, but this attachment can be flimsy.56 Also, a fibrous band of variable thickness, the transverse intermeniscal ligament, connects the anterior horn of the medial meniscus with the lateral meniscus (Fig. 1-33). Peripherally, the medial meniscus is continuously attached to the capsule of the knee. The midpoint of the medial meniscus is more firmly attached to the femur via a condensation in the capsule known as the deep medial ligament (Fig. 1-34). The tibial attachment of the meniscus, sometimes known as the coronary ligament, attaches to the tibial margin a few millimeters distal to the articular surface, where it gives rise to a synovial recess. Posteromedially, according to Kaplan, the meniscus receives a portion of the insertion of the semimembranosus via the capsule.61
Lateral Meniscus
In contrast to the C-shaped medial meniscus, the lateral meniscus is nearly circular and covers a larger portion of the articular surface (see Fig. 1-32). The anterior horn is attached to the intercondylar fossa, directly anterior to the lateral tibial tubercle and adjacent to the ACL. The posterior horn is attached to the intercondylar fossa directly posterior to the lateral tibial tubercle and adjacent and anterior to the posterior horn of the medial meniscus.56 Somewhat variable fibrous bands, the meniscofemoral ligaments, connect the posterior horn of the lateral meniscus to the intercondylar wall of the medial femoral condyle. These meniscofemoral ligaments, which embrace the PCL, are also known by the eponyms Humphry and Wrisberg (Fig. 1-35). The ligament of Humphry passes anterior to the PCL, whereas the ligament of Wrisberg passes posterior to the PCL (Fig. 1-36). One or the other of these meniscofemoral ligaments has been identified in 71% to 100% of cadaver knees; the ligament of Wrisberg is a more constant finding, and both ligaments together are found in only a small percentage of specimens.96,111,114 Meniscofemoral ligaments running from the anterior horns of the medial and lateral menisci to the intercondylar notch anterior to the ACL have also been identified. Wan and Felle114 reported a 15% incidence of both of these structures in 60 cadaver knees, and one or the other was present in 25% of specimens. In general, the ligaments of Wrisberg and Humphry were much more robust structures than either of the meniscofemoral ligaments originating from the anterior horns.
The peripheral capsular attachment of the medial meniscus is continuous, but the attachment of the lateral meniscus is interrupted by the popliteal hiatus, through which passes the popliteal tendon (Fig. 1-37). In addition, unlike the anatomy on the medial side, the lateral meniscus does not have a direct attachment to the collateral ligament. Posterolaterally at the popliteal hiatus, the lateral meniscus is grooved by the popliteal tendon. Some fibers of the tendon insert into the periphery and superior border of the meniscus at this site.62,63 Because the lateral meniscus is not as extensively attached to the capsule as the medial meniscus is, it is more mobile and may displace up to 1 cm. The controlled mobility of the lateral meniscus, which is guided by the popliteal tendon and meniscofemoral ligament attachments, may explain why meniscal injuries occur less frequently on the lateral side.71,72 Although the meniscofemoral ligaments appear to perform an important function, little is known about the significance of injury to these structures.
Synovial Cavity
Synovium is normally a smooth, translucent pink tissue. Histologically, a thin layer of synovial cells, or synoviocytes, is found at the surface (Fig. 1-38). The synoviocytes consist of two cell populations, broadly classified into those that have macrophage-type function and those with a synthetic function. Type 1 cells contain numerous mitochondria, lysosomes, phagosomes, and surface undulations indicative of their macrophage function. Type 2 cells have rough endoplasmic reticulum and free ribosomes characteristic of secretory cells. This layer of cells, the intimal layer, lies above a fibrovascular zone, the subintimal layer, which contains arterioles, fat, and a variety of connective tissue cells, including fibroblasts and histiocytes. The fibrovascular zone gradually becomes more fibrous at capsular insertions. In specific disease processes, including rheumatoid arthritis, the synovium becomes hypertrophic and inflamed and contributes to intra-articular destruction (Fig. 1-39).
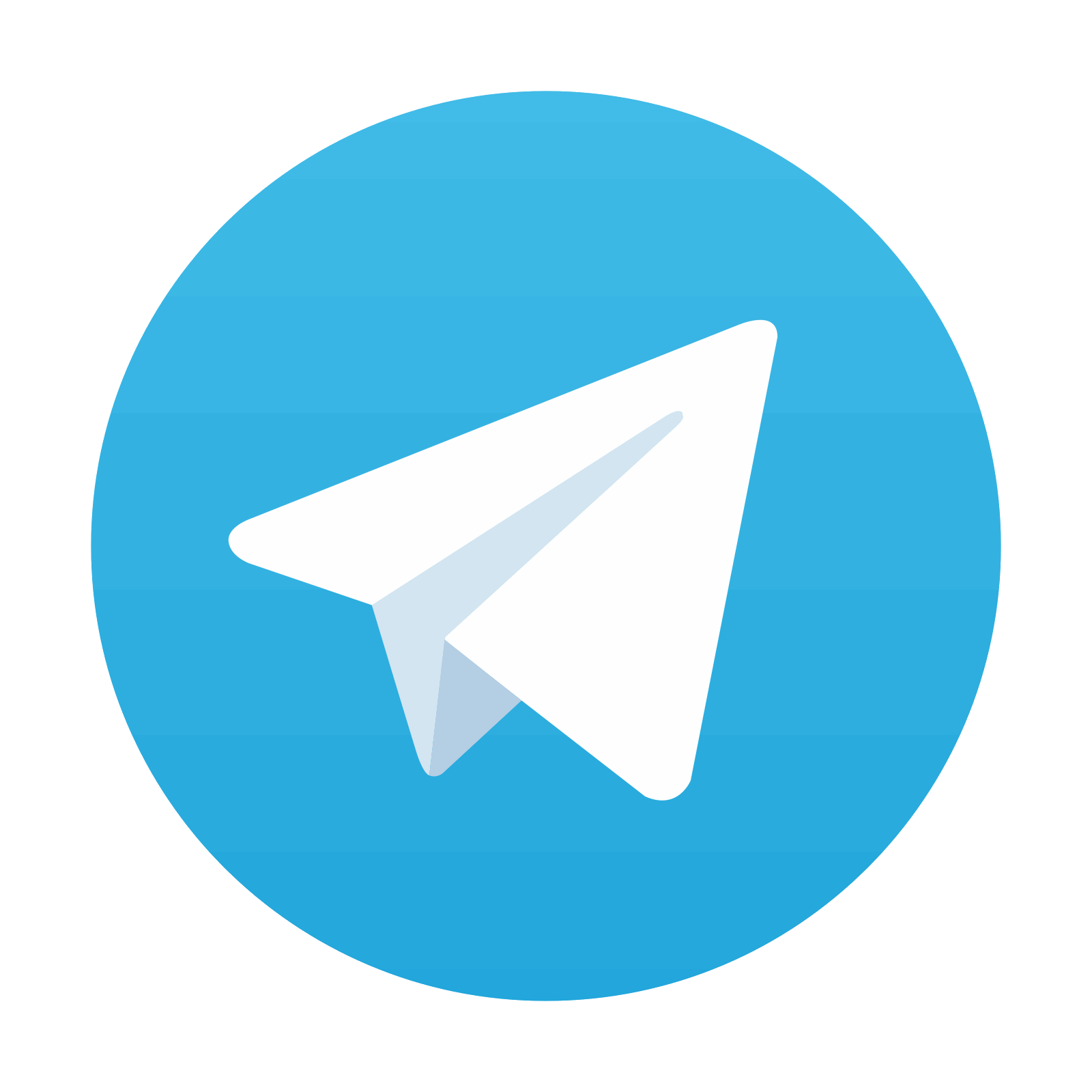
Stay updated, free articles. Join our Telegram channel

Full access? Get Clinical Tree
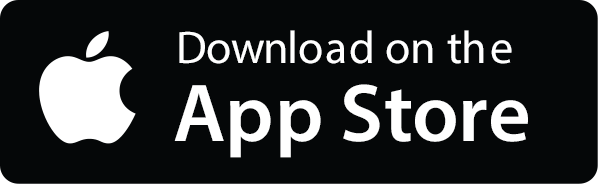
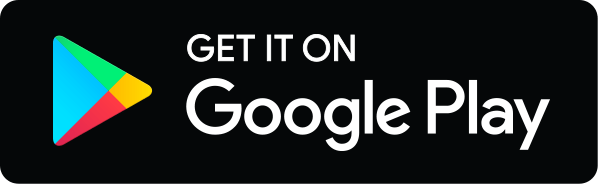