Dominant axis
a w [m/s2]
MTVV z [m/s2]
MTVV/a w
VDV z [m/s1.75]
Kitesurf
Z
5.5
25
4.5
65 (1 h)
Alpine ski
Z
8.9
100
11
95 (2 h)
Snowboard
Z
6.1
250
41
210 (2 h)
Results outline that the dominant vibration is always the vertical one; the weighted accelerations are at least 5 times larger than the limits of the EU legislation. The MTVV/a w ratio always exceeds the ratio 1.5 suggested by the standard (values between 4.5 and 41), outlining the presence of impulsive events (jumps in snowboarding and kitesurfing, snow irregularities in skiing). Also the vibration dose value (VDV) largely exceeds the EU regulation limit (21 m/s1.75).
Additional analyses proved that the vibration distribution at the feet is not uniform. In kitesurfing, the low-frequency roll, pitch and yaw of the board (whose behaviour is rigid in the frequency range of interest for WBV) entail a different exposure of the bow and stern feet. The stern (flexed) leg mainly supports the kitesurfer weight; at the rear pad location, the average vibration level of the table is relatively low (2.39 m/s2). The bow (straight) leg does not support the static weight of the subject, but the vibration level at the bow pad is larger than 15 m/s2 at the forefoot. The centre of rotation of the board is behind the stern leg, and consequently the two legs move in phase. At the current state of the art, it is not possible to predict the stress on the intervertebral discs, but the studies of Rohlman et al. [36] indicated the importance of the phase difference between the vibrations at the feet on the bending moments on the vertebrae.
In alpine ski, the vibration exposure of the feet is symmetrical, given that no differences were noticed between the left and right ski (differences smaller than 0.2 m/s2). Nevertheless, during turns, both the vibration exposure and load on the 2 feet are different (the majority of the load is carried by the internal ski). The vibration at the rearfoot was averagely 20 % larger than that at the forefoot. The acceleration, measured in proximity of the ski binding, outlined that the motion is the superposition of a vertical vibration and a rotation along the Y (lateral) axis. The rotation leads to a fore-and-aft motion of the upper part of the body.
In snowboarding, the vibration on the fore leg is larger than that on the aft leg [37]. The vibration level increases with the speed [12] and depends on the snow conditions [37]. The vibration during the turns is 3–5 times larger than the vibration in straight trajectories. The maximum transient vibration level was the largest among the investigated conditions and was reached in jumps and other evolutions in a snow park.
Given that the vibration transmissibility between the body segments depends on the frequency, the spectral analysis adds useful information to the mere vibration levels presented in the previous section. The vibration at the feet does not show dominant frequency components: the averaged spectra in Fig. 30.1 summarize 30 min of measurements in alpine skiing, snowboarding and kitesurfing. Data are averaged in order to obtain a frequency resolution of 1 Hz; in case of alpine ski and snowboard, data are the average of the four vertical accelerometers placed at the tip and at the toe of the 2 feet; in case of kitesurf, the acceleration is directly measured on the central part of the board.
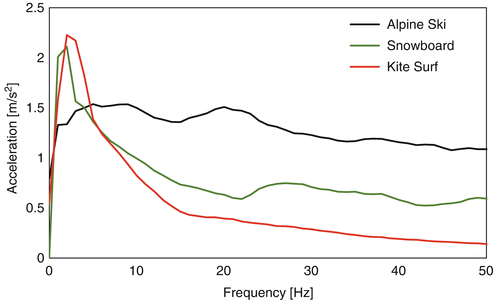
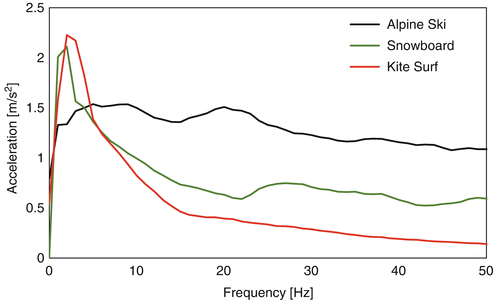
Fig. 30.1
Averaged vibration spectra (Z axis) in skiing, snowboarding and kitesurfing
The vibration spectrum in alpine skiing ranges between 1 and 1.5 m/s2 up to 50 Hz. The vibration spectra in kitesurfing and snowboarding decrease above 5 Hz, reasonably because of the larger compliance of the boards with respect of the ski. As outlined in the literature [38, 39], the effect of the snow and water conditions on the vibration is important, but is generally low in comparison with the effect of the speed.
30.3 Biomechanical Response
The biomechanical response of the human body to vibration is commonly expressed by the vibration transmissibility and by the apparent mass. The vibration transmissibility is the frequency response function between the vibrations at two positions (the stimulus is often at the driving point, and the response location varies depending on the application). The apparent mass is defined as the ratio between the inertial force that a subject transmits to the supporting interface and the acceleration of the interface itself.
The biomechanical responses of standing subjects (apparent mass and transmissibility) have been extensively studied with subjects standing over rigid plates with barefoot or shoes. Nevertheless, the sport equipment can modify both the vibration transmissibility along the human body and the apparent mass. To our knowledge, there are only two studies [38, 39] focused on the identification of the vibration transmissibility using sports equipment.
The vibration transmissibility in kitesurfing has been studied in Ref. [39] with two subjects (body masses 64 and 80 kg, 1.72 and 1.78 m tall, respectively) standing on two different boards. The transmission of vibration to the body is affected by the presence of the board pads; consequently, studies were performed to evaluate the transmissibility from the board to the heel. Results (summarized in Fig. 30.2) showed that the transmissibility in kitesurfing posture (weight on the stern leg) is different from the one of subjects standing on the board in a balanced position. The mechanisms of vibration transmission to the remaining parts of the body are therefore expected to be different from those of the standing subjects.
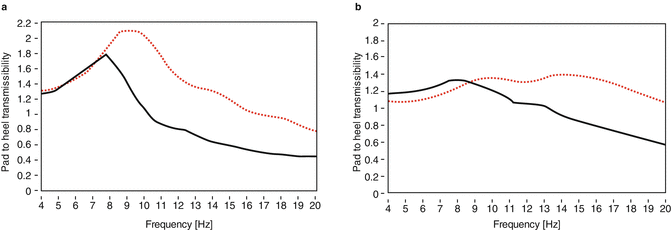
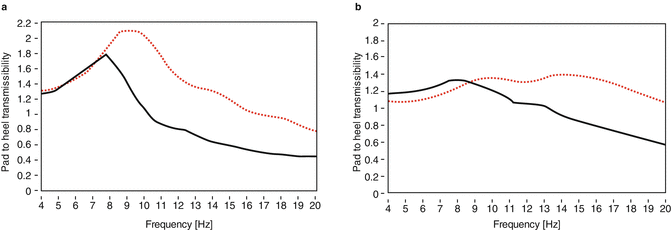
Fig. 30.2
Vibration transmissibility from the kitesurf board to the subject heel on two different boards (plots a and b) and in different postures (black line: standing posture, red dotted line: kitesurf posture)
A similar issue was evidenced for alpine skiing, in the analysis of vibration transmissibility from the supporting surface to the third lumbar vertebra with and without the skis [39]. Results (Fig. 30.3 ) show that the ski boots increase the amplification of the main resonance of the upper body from 2 to 2.5; the amplification at the second resonance frequency decreases from 1 to 0.35. Also in this case, the mechanisms of vibration transmission are expected to be totally different from those of standing subjects.
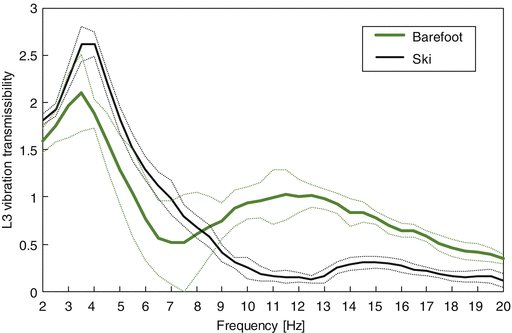
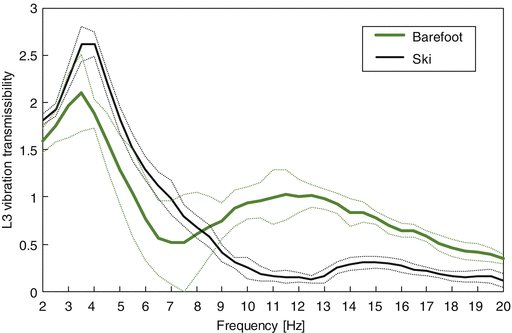
Fig. 30.3
Effect of the skis on the transmissibility between the feet and the L3 lumbar vertebra
30.4 Discussion and Conclusions
To date, the effect of whole-body vibration on the aetiology of back pathologies in extreme sports is not clear. WBV has documented positive effects: it improves the muscle strength, the bone density, the blood flow and the mobility [32]. Nevertheless, the continuous exposure to WBV increases the possibility of back degenerative pathologies [2–7] mainly because of the stress induced by the acceleration on the spine. A weak correlation between the vibration amplitude and the stress on the intervertebral discs has been proven by the studies performed by Rohlman et al., in vivo [31], and the extremely large vibration levels experienced by kitesurfers, snowboarders and alpine skiers have been documented by Tarabini et al. [12]. The stress on the spine is surely affected by the particular postures assumed in the extreme sports. The knee flexion, in particular, largely reduces the amount of vibration reaching the trunk, but the adoption of a nonsymmetrical posture, paired with tilting motion of the feet supports, induces bending moment on the spine that is balanced with an increase of compression on one part of the intervertebral disc.
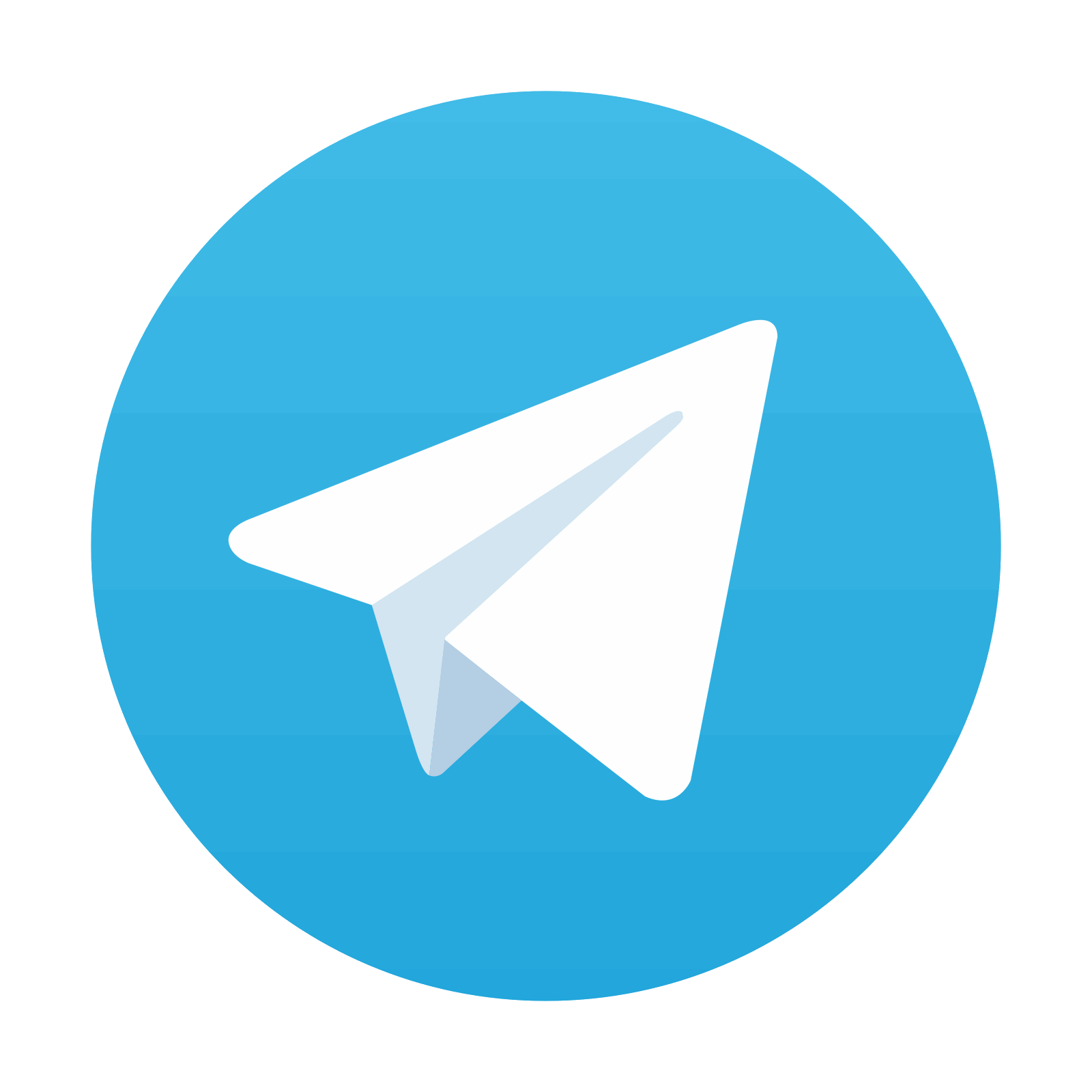
Stay updated, free articles. Join our Telegram channel

Full access? Get Clinical Tree
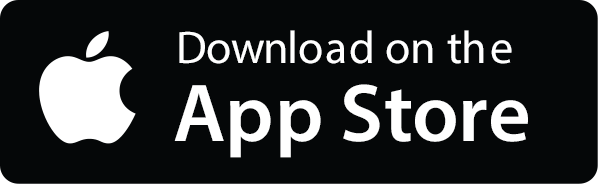
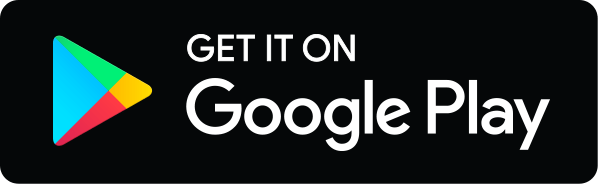