where
S = rate of body heat storage
M = rate of metabolic energy (heat) production
W = external mechanical force production (concentric, eccentric, isometric in isolation or combination)
R + C = rate of radiant and convective energy exchanges
K = rate of conduction (important when in water)
E = evaporative losses
The sum of this equation represents heat gain if positive and heat loss if negative, such that body temperature increases when S is positive, decreases when S is negative and remains the same when S is zero [11].
31.3.1 Neurological and Thermoregulatory Responses to Cold Stress
To regulate body temperature within a narrow range, humans combine behavioural and autonomic physiological processes [11]. Behavioural modifications during sport in cold environments are limited by the intensity of exercise or task and addition of clothing layers, whilst autonomic physiological modifications include decreased skin blood flow via cutaneous vasoconstriction, catecholamine secretion and shivering and non-shivering thermogenesis [12]. Autonomic reflexes are proposed to be under the control of a central integrator, presumed to be the preoptic anterior hypothalamus (POAH). The POAH receives information relayed from afferent thermoreceptors in the skin [13, 14], core [10] and brain [15]. Most skin thermosensors are cold sensitive, and sensory information from the skin triggers cold defence mechanisms [16–19]. When ambient temperatures drop below ~26 °C, cold-sensitive thermoreceptors [20] are activated and send projections via polysynaptic pathways to the POAH. The level of activation is referenced to an activation (temperature) ‘set point’, and when body temperature breaches a threshold from the ‘set point’, synaptic activation of cold-sensitive neurons is proposed to trigger autonomic heat gain reflexes.
The initial response to cold stress is a decrease in peripheral blood flow, primarily to the skin vasculature but also some peripheral regions of skeletal muscle. The response reduces heat loss via convection from the body core to the shell enabling the skin and subcutaneous fat to act as an insulative layer. As heat is lost from the skin surface but not replaced by blood flow, it is manifested as a decline in skin temperature. Vasoconstriction occurs when skin temperature drops below thermoneutral (34 °C) as cold-sensitive thermoreceptors in the skin are rapidly activated. Maximal vasoconstriction is environment specific and occurs at a higher skin temperature (31 °C) in whole-body water immersion and lower (26–28 °C) during local cooling. Thus, the initial vasoconstrictive response is an effective cold defence mechanism but is at the detriment to blood pressure regulation, skin temperature and in particular muscle temperature.
Typical intramuscular temperature under thermoneutral conditions is around 35 °C, and within the region of 27–35 °C, there appears to be no substantial effects on muscular force production [6]. However, at temperatures of 20 °C and below, muscle force is substantially impaired. Several methodological issues limit the direct comparison of research on muscle cooling and force production [21], but the general consensus is that muscle force production is not substantially impaired until muscle temperature drops below 27 °C [21, 22]. It is worth noting that most of the studies investigating muscle temperature have used local passive cooling designs and as such the results might not extend to whole-body cooling during exercise as often occurs in extreme environments. Nevertheless cold muscles impair force production and performance and increase the risk of hypothermia. It is likely that the reduced ability to produce force is due to a combination of reduced metabolic rate [21], impaired kinetics of muscle fibre action potentials, reduced actomyosin ATPase activity and calcium release from the sarcoplasmic reticulum, thus restricting cross-bridge cycling [21]. Reduced force production and muscular co-ordination is of particular concern in extreme sports. A reduction in dexterity during sports such as climbing can impair performance and increase risk of injury, whilst individuals who compete in water sports or in arctic competitions might find it difficult to perform fine motor skills. Moreover in life-threatening situations impaired dexterity can increase time to take control measures such as opening kit bags, layering on more clothes, using first-aid equipment and operating technical equipment (e.g. radios). In situations that cause rapid reductions in muscle temperature but full consciousness/awareness such as whole-body cold water immersion, decisions to perform activity (such as swimming to safety) might accelerate exhaustion due to ineffective muscle activity prior to reaching critical hypothermia – in these instances exhaustion can cause drowning and might be the primary risk to survival.
31.3.2 Thermoregulatory Responses to Cold Stress
The physiological challenges induced by reductions in core temperature are listed in Table 31.1. Humans generally tolerate reductions in body temperature around 2 °C before maximal shivering occurs. Thereafter, reductions in body temperature continue despite shivering and might cause amnesia and poor judgement (such as not taking shelter) before cardiac arrhythmias and unconsciousness occur around 30 °C.
Table. 31.1
Typical physiological responses to decreases in core temperature
Stage | Core temperature (°C) | Physiological response |
---|---|---|
Normothermia | 37 | |
Mild hypothermia | 35 | Maximal shivering |
Increased blood pressure | ||
34 | Amnesia | |
Dysarthria | ||
Poor judgement | ||
Behaviour change | ||
33 | Ataxia | |
Apathy | ||
Moderate hypothermia | 32 | Stupor |
31 | Shivering ceases | |
Pupils dilate | ||
30 | Cardiac arrhythmias | |
Decreased cardiac output | ||
29 | Unconsciousness | |
Severe hypothermia | 28 | Ventricular fibrillation |
Likely hypoventilation | ||
27 | Loss of reflexes and voluntary motion | |
26 | Acid-base disturbances | |
No response to pain | ||
25 | Reduced cerebral blood flow | |
24 | Hypotension | |
Bradycardia | ||
Pulmonary edema | ||
23 | No corneal reflexes | |
Areflexia | ||
19 | Electroencephalographic silence | |
18 | Asystole | |
15.2 | Lowest infant survival from accidental hypothermia | |
13.7 | Lowest adult survival from accidental hypothermia |
31.4 Predisposing Factors for Cold Strain
Physiological responses to cold exposure might vary between individuals because of sex differences, anthropometrics, fitness and level of acclimatisation to the particular environmental condition. For example, individuals who have a large body surface area, are lean and have a small body mass (ectomorphic) tend to lose body heat content quickly in low ambient or water temperatures when metabolic heat production is low [24, 25]. This is because there is a large skin surface area for convective heat exchange to occur. The opposite is true for endomorphs; the added thermal resistance of adipose tissue lends well to heat conservation [26], and there is evidence to suggest that individuals with body fat percentages greater than 25 % have a higher threshold for vasoconstriction enabling them to limit heat loss [27]. When compared to men of comparable age and body mass, generally women have more body fat content, greater subcutaneous fat content, less muscle mass, higher surface to mass ratio and are more resistant to cold stress. However, when matched for body fatness, there are little gender differences [28], and in most cases women have a greater surface area but smaller body mass and musculature than men placing them at a greater risk of rapid declines in body temperature. These anthropometric characteristics are similar to that of children who have less subcutaneous fat compared to adults thus tend to lose body heat content faster [25] despite more pronounced vasoconstrictor and metabolic responses compared to men [29]. Conversely, populations of advanced age (>60 years) exhibit impaired thermosensitivity, vasoconstriction and heat conservation [30–33] and because of a generally lower level of physical fitness produce less metabolic heat during activity that might lead to a more pronounced rate of heat loss when compared to young healthy counterparts. However, in younger adults physical fitness appears to have a minor influence on thermoregulatory responses to cold. Cutaneous vasoconstrictor activity might be upregulated after endurance training [34] but with little impact on maintenance of core temperature. Resistance-trained athletes might have higher metabolic heat production due to greater muscle mass, allowing them to produce more body heat in the cold [26]. The human adaptive response to repeated cold stress is likely individual specific and is modest compared to heat stress [35]. It is believed that three possible mechanisms might contribute to cold adaptation:
- 1.
Blunted physiological responses to cold stress (habituation)
- 2.
Enhanced thermogenic response (metabolic)
- 3.
Enhanced body heat conservation (insulative)
Despite the belief that the cold-adapted individuals are able to produce more metabolic heat, evidence to suggest this is limited to case studies and weak observational experimental designs [35–39]. Nevertheless shivering is a response to cold stress that increases metabolic heat production, and it is possible that individuals that are adapted to the cold are able to generate more metabolic heat through shivering and non-shivering thermogenesis in comparison to unacclimated persons. Insulative adaptations might occur through enhanced regulation of cutaneous blood flow. Convective heat losses are likely to be minimised as the cold-acclimated individual constricts peripheral blood vessels, reducing blood flow and narrowing the gradient between skin temperature and the environment [40]. Conversely these adaptive physiological responses might be blunted by habituation, particularly metabolic heat production [41, 42]. However, blunted shivering and metabolic heat production limit heat loss and are aided by cutaneous vasoconstriction [40]. In most circumstances, adaptations are environmental and context specific, and behavioural adjustments such as seeking warmth and layering clothing become the primary method of defence against hypothermia. In recent years technological advancements, such as the development of wetsuits for diving in cold water and insulative and wind-protective clothing, have advanced human ability to function and survive cold environments. It should be noted that despite these general concepts, even those matched for anthropometrics, sex, age and acclimatisation and when combined with training factors such as inactivity, fatigue, lack of sleep and endocrine (hypopituitarism, hypoadrenalism, hypothyroidism, hypoglycaemia, diabetes), individual responses to cold stress can vary widely [23].
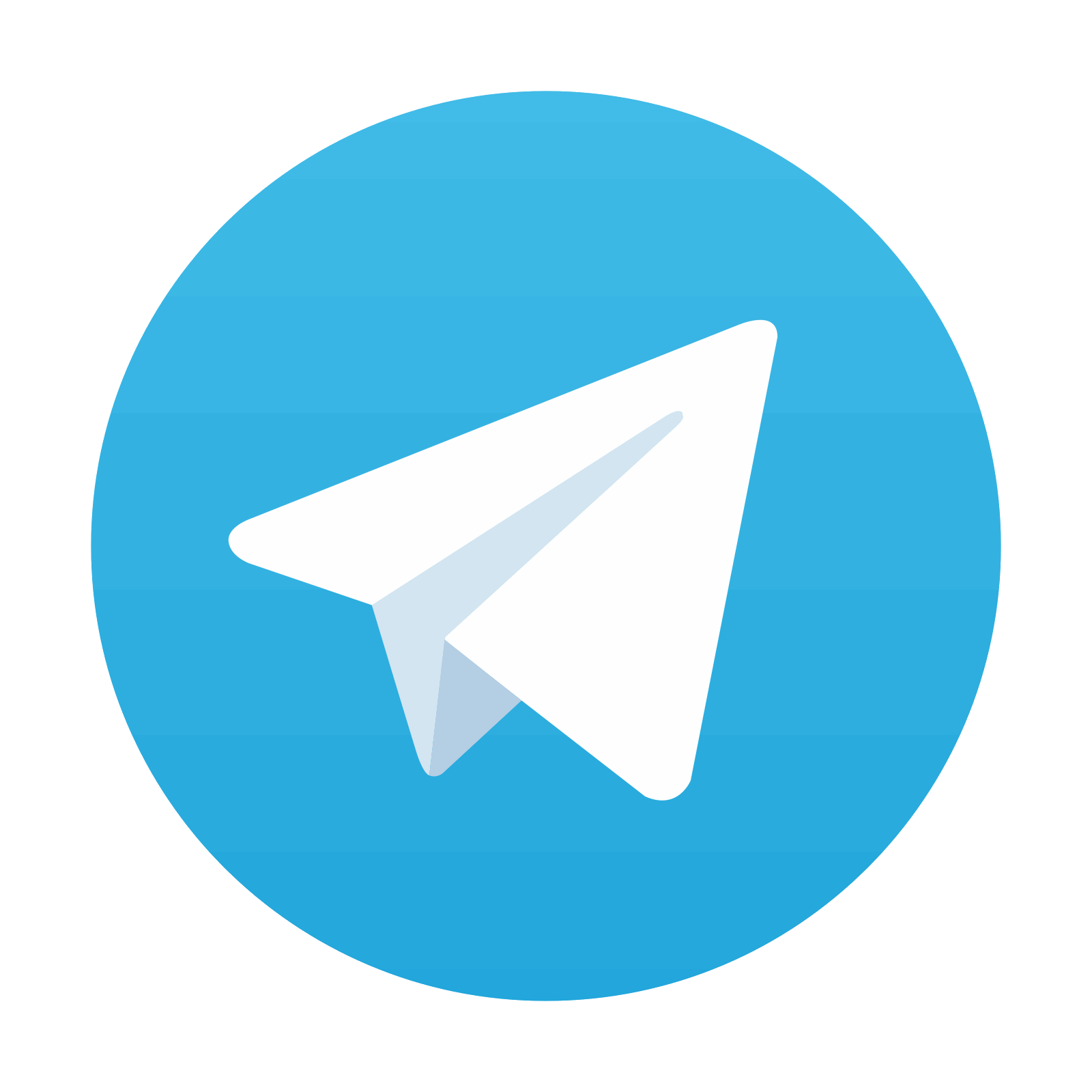
Stay updated, free articles. Join our Telegram channel

Full access? Get Clinical Tree
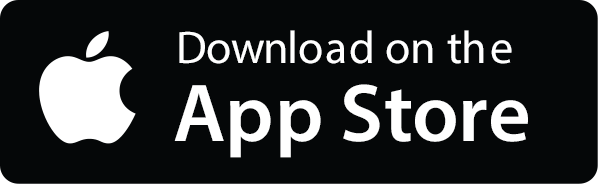
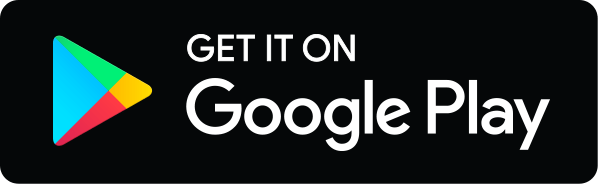