© Springer International Publishing Switzerland 2016
Piero Volpi (ed.)Arthroscopy and Sport Injuries10.1007/978-3-319-14815-1_5757. Use of Scaffolds in Sports Medicine
(1)
II Clinic – Nano-Biotechnology Laboratory, Rizzoli Orthopaedic Institute, Via di Barbiano n. 1/10, Bologna, 40136, Italy
(2)
Biomechanics Laboratory, Rizzoli Orthopaedic Institute, Via di Barbiano n. 1/10, Bologna, 40136, Italy
Keywords
ScaffoldsCartilageSportOsteochondralMultilayerElizaveta Kon is a consultant for CartiHeal (2009) Ltd (Israel) and has stocks in it. She is a consultant and receives institutional support from Fin-Ceramica Faenza S.p.A (Italy). She also receives institutional support from Fidia Farmaceutici S.p.a (Italy), IGEA Clinical Biophysics (Italy), BIOMET (USA), and Kensey Nash (USA).
is a consultant and receives institutional support from Fin-Ceramica Faenza S.p.A. (Italy), Fidia Farmaceutici S.p.a. (Italy), and CartiHeal (2009) Ltd (Israel). He is also a consultant for EON Medica SRL (Italy). He receives institutional support from IGEA Clinical Biophysics (Italy), BIOMET (USA), and Kensey Nash (USA).
has no conflict of interests.
has no conflict of interests.
receives royalties and research institutional support from Fin-Ceramica Faenza S.p.A. (Italy). He receives institutional support from Fidia Farmaceutici S.p.a. (Italy), CartiHeal (2009) Ltd (Israel), IGEA Clinical Biophysics (Italy), BIOMET (USA), and Kensey Nash (USA).
57.1 Introduction
Chondral and osteochondral injuries occur frequently in athletes, thus determining a higher risk of developing premature knee osteoarthritis (OA) with respect to the general population [1, 2]. Flanigan et al. [1] recently showed a mean 36 % prevalence of full-thickness focal chondral defects in athletes; moreover, Walczak et al. [3] found knee cartilage abnormalities in 89 % of asymptomatic professional basketball players using magnetic resonance imaging (MRI). Furthermore, it is well documented that articular cartilage lesions in a sports population commonly arise with concomitant injuries: meniscal (47 %) or anterior cruciate ligament (30 %) tears and medial or lateral collateral ligament tears (14 %) are the most commonly associated conditions [1]. Although the pathogenesis of early OA has not been clarified, it is recognized that traumatic injuries and chronic joint stress due to high-impact sports can lead to an unfavorable biomechanical environment, thus altering the natural homeostasis of articular cartilage [4]. Unfortunately, chondral injuries do not heal spontaneously and, if untreated, might cause a progression of joint degeneration with associated pain and functional limitation, which in turn will impede participating in sport and lead to disability [2, 5–7].
For such reasons, when treating young competitive athletes affected by articular cartilage defects, it is even more important to obtain the most effective and durable joint surface restoration, since the regenerated tissue must be able to withstand the significant joint stresses generated during sports activity [7–9].
Techniques such as microfractures (MF) and mosaicplasty can successfully permit the athlete with articular cartilage injury to return to high-impact sports. MF represents the most frequently applied surgical approach in the clinical practice [10] and consists of arthroscopic bone marrow stimulation aimed at forming a clot of fibrin and precursor cells migrating from the adjacent bone marrow. Although the minimal invasiveness of this technique allows an early return to activity, it has been shown that this procedure offers mainly short-term benefits but tends to fail to provide long-lasting results, probably because of the poor mechanical properties of the newly formed fibrous tissue [11–13]. Mosaicplasty consists of the transplantation of osteochondral autologous cylinders harvested from a low weight-bearing area and implanted in the defect site by a press-fit technique [14]. Unfortunately, despite the positive results documented in the literature and the high percentage of return to sports activity [15], the issue of donor-site morbidity limits the indication of this technique for lesions smaller than 2–3 cm2 [9].
Therefore, novel treatment concepts and techniques have been developed to overcome the limits of the classic approaches. Regenerative procedures are emerging which aim at restoring the articular cartilage defect by producing a high qualitative repair tissue, as similar as possible to the hyaline physiological one, and to allow immediate postoperative rehabilitation, which is directly correlated both with the short-term outcome and longer-lasting results [16–18].
This review briefly illustrates modern surgical strategies for articular cartilage regeneration, particularly focusing on scaffold-based procedures and the evidence for their use in athletic patients.
57.2 Articular Cartilage Scaffolds
Besides the satisfactory results obtained with the first generation of autologous chondrocyte implantation (ACI) in isolated femoral condyle lesions [19], numerous developments have been introduced taking advantage of bioengineering. In fact, first-generation ACI presented several biological and surgical concerns, which led to the development of the so-called “matrix-assisted” autologous chondrocyte transplantation (MACT) [20]. Scaffolds are a temporary three-dimensional structure of biodegradable polymers, which are supposed to promote the growth of living cells and the maintenance of the differentiated chondrocyte phenotype and promote a more homogeneous distribution while avoiding the risk of chondrocyte leakage [21]. Moreover, the solid structure and easy handling of these engineered tissues allow a minimally invasive surgical application and then an early postoperative rehabilitation, which can favor and accelerate functional recovery [16, 22].
The ideal scaffold should fulfill a set of chemical, biochemical, and biophysical requirements able to meet several demands: it should consist of materials with controlled biodegradability or bioresorbability, characterized by adequate interconnecting pores to promote tissue integration and desired mechanical properties to match the site of implantation and handling; moreover, appropriate surface chemistry might promote cell attachment, proliferation, and differentiation. Finally, scaffolds should not induce adverse reactions and also be easily manufactured into a variety of shapes and sizes [23]. All these requirements explain why, despite the concept of “scaffolding” to support cells to infiltrate and regenerate the local tissue was firstly introduced more than one century ago [23], the introduction of scaffolds into clinical practice began only in recent decades [20]. Designing a material able to guide the tissue regeneration process is challenging, but advances in the field of molecular biology and material science have led to the development of numerous biomaterials [23]. Among the several scaffolds proposed in an attempt to better fulfill the requirements for cartilage regeneration, there are substantial differences regarding the materials chosen (natural or synthetic) and their physical forms (fibers, meshes, gels). Natural materials (hyaluronic acid, collagen derivatives, agarose, alginate, fibrin glue, and chitosan) have good biocompatibility, enhance cell proliferation, and are processed in a reliable and reproducible way. Concerning synthetic matrices, they are commonly made of polylactides, including polylactic and polyglycolic, and innovations in the chemistry of these materials have improved their biocharacteristics and biocompatibility.
Unfortunately, concerns about cell-based strategies, such as cost and time consumption because of the ex vivo cell processing [21], oriented research efforts toward different alternative solutions, developed to avoid manipulation of cells and the inherent regulatory obstacles. As an example of these new strategies, some scaffolds may have a potential themselves to promote tissue regeneration by exploiting the self-regenerative potential of the body. Thus, the use of cell-free scaffolds is the most recent trend nowadays. In fact, one-step cell-free approaches avoid problems related to the ex vivo chondrocyte culture and expansion in a scaffold, with marked advantages both from surgical and economic perspectives. The possibility of a cell-free implant, “smart” enough to provide the joint with the appropriate stimuli to induce orderly and durable tissue regeneration, is attractive, and new biomaterials and surgical strategies have been recently proposed to induce in situ cartilage regeneration after direct transplantation onto the defect site [24, 25]. Moreover, increasing awareness about the role of the subchondral bone in the pathogenesis of joint degeneration and early OA progression has led to the development of bilayer products to treat the entire osteochondral unit, thus reproducing the different biological and functional requirements for guiding the growth of both bone and cartilage tissues. In particular, this strategy becomes crucial when treating large chondral or osteochondral articular defects [24, 26].
Among the numerous osteochondral scaffolds developed and tested preclinically, only two have been reported for their clinical application [21]. One is a bilayer porous PLGA-calcium-sulfate biopolymer (TruFit®, Smith & Nephew, Andover, MA) in cylinder form, conceived as an alternative for mosaicplasty plugs [27, 28]. The second one is a three-layered nanostructured biomimetic collagen hydroxyapatite scaffold (MaioRegen®, Fin-Ceramica, Faenza, Italy) composed of the following: (1) a cartilaginous layer, consisting of type I collagen, with a smooth surface; (2) an intermediate layer, a combination of type I collagen (60 % of weight) and Mg-hydroxyapatite (HA) (40 % of weight); and (3) a lower layer that consists of a mineralized blend of type I collagen (30 % of weight) and Mg-HA (70 % of weight). Preclinical studies tested the safety and effectiveness of the implant to promote cartilage and bone tissue formation. A comparative analysis showed similar macroscopic, histological, and radiographic results for the scaffold either loaded with autologous chondrocytes or implanted alone, thus suggesting its ability to induce an in situ regeneration through cells coming from the surrounding bone marrow in the animal model and led to its introduction in the clinical practice as a cell-free approach [29, 30].
57.3 Clinical Evidence in Scaffold Application
MACT techniques were introduced in 1998 to improve the intrinsic limits of the traditional ACI technique. Their application was initially proposed for use in the knee joint, with a considerable number of clinical studies subsequently reported, and these techniques are more recently being applied also for the treatment of articular cartilage lesions in other joints [31]. However, only a few of these studies deal with long-term outcomes [32]. Filardo et al. [33] treated a series of 62 patients (48 M, 14 F, mean age 28.1 ± 11.4 years) with hyaluronan-based MACT (Hyalograft C®, Fidia, Padova, Italy) for chondral lesions (ICRS grade III–IV, mean size 2.5 ± 1.0 cm2) at the femoral condyles and prospectively evaluated them at 7 years’ follow-up. A statistically significant improvement was observed in all the clinical scores from the basal evaluation to 84 months’ follow-up. The IKDC subjective score increased from 39.6 ± 15.0 to 73.6 ± 18.8 at 12 months (p < .005), and a further slight improvement was observed at 24 months’ follow-up (76.5 ± 20.7, ns), then the results were stable up to 77.3 ± 21.5 at final follow-up. Postoperative MRIs were performed in 42 cases, revealing a complete filling of the cartilage defect in 57 %. Further analysis showed that young men with higher pre-injury activity level reached better outcomes, whereas patients with degenerative lesions had worse results. Ibarra et al. documented a different arthroscopic approach based on matrix-encapsulated chondrocytes which obtained a positive clinical outcome in ten patients at 36 months [34]. Positive findings were confirmed by MRI using the MOCART score and T2 mapping quantitative evaluations, and patients were allowed to reach a satisfactory activity and sports level, as shown by the 5.8 mean Tegner score at 24 months, which remained stable at the final follow-up of 3 years [34].
In 2012, Kon et al. performed a comparative study to evaluate whether the regenerative cell-based approach allows highly demanding athletes a better functional recovery compared with the bone marrow stimulation [35]. Forty-one professional or semiprofessional male soccer players were treated and evaluated prospectively at 2 years and at a mean of 7.5 years’ follow-up (minimum 4 years). Twenty-one patients received an arthroscopic MACI (Hyalograft C®), and 20 were treated with MF. Both groups had a significant improvement in all the scores used from preoperative evaluation to final follow-up. The IKDC subjective score showed similar results at 2 years, but the MACI group had a significantly better outcome at the final follow-up. In the MF group, the results decreased over time (from 86.8 ± 9.7 to 79.0 ± 11.6, p < .0005), whereas the Hyalograft C® group presented a more durable outcome, with stable results (90.5 ± 12.8 at 2 years and 91.0 ± 13.9 at final follow-up). Concerning the return to sports activity, 80 % of the patients treated with MF returned back to their previous level, playing the first official soccer game after a median of 8 months; in the Hyalograft C® group, 86 % of patients were back to previous level competitions in a median time of 12.5 months (p = .009). Despite the similar success rate in returning to competitive sport, it can be assumed that MF allows a faster recovery but presents a clinical deterioration over time, whereas arthroscopic MACT might delay the return of high-level male soccer players to competition, but at the same time offering a more durable clinical improvement. Moreover, MACT procedures might offer encouraging outcomes even for the treatment of patellofemoral full-thickness chondral defects, which are considered one of the most challenging lesions to treat. A study on 38 active patients treated with the same hyaluronan-based MACT showed a significant improvement in the clinical scores evaluated 2 and 5 years after surgery and a return to a satisfactory sports activity level, as evaluated by the Tegner score [36, 37].
This approach has even been tested for the treatment of degenerative lesions of the articular surface and gave encouraging clinical results but with a lower improvement and a higher number of failures with respect to patients affected by traumatic injuries [38]. Therefore, in recent years the focus on this kind of articular surface defects, usually linked to an altered joint environment, has extended to the role of the subchondral bone. Biphasic scaffolds were introduced to address both the cartilage and the subchondral bone issues. Currently, the use of just two scaffolds of this kind has been reported in the literature. The first one, a bilayer porous PLGA-calcium-sulfate, produced controversial results and thus is being abandoned in the clinical practice [39, 40].
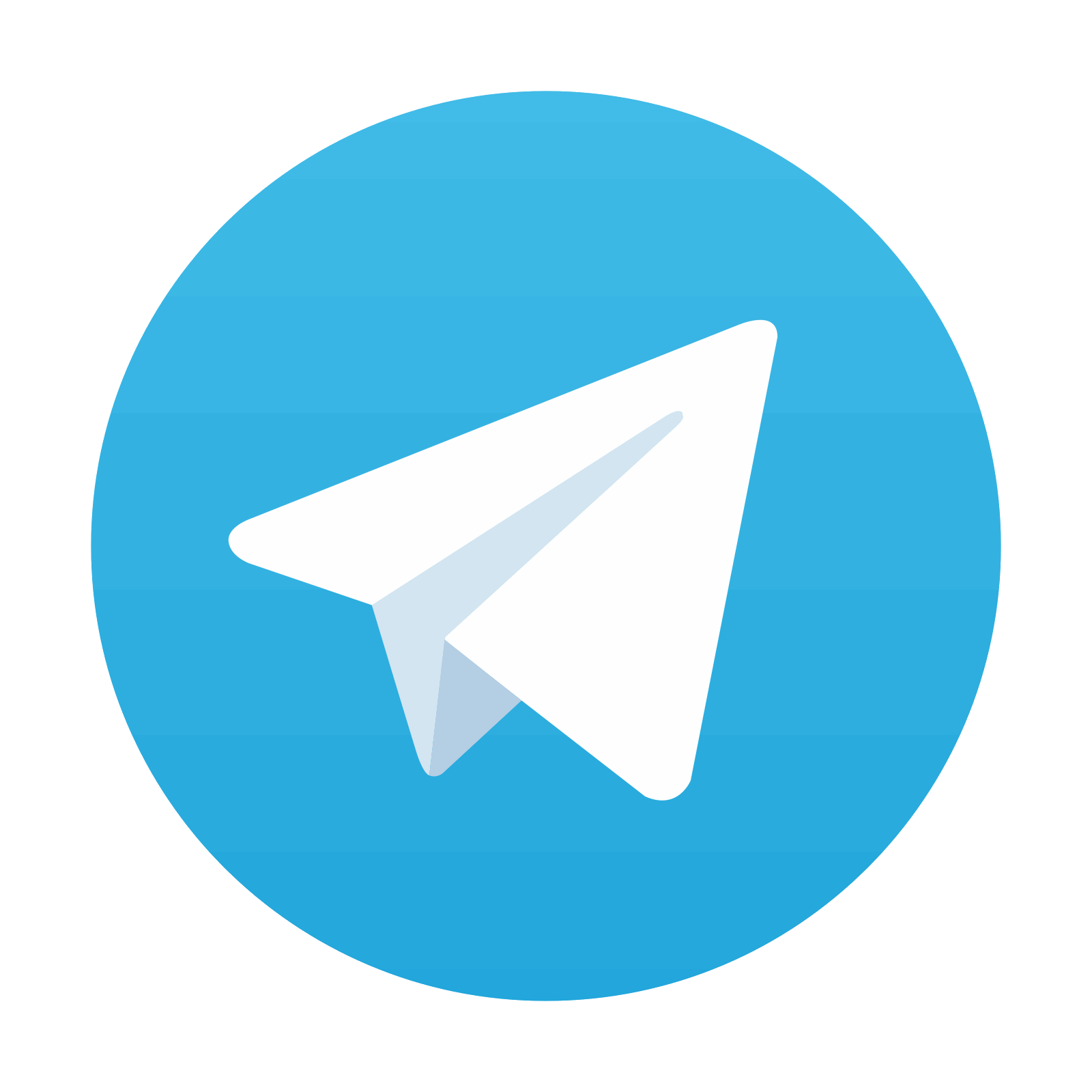
Stay updated, free articles. Join our Telegram channel

Full access? Get Clinical Tree
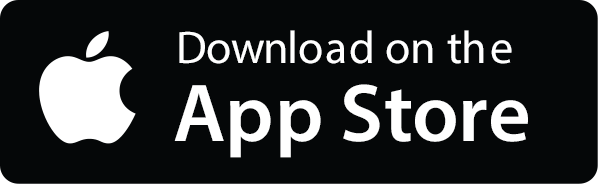
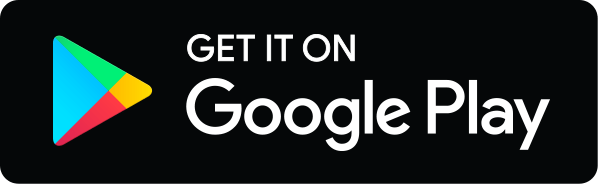