Chapter 14 Upper limb orthoses for the stroke and brain-injured patient
General principles
Stroke and brain injury are often complicated by the development of upper motor neuron syndrome.23,22,39,52 Upper motor neuron syndrome is characterized by impairment of motor control, spasticity, muscle weakness, stereotypical patterns of movement (synergy), and stimulation of distant movement by noxious stimuli (synkinesis). Often the spasticity is severe and prevents adequate range of motion therapy of joints or maintenance of acceptable limb position. Contractures can occur despite the most conscientious and aggressive treatments.
Prevention of deformity and myostatic contractures in the presence of severe spasticity is challenging. Splints applied to only one side of an extremity are not sufficient to control excessive spasticity and may result in skin breakdown from motion of the extremity against the splint.5 If used inappropriately, an orthosis may conceal the severity of a deformity or may cause additional deformity. It is important to treat the underlying spasticity in order to utilize orthoses effectively.
Treatment options for temporary control of spasticity
The majority of spontaneous motor recovery occurs within 6 months of stroke and traumatic brain injury. Definitive surgical procedures to reduce spasticity, such as neurectomies, tendon releases, and transfers, are delayed until the patient shows minimal further improvement in motor control.10,36,48
The prolonged period of spontaneous neurologic recovery is further complicated by spasticity (resistance to quick stretch), rigidity (resistance to slow stretch), impairment of motor control, synergistic patterns of movement, synkinesis (involuntary movement in one limb or limb segment when another part is moved [associated distant movement]), and immobility. These phenomena make temporary control of spasticity difficult but essential. Nerve blocks, chemodenervation, and casting techniques are used commonly and aggressively.6,7,9,13,15,17,22,23,26,51
Phenol nerve blocks
When muscle spasticity requires control for an extended period but the patient still has potential for spontaneous improvement, a phenol nerve block may be indicated.6,7,13,19,27,28,35,37,41,44,45,53,54,69 Phenol exerts two actions on the nerves. The first is a short-term effect. The short-term effect is similar to that produced by a local anesthetic and is directly proportional to the thickness of the nerve fibers. The second is a long-term effect that results from protein denaturation.
Chemodenervation
Use of botulinum toxin also exemplifies a temporary, localized approach to controlling spasticity.15,17,20,22,23,26,29,30,59,61,62,63,66,68 Ordinarily, an action potential propagating down a motor nerve to the neuromuscular junction triggers the release of acetylcholine (ACh) from presynaptic storage sites in the nerve terminal into the synaptic space. The released quanta of ACh, after traversing the synapse and attaching onto receptors located on the postsynaptic muscle membrane, cause its depolarization. This activates a biochemical sequence that ultimately leads to forceful muscle contraction. Botulinum toxin type A is a protein produced by Clostridium botulinum that inhibits the calcium-mediated release of ACh at the neuromuscular junction. Botulinum toxin A attaches to the presynaptic nerve terminal, and a component of the toxin crosses the nerve cell membrane. This component interferes with “fusion proteins” affiliated with vesicles of ACh and thereby prevents release of ACh from their storage vesicles.
Because botulinum toxin is the most potent biologic toxin known and the cost is relatively high, the smallest possible dose should be used to achieve results. Most studies have reported side effects in 20% to 30% of patients per treatment cycle. The incidence of adverse effects varies based on the dosage used (i.e., the higher the dose, the more frequent the adverse effects); however, it has been reported that incidence of complications is not related to the total dose of botulinum toxin used. Local pain at the injection site is the most commonly reported side effect. Other adverse effects (e.g., local hematoma, generalized fatigue, lethargy, dizziness, flulike syndrome, pain in neighboring muscles) also have been reported.
Causes of limited joint motion
The brain-injured patient is likely to have quadriplegic involvement, concomitant peripheral nerve injuries, residual deformities from fractures, and limitation of joint motion from heterotopic ossification.11,47,49 Distinguishing from among several possible causes of decreased range of motion often is difficult in a brain-injured patient. The causes of decreased motion to be considered are increased muscle tone, myostatic contracture, heterotopic ossification, undetected fracture or dislocation, pain, or lack of patient cooperation secondary to decreased cognition.8,10,31 The stroke patient tends to be older. Limited joint motion can be associated with degenerative arthritis. Congenital deformities can be present.
When focal intervention (chemodenervation, neurolysis, or surgery) is being considered, differentiating between the resistance to stretch offered by muscle contraction on a reflex basis versus resistance to stretch generated by inherent physical stiffness properties of muscle tissue is extremely important. EMG examination is another tool that can help make this distinction.21,23,43,48
General classification of orthoses
Uses of orthotic devices
Contracture prevention
A combination of peripheral nerve blocks and casting or splinting techniques are commonly used to give temporary relief of spasticity.10,56–58 Positioning a limb in the desired position for later function is important. Because the clinical situation may change quickly after a traumatic brain injury, a short-term orthosis such as a cast often is a practical choice.5 Casting maintains muscle fiber length and diminishes muscle tone by decreasing sensory input. Lidocaine blocks are helpful when done prior to cast application, because relieving the spasticity allows for easier limb positioning. Casts are used prophylactically to prevent contracture formation in a nonfunctional position. A well-applied circular cast will protect the skin in unconscious patients. Casts are commonly used to treat pressure sores in these patients. Close neurovascular observation is necessary in head injury patients after circular plaster application because many cannot complain of pain secondary to a tight cast.
Orthoses as reinforcement after chemodenervation
When a decision to perform a botulinum toxin injection is made, an orthotic device can be used to maintain the injected muscles in a stretched position and to enhance the effect of the botulinum toxin. In this case, it is better to use progressive or serial orthoses that can be adjusted to increase the amount of stretch.
Contracture correction
Correction of contractual deformities can be obtained by serial cast application done at weekly intervals or use of dropout casts.5 Serial casting is most successful when a contracture has been present for less than 6 months. The patient is sedated, and an anesthetic nerve block is given if necessary to decrease the spasticity. The limb is manipulated for 10 minutes prior to cast application to gain increased joint motion. A well-padded cast is applied, holding the arm in the improved position. Care must be taken not to exert excessive force while applying the cast. The major correction in joint position should have been obtained by the manipulation.
< div class='tao-gold-member'>
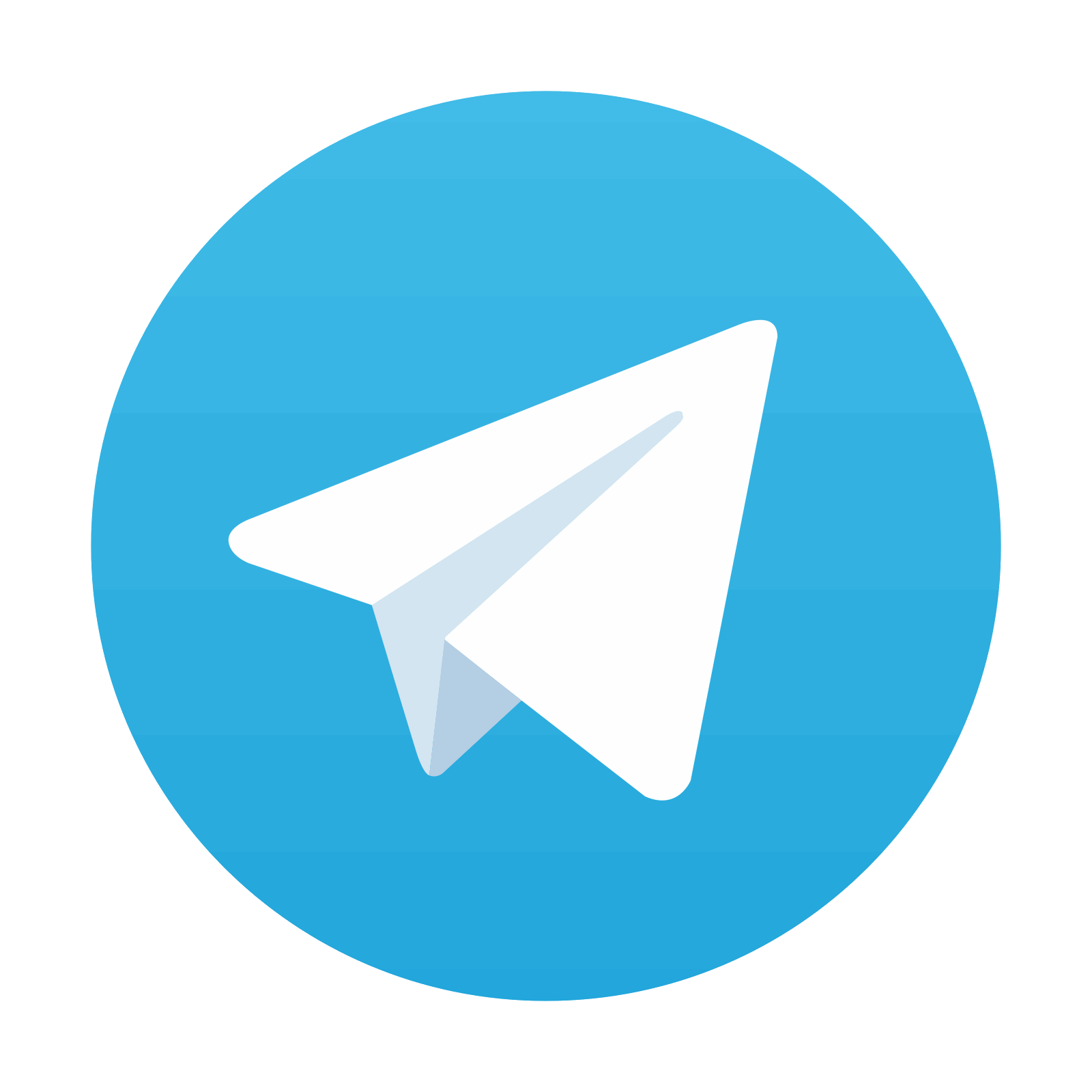
Stay updated, free articles. Join our Telegram channel

Full access? Get Clinical Tree
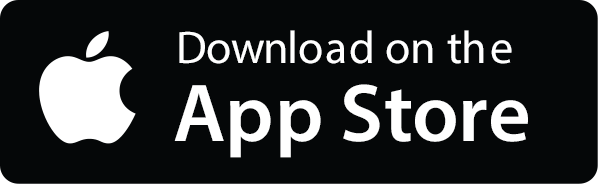
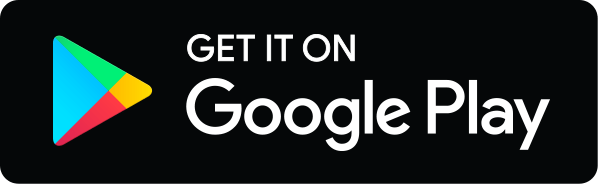