Tissues in the body are designed to function while undergoing the stresses of everyday living. Body weight, friction, and air or water resistance are all types of stresses that commonly act on the body. The ability of the tissues to respond to stress is due to the differing viscoelastic properties of the tissue, with each tissue responding to stress in an individual manner based on design. Maintaining the health of the various tissues is a delicate balance because insufficient, excessive, or repetitive stresses can prove deleterious. Fortunately, most tissues have an inherent ability to self-heal—a process that is an intricate phenomenon. Kinetics is the term applied to define the forces acting on the body. Posture and movement are both governed by the body’s ability to control these forces. The same forces that move and stabilize the body also have the potential to deform and injure the body.1 A wide range of external and internal forces are either generated or resisted by the human body during daily activities. Examples of these external forces include ground reaction force, gravity, and applied force through contact. Examples of internal forces include structural tension, joint compression, and joint shear forces (Fig. 2-1). Under the right circumstances, the body can respond and adapt to these stresses. The terms stress and strain have specific mechanical meanings. Stress, or load, is defined in units of force per area, and is used to describe the type of force applied. Stress is independent of the amount of material, but is directly related to the magnitude of force and inversely related to the unit area.2 Strain is defined as the change in length of a material due to an imposed load, divided by the original length.2 The two basic types of strain are a linear strain, which causes a change in the length of a structure, and shear strain, which causes a change in the angular relationships within a structure. It is the concentration of proteoglycans in solution (see Chapter 1) that is responsible for influencing the mechanical properties of the tissue, including compressive stiffness, sheer stiffness, osmotic pressure, and the regulation of hydration.3 FIGURE 2-1 Internal forces acting on the body.
CHAPTER 2
Tissue Behavior, Injury, Healing, and Treatment
OVERVIEW
THE RESPONSE OF TISSUE TO STRESS
The inherent ability of a tissue to tolerate load can be observed experimentally in graphic form. When any stress is plotted on a graph against the resulting strain for a given material, the shape of the resulting load–deformation curve depends on the kind of material involved. The load–deformation curve, or stress–strain curve, of a structure (Fig. 2-2) depicts the relationship between the amount of force applied to a structure and the structure’s response in terms of deformation or acceleration. The horizontal axis (deformation or strain) represents the ratio of the tissue’s deformed length to its original length. The vertical axis of the graph (load or stress) denotes the internal resistance generated as a tissue resists its deformation, divided by its cross-sectional area. The load–deformation curve can be divided into four regions, each region representing a biomechanical property of the tissue (Fig. 2-2):
FIGURE 2-2 The stress–strain curve.
Toe region. Collagen fibers have a wavy, or folded, appearance at rest or on slack. When a force that lengthens the collagen fibers is initially applied to connective tissue, this slack range is affected first, and the fibers unfold as the slack is taken up (see Crimp later). The toe region is an artifact caused by this take-up of slack, alignment, and/or seating of the test specimen. The length of the toe region depends on the type of material and the waviness of the collagen pattern.
Elastic region. Within the elastic deformation region, the structure imitates a spring—the geometric deformation in the structure increases linearly with increasing load, and after the load is released the structure returns to its original shape. The slope of the elastic region of the load–deformation curve from one point in the curve to another, which corresponds to the physiological range of a structure, is called the modulus of elasticity or Young’s modulus, and represents the extrinsic stiffness or rigidity of the structure—the stiffer the tissue, the steeper the slope. A key characteristic of passive tendon loading is its stiffness—the force in the tendon divided by the amount of lengthening of the tendon.4 Young’s modulus is a numerical description of the relationship between the amount of stress a tissue undergoes and the deformation that results—stress divided by the strain. The ratio of stress to strain in an elastic material is a measure of its stiffness. Young’s modulus is independent of specimen size and is, therefore, a measure of the intrinsic stiffness of the material. The greater the Young’s modulus for a material, the better it can withstand greater forces. Mathematically, the value for stiffness is found by dividing the load by the deformation at any point in the selected range. All normal tissues within the musculoskeletal system exhibit some degree of stiffness. Larger structures will have greater rigidity than smaller structures of similar composition. Stiffness is not necessarily a negative characteristic—tendons transmit force more effectively and efficiently when they are stiffer.4
Plastic region. The end of the elastic deformation range, and the beginning of the plastic deformation range, represents the point where an increasing level of stress on the tissue results in progressive failure and microscopic tearing of the collagen fibers. Further increases in strain result in microscopic damage and permanent deformation. The permanent change results from the breaking of bonds and their subsequent inability to contribute to the recovery of the tissue. Unlike the elastic region, removal of the load in this region will not result in a return of the tissue to its original length.
Failure region. Deformations exceeding the ultimate failure point (Fig. 2-2) produce mechanical failure of the structure, which in the human body may be represented by the fracturing of bone or the rupturing of a soft tissue.
Biological tissues are anisotropic, which means they can demonstrate differing mechanical behavior as a function of test direction. The properties of extensibility and elasticity are common to many biologic tissues. Extensibility is the ability to be stretched, and elasticity is the ability to return to normal length after lengthening or shortening.5
Crimp
The crimp of collagen is one of the major factors behind the viscoelastic properties of connective tissue. Crimp, a collagen tissue’s first line of response to stress, is different for each type of connective tissue, providing each with different viscoelastic properties. Collagen fibers are oriented obliquely when relaxed. However, when a load is applied, the fibers line up in the direction of the applied force as they uncrimp. Crimping is seen primarily in ligaments, tendons, and joint capsules, and occurs in the toe phase of the stress–strain curve (Fig. 2-2).
Viscoelasticity
Viscoelasticity is the time-dependent mechanical property of a material to stretch or compress over time, and to return to its original shape when a force is removed. The mechanical qualities of a tissue can be separated into categories based on whether the tissue acts primarily as a solid, fluid, or a mixture of the two. Solids are described according to their elasticity, strength, hardness, and stiffness. Bone, ligaments, tendons, and skeletal muscle are all examples of elastic solids. Biological tissues that demonstrate attributes of both solids and fluids are viscoelastic. The viscoelastic properties of a structure determine its response to loading. For example, a ligament demonstrates more viscous behavior at lower loads whereas, at higher loads, elastic behaviors dominate.7
Creep and Stress Relaxation
Creep and stress relaxation are two characteristics of viscoelastic materials that are used to document their behavior quantitatively.5
Creep is the gradual rearrangement of collagen fibers, proteoglycans, and water that occurs because of a constantly applied force after the initial lengthening caused by crimp has ceased. Creep is a time-dependent and transient biomechanical phenomenon. Short duration stresses (<15 minutes) do not have sufficient time to produce this displacement; however, longer times can produce it. Once creep occurs, the tissue has difficulty returning to its initial length (see below).
Stress relaxation is a phenomenon in which stress or force in a deformed structure decreases with time while the deformation is held constant.5 Unlike creep, stress relaxation responds with a high initial stress that decreases over time until equilibrium is reached and the stress equals zero, hence the label “relaxation.” As a result, no change in length is produced.
Thus, stress to connective tissues can result in no change, a semipermanent change, or a permanent change to the microstructure of the collagenous tissue. The semipermanent or permanent changes may result in either microfailure.
Plastic Deformation
Plastic deformation of connective tissue occurs when a tissue remains deformed and does not recover its prestress length. Once all of the possible realignment has occurred, any further loading breaks the restraining bonds, resulting in microfailure. On average, collagen fibers can sustain a 3% increase in elongation (strain) before microscopic damage occurs.8 Following a brief stretch, providing the chemical bonds remain intact, the collagen and proteoglycans gradually recover their original alignment. The recovery process occurs at a slower rate and often to a lesser extent. The loss of energy that occurs between the lengthening force and the recovery activity is referred to as hysteresis. The more chemical bonds that are broken with applied stress, the greater the hysteresis. If the stretch is of sufficient force and duration, and a sufficient number of chemical bonds are broken, the tissue is unable to return to its original length until the bonds are re-formed. Instead, it returns to a new length and a new level of strain resistance. Increased tissue excursion is now needed before tension develops in the structure. In essence, this has the effect of decreasing the stabilizing capabilities of the connective tissue.
Stress Response
Exercises may be used to change the physical properties of both muscles/tendons and ligaments, as both have demonstrated adaptability to external loads with an increase in strength:weight ratios.9–11 The improved strength results from an increase in the proteoglycan content and collagen cross-links.9–11
TISSUE INJURY
Soft tissue injuries of all types are extremely common in the general population. Studies have shown that there is a linear relationship between soft tissue injuries and aging, with fewer than 10% of individuals younger than 34 years being affected, in contrast to 32–49% of those older than 75 years being affected.12 Whether a stress proves to be beneficial or detrimental to a tissue is very much dependent on the physiologic capacity of the tissue to accept load. This capacity is dependent on some factors, among them:
The health of the tissue. Healthy tissues can resist changes in their shape. Any tissue weakened by disease or trauma may not be able to resist adequately the application of force.
Age. Increasing age reduces the capacity of the tissues to cope with stress loading.
Proteoglycan and collagen content of the tissue. Both increasing age and exposure to trauma can result in unfavorable alterations in the proteoglycan and collagen content of a tissue.
The ability of the tissue to undergo adaptive change. All musculoskeletal tissue can adapt to change. This capacity to change is determined primarily by the viscoelastic property of the tissue.
The speed at which the adaptive change occurs. This is dependent on the type and severity of the insult to the tissue. Insults of low force and longer duration may provide the tissue an opportunity to adapt. In contrast, insults of a higher force and shorter duration are less likely to provide the tissue time to adapt. The distinction between sudden and repetitive stress is important. An acute stress (loading) occurs when a single force is large enough to cause injury on biological tissues; the causative force is termed macrotrauma. A repetitive stress (loading) occurs when a single force itself is insufficient to cause injury on biological tissues. However, when repeated or chronic stress over a period causes an injury, the injury is called a chronic injury, and the causative mechanism is termed microtrauma. Etiologic factors for microtraumatic injuries are of two basic types: intrinsic or extrinsic. Intrinsic factors are physical characteristics that predispose an individual to microtrauma injuries and include muscle imbalances, leg length discrepancies, and anatomical anomalies.13 Extrinsic factors, which are the most common cause of microtrauma injuries, are related to the external conditions under which the activity is performed. These include training errors, type of terrain, environmental temperature, and incorrect use of equipment.13
INJURY CLASSIFICATION
Injuries to the soft tissues can be classified as primary or secondary.
Primary, or macrotraumatic, injuries can be self-inflicted, caused by another individual or entity, or caused by the environment.14–17 These injuries include fractures, subluxations, and dislocations, which are outside the scope of practice for a physical therapist, and sprains, and strains, which make up the majority of conditions seen in the physical therapy clinic. For the purposes of the intervention, primary injuries are classified into acute, subacute, or chronic.
Acute. This type of injury is usually caused by macrotrauma and indicates the early phase of injury and healing, which typically lasts approximately 4–6 days unless the insult is perpetuated.
Subacute. This phase occurs after the acute phase and typically lasts from 10 to 17 days after the acute phase has ended but may last weeks in those tissues with limited circulation, such as tendons.18
Chronic. This type of injury can have several definitions. On the one hand it may indicate the final stage of healing that occurs 26–34 days after injury but can last 6 months to 1 year depending on the tissue involved and the amount of tissue damage. On occasion, a persistent inflammatory state results in an accumulation of repetitive scar adhesions, degenerative changes, and other harmful effects referred to as subclinical adaptations (see Chapter 8).
Secondary or microtraumatic injuries are essentially the inflammatory response that occurs with the primary injury.19 Microtraumatic injuries include tendinopathy, tenosynovitis, and bursitis.
TISSUE HEALING
Fortunately, the majority of tissue injuries heal without complication in a predictable series of events (Fig. 2-3). The most important factor regulating the regional time line of healing is sufficient blood flow.20 Many factors can determine the outcome of the tissue injury, including those listed in Table 2-1. Also, complications such as infection, compromised circulation, and neuropathy hurt the healing process and can cause great physical and psychological stress to the involved patient and his or her family.
FIGURE 2-3 Stages of healing.
TABLE 2-1 | Factors Impacting Healing |
Intrinsic (Local) | Systemic | Extrinsic |
Extent of injury. Microtears involve only minor damage, whereas macrotears involve significantly greater destruction | Age. The ability to heal injuries decreases with age | Drugs. Nonsteroidal anti-inflammatory drugs and corticosteroids decrease inflammation and swelling, resulting in decreased pain |
Edema. Swelling can cause increased pressure that can impede nutrition to the injured part, inhibit neuromuscular control, and retard the healing process | Obesity. Oxygen pressure in the tissues is lower in obese patients. | Absorbent dressings. The degree of humidity greatly affects the process of epithelialization—the epithelium regenerates twice as quickly in a moist environment |
Hemorrhage. Bleeding produces the same negative effects on healing as does the accumulation of edema | Malnutrition. Wound healing places a higher than usual demand on a patient’s energy resources. In every stage of wound healing, protein is needed. In addition, adequate nutritional intake and body stores of all vitamins are essential. | Temperature and oxygen tension. Hypothermia has a negative effect on healing. Oxygen tension relates to the neovascularization of the wound. |
Poor vascular supply. Wounds heal poorly and at a slower rate when the blood supply is inadequate | Hormone levels. Hormones affect the composition and structure of a variety of tissues. | |
Separation of tissue. A wound that has smooth edges and good apposition will tend to heal by primary intention with minimal scarring. | Infection. Infection can delay healing | Physical modalities. These can be used to promote an efficient healing environment for an injury when used individually, or in combination with other modalities or exercise |
Muscle spasm. Spasm causes traction on the already torn tissue, preventing approximation. | General health. Comorbidity can play a significant role in the overall healing process. For example, diabetes can impede tissue healing | Exercise. Exercise can help in the remodeling process of all connective tissues. Wolff’s law states that tissue remodeling and the response to therapeutic exercise are determined by the specific adaptation of the tissue to the imposed level of demand. |
Atrophy. Consider a secondary impairment to injury and subsequent disuse | ||
Degree of scarring. Scarring that occurs normally, but hypertrophic scarring produces keloids when the rate of collagen production exceeds the rate of collagen breakdown |
Stages of Tissue Healing
The general stages of soft tissue healing are described here, whereas the healing of specific structures is described later under the relevant headings. After microtrauma, macrotrauma, or disease, the body attempts to heal itself through a predictable series of overlapping events that include coagulation and inflammation (acute), which begins shortly after the initial injury; a migratory and proliferative process (subacute), which begins within days and includes the major processes of healing; and a remodeling process (chronic), which may last for up to a year depending on the tissue type, and is responsible for scar tissue formation and the development of new tissue.14,20–24
Whereas simplification of the complex events of healing into separate categories may facilitate understanding of the phenomenon, in reality these events occur as an amalgamation of different reactions, both spatially and temporally.25
The various therapeutic approaches that can be used during each of these stages of healing are described in Chapter 8.
Coagulation and Inflammatory Stage
An injury to the soft tissue triggers a process that represents the body’s immediate reaction to trauma, which includes a series of repair and defensive events.20,26 Following an injury to the tissues, the cellular and plasma components of blood and lymph enter the wound. Capillary blood flow is disrupted, causing hypoxia to the area. This initial period of vasoconstriction, which lasts 5–10 minutes, prompts a period of vasodilation, and the extravasation of blood constituents.20 Extravasated blood contains platelets, which secrete substances that form a clot to prevent bleeding and infection, clean dead tissue, and nourish white cells. These substances include macrophages and fibroblasts.27 The coagulation and platelet release results in the excretion of platelet-derived growth factor (PDGF),28 platelet factor 4,29 transforming growth factor-alpha (TGF-α),30 and transforming growth factor-beta (TGF-β).31 The main functions of a cell-rich tissue exudate are to provide cells capable of producing the components and biological mediators necessary for the directed reconstruction of damaged tissue, while diluting microbial toxins and removing contaminants present in the wound.25
Inflammation is mediated by chemotactic substances, including anaphylatoxins that attract neutrophils and monocytes.
Neutrophils. Neutrophils are white blood cells (WBCs) of the polymorphonuclear (PMN) leukocyte subgroup (the others being eosinophils, and basophils) that are filled with granules of toxic chemicals (phagocytes) that enable them to bind to microorganisms, internalize them, and kill them.
Monocytes. Monocytes are WBCs of the mononuclear leukocyte subgroup (the other being lymphocytes). The monocytes migrate into tissues and develop into macrophages, providing immunological defenses against many infectious organisms. Macrophages serve to orchestrate a “long-term” response to injured cells subsequent to the acute response.32
The WBCs of the inflammatory stage serve to clean the wound debris of foreign substances, increase vascular permeability, and promote fibroblast activity.32 Other cell participants include local immune accessory cells, such as endothelial cells, mast cells, and tissue fibroblasts. The PMN leukocytes, through their characteristic “respiratory burst” activity, produce superoxide anion radical, which is well known to be critical for defense against bacteria and other pathogens.33 Superoxide is rapidly converted to a membrane permeable form, hydrogen peroxide (H2O2), by superoxide dismutase activity or even spontaneously.32 The release of H2O2 may promote the formation of other oxidants that are more stable (have a longer half-life), including hypochlorous acid, chloramines, and aldehydes.32 The phagocytic cells that initiate the innate immune response produce a set of proinflammatory cytokines (e.g., TNF-α, IL-1, and IL-6) in the form of a cascade that amplifies the local inflammatory response, influences the adaptive immune response, and serves to signal the central nervous system (CNS) of an inflammatory response (Fig. 2-3). The extent and severity of this inflammatory response depend on the size and the type of the injury, the tissue involved, and the vascularity of that tissue.17,23,34–36
Local vasodilation is promoted by biologically active products of the complement and kinin cascades25:
The complement cascade involves 20 or more proteins that circulate throughout the blood in an inactive form.25 After tissue injury, activation of the complement cascade produces a variety of proteins with activities essential to healing.
The kinin cascade handles the transformation of the inactive enzyme kallikrein, which is present in both blood and tissue, to its active form, bradykinin. Bradykinin also contributes to the production of tissue exudate through the promotion of vasodilation and increased vessel-wall permeability.37
Because of the variety of vascular and other physiological responses occurring, this stage of healing is characterized by swelling, redness, heat, and impairment or loss of function. The edema is due to an increase in the permeability of the venules, plasma proteins, and leukocytes, which leak into the site of injury, resulting in edema.38,39 New stroma, often called granulation tissue, begins to invade the wound space approximately 4 days after the injury.38,39 The complete removal of the wound debris marks the end of the inflammatory process.
Clinically, this stage is characterized by pain at rest or with active motion, or when specific stress is applied to the injured structure. The pain, if severe enough, can result in muscle guarding and a loss of function. This is often referred to as the protection phase based on the focus of the intervention. The goals of the intervention during this phase are therefore to minimize pain and edema, control inflammation, restore full, passive range of motion, prevent atrophy, maintain soft tissue joint integrity, and to enhance function (see Chapter 8).
Two key types of inflammation are recognized: the normal acute inflammatory response and an abnormal, chronic, or persistent inflammatory response. Common causes for a persistent chronic inflammatory response include infectious agents, persistent viruses, hypertrophic scarring, poor blood supply, edema, repetitive mechanical trauma, excessive tension at the wound site, and hypersensitivity reactions.40,41 The monocyte-predominant infiltration, angiogenesis, and fibrous changes are the most characteristic morphologic features of chronic inflammation. This perpetuation of inflammation involves the binding of neutrophilic myeloperoxidase to the macrophage mannose receptor.42
Migratory and Proliferative Stage
The second stage of soft tissue healing, characterized by migration and proliferation, usually occurs from the time of the initial injury and overlaps the inflammation phase. Characteristic changes include capillary growth and granulation tissue formation, fibroblast proliferation with collagen synthesis, and increased macrophage and mast cell activities. This stage handles the development of wound tensile strength.
After the wound base is free of necrotic tissue, the body begins to work to repair and close the wound (Fig. 2-3). The connective tissue in healing wounds is composed primarily of collagen, types I and III43 cells, vessels, and a matrix that contains glycoproteins and proteoglycans. Proliferation of collagen results from the actions of the fibroblasts that have been attracted to the area and stimulated to multiply by growth factors, such as PDGF, TGF-β, fibroblast growth factor (FGF), epidermal growth factor, and insulin-like growth factor-1, and tissue factors such as fibronectin.25 This proliferation produces first fibrinogen and then fibrin, which eventually becomes organized into a honeycomb matrix and walls off the injured site.44
The wound matrix functions as glue to hold the wound edges together, giving it some mechanical protection while also preventing the spread of infection. However, the wound matrix has a low tensile strength and is vulnerable to breakdown until the provisional extracellular matrix (ECM) is replaced with a collagenous matrix. The collagenous matrix facilitates angiogenesis by providing time and protection to new and friable vessels. Angiogenesis occurs in response to the hypoxic state created by tissue damage as well as to factors released from cells during injury.25
The process of neovascularization during this phase provides a granular appearance to the wound as a result of the formation of loops of capillaries and migration of macrophages, fibroblasts, and endothelial cells into the wound matrix. Once an abundant collagen matrix has been deposited in the wound, the fibroblasts stop producing collagen, and the fibroblast-rich granulation tissue is replaced by a relatively acellular scar, marking the end of this stage.
Upon progressing to this stage, the active effusion and local erythema of the inflammation stage are no longer present clinically. However, residual effusion may still be present at this time and resist resorption.45,46 From a clinical perspective, this phase is often referred to as the controlled motion phase. The treatment goals for this phase are to protect the forming collagen, direct its orientation to be parallel to the lines of force it must withstand, and prevent cross-linking and scar contracture (see Chapter 8).
Remodeling Stage
An optimal wound environment lessens the duration of the inflammatory and proliferative phases and protects fragile tissue from breakdown during early remodeling. The remodeling phase involves a conversion of the initial healing tissue to scar tissue. This lengthy phase of contraction, tissue remodeling, and increasing tensile strength in the wound can last for up to 1 year. Fibroblasts handle the synthesis, deposition, and remodeling of the ECM. Following the deposition of granulation tissue, some fibroblasts are transformed into myofibroblasts, which congregate at the wound margins and start pulling the edges inward, reducing the size of the wound. Increases in collagen types I and III and other aspects of the remodeling process are responsible for wound contraction and visible scar formation. Epithelial cells migrate from the wound edges and continue to migrate until similar cells from the opposite side are met. This contracted tissue, or scar tissue, is functionally inferior to original tissue and is a barrier to diffused oxygen and nutrients.47 Eventually, the new epidermis becomes toughened by the production of the protein keratin. The visible scar changes color from red or purple that blanches with slight pressure, to nonblanchable white as the scar matures.
Imbalances in collagen synthesis and degradation during this phase of healing may result in hypertrophic scarring or keloid formation with superficial wounds. If the healing tissues are kept immobile, the fibrous repair is weak, and there are no forces influencing the collagen—if left untreated, the scar formed is less than 20% of its original size.48 Contraction of the scar results from cross-linking of the collagen fibers and bundles, and adhesions between the immature collagen and surrounding tissues, producing hypomobility. In areas where the skin is loose and mobile, this creates the minimal effect. However, in areas such as the dorsum of the hand where there is no extra skin, wound contracture can have a significant effect on function. Consequently, controlled stresses must always be applied to new scar tissue to help prevent it from shortening.20,36 Scarring that occurs parallel to the line of force of a structure is less vulnerable to reinjury than a scar that is perpendicular to those lines of force.49
Normally, the remodeling phase is characterized by a progression to pain-free function and activity. For this reason, this healing phase is often referred to as the return to function phase (see Chapter 8). The therapeutic goals for this phase are outlined in Chapter 8. Clinically, the chronic inflammatory response is characterized by the signs and symptoms of acute inflammation (redness, heat, edema, and pain), but at a much less pronounced level. In the ideal world, the injured patient makes a smooth transition through the various stages of healing, and the sharp and burning acute pain is replaced by a duller ache, which then subsides to a point where no pain is felt. However, a persistent chronic inflammatory response results in the continued release of inflammatory products and a local proliferation of mononuclear cells. The macrophages remain in the inflamed tissue if the acute inflammation does not resolve, and begin to attract large numbers of fibroblasts, which invade and produce increased quantities of collagen.13 This failure during the healing phase continuum can result in chronic pathologic changes in the tissue. Often, the increased collagen production results in decreased extensibility of a joint or soft tissue structure. Characteristics of this chronic inflammation include a physiologic response that is resistant to both physical and pharmacologic intervention, resulting in a failure to remodel adequately, an imperfect repair, and a persistence of symptoms.40,51 Also, fibrosis can occur in synovial structures, and in extraarticular tissues, including tendons and ligaments; in bursa; or in muscle.
MUSCLE BEHAVIOR, INJURY, HEALING, AND TREATMENT
As outlined in Chapter 1, skeletal muscles primarily function to transmit forces mechanically via their respective tendons to provide movement.
Behavior
There are approximately 430 skeletal muscles in the body, each of which can be considered anatomically as a separate organ. Of these 430 muscles, about 75 pairs provide the majority of body movements and postures.52 Like any collagenous tissue, the musculotendinous unit exhibits viscoelastic properties (stress relaxation, creep, hysteresis, etc.) allowing it to respond to load and deformation appropriately, with the rate of deformation being directly proportional to the applied force when considering the viscous property. Three important factors can influence muscle performance:53
Age. With aging, the cross-sectional area of muscle decreases decline and the number of muscle fibers are reduced by about 39% by age 80.54 Type I muscle fibers are not affected much by aging, but type II fibers demonstrate a reduction in cross-sectional area of 26% from age 20 to 80, most likely a result of denervation.55 These changes seem to be secondary to the declining demand of the muscle and lack of physical activity, and thus can be minimized or even reversed with adequate training (see “Muscle and Aging” section).54
Temperature. Collagenous tissues have an inverse temperature-elastic modulus relationship, especially at higher temperatures, which means that temperature elevation results in increased elasticity and decreased stiffness.56 This would suggest that warming a muscle may confer a protective effect against muscle strain injury as warmer muscles must undergo greater deformation before failure. However, somewhat contradictory, a warm muscle may be prone to injury because it undergoes greater deformation to attain a given load.
Immobilization or disuse. The effect of rigid immobilization on muscle has been well detailed (see “Detrimental Effects of Immobilization” section later). However the results of restricted motion, in which joint and associated muscles are not allowed to move through the complete range, have not been well studied. Biomechanically, muscle immobilized in a shortened position develops less force and stretches to a shorter length before becoming susceptible to injury than does a nonimmobilized muscle. A muscle immobilized in a lengthened position responds differently; greater force and a greater change in length are required to cause a tear than in nonimmobilized muscle.
Injury
Muscle injury can result from excessive strain, excessive tension, contusions, lacerations, thermal stress, and myotoxic agents, such as some local anesthetics, excessive use of corticosteroids, and snake and bee venoms.57 The majority of muscle injuries (>90%) are caused either by excessive strain of the muscle or by contusion.58 Muscle strains may be graded by severity (Table 2-2). Muscle injuries are the most common injury in sports, with an incidence varying from 10% to 55% of all injuries sustained in sports events.59,60 A number of factors contribute to muscle strain injury, including:53
TABLE 2-2 | Classification of Muscle Injury |
Type | Related Factors |
Exercise-induced muscle injury (delayed muscle soreness) | Increased activity Unaccustomed activity Excessive eccentric work Viral infections Muscle cell damage |
Strains First degree (mild): minimal structural damage; minimal hemorrhage; early resolution Second degree (moderate): partial tear; large spectrum of injury; significant early functional loss Third degree (severe): complete tear; may require aspiration; may require surgery |
Onset at 24–48 hours after exercise Sudden overstretch Sudden contraction Decelerating limb Insufficient warm-up Lack of flexibility Increasing severity of strain associated with greater muscle fiber death, more hemorrhage, and more eventual scarring Steroid use or abuse Previous muscle injury Collagen disease |
Contusions Mild, moderate, severe Intramuscular vs. intermuscular | Direct blow, associated with increasing muscle trauma and tearing of fiber proportionate to severity |
Data from Reid DC. Sports Injury Assessment and Rehabilitation. New York, NY: Churchill Livingstone; 1992. |
inadequate flexibility
inadequate strength or endurance
dyssynergistic muscle contraction
insufficient warm-up
inadequate rehabilitation from the previous injury
A distraction strain occurs in muscle to which an excessive pulling force is applied, resulting in overstretching.58 A contusion may occur if a muscle is injured by a heavy compressive force, such as a direct blow. At the site of the direct blow, a hematoma may develop. Two types of hematoma can be identified:61
- Intramuscular. This type of hematoma is associated with a muscle strain or bruise. The size of the hematoma is limited by the muscle fascia. Clinical findings may include pain and loss of function.
- Intermuscular. This type of hematoma develops if the muscle fascia is ruptured, and the extravasated blood spreads into the interfascial and interstitial spaces. The pain is usually less severe with this type.
Healing
Skeletal muscle has considerable regenerative capabilities, and the process of skeletal muscle regeneration after injury is a well-studied cascade of events.62–64 The essential process of muscle regeneration is similar, irrespective of the cause of injury, but the outcome and time course of regeneration vary according to the type, severity, and extent of the injury.62,65,66 Broadly speaking, there are three phases in the healing process of an injured muscle: the destruction phase, the repair phase, and the remodeling phase.61
Destruction Phase
The pathology of skeletal muscle damage varies, depending on the initiating cause. Muscle damage can occur during the prolonged immobility of hospitalization and from external sources such as mechanical injury.67 One of the potential consequences of muscle injury is atrophy. The amount of muscle atrophy that occurs depends on the usage prior to bed rest and the function of the muscle.67 Antigravity muscles (such as the quadriceps) tend to have greater potential for atrophy than antagonist muscles (such as the hamstrings). Research has shown that a single bout of exercise protects against muscle damage, with the effects lasting between 6 weeks68 and 9 months.69
Muscle resistance to damage may result from an eccentric exercise-induced morphologic change in the number of sarcomeres connected in series.70 This finding appears to support initiating a reconditioning program with gradual progression from lower intensity activities with minimal eccentric actions to protect against muscle damage.67,71 If a muscle is severely injured, the muscle fibers and their connective tissue sheaths are totally disrupted, and a gap appears between the ends of the ruptured muscle fibers after the muscle fibers retract.64 This phase is characterized by the necrosis of muscle tissue, degeneration, and an infiltration by PMN leukocytes as hematoma and edema form at the site of injury.
Repair Phase
The repair phase usually involves the following steps:
Hematoma formation. The gap between the ruptured ends of the fibers is at first filled by a hematoma. During the first day, the hematoma is invaded by inflammatory cells, including phagocytes, which begin disposal of the blood clot.64
Matrix formation. Blood-derived fibronectin and fibrin cross-link to form a primary matrix, which acts as a scaffold and anchorage site for the invading fibroblasts.63,64 The matrix gives the initial strength for wound tissue to withstand the forces applied to it.72 Fibroblasts begin to synthesize proteins of the ECM.
Collagen formation. The production of type I collagen by fibroblasts increases the tensile strength of the injured muscle. An excessive proliferation of fibroblasts can rapidly lead to an excessive formation of dense scar tissue, which creates a mechanical barrier that restricts or considerably delays complete regeneration of the muscle fibers across the gap.61,64
During the first week of healing, the injury site is the weakest point of the muscle–tendon unit. This phase also includes regeneration of the striated muscle, production of a connective-tissue scar, and capillary ingrowth. The regeneration of the myofibers begins with the activation of satellite cells, located between the basal lamina and the plasma membrane of each myofiber.73
Satellite cells, myoblastic precursor cells, proliferate to reconstitute the injured area.66 During muscle regeneration, it is presumed that trophic substances released by the injured muscle activate the satellite cells.74 Unlike the multinucleated myofibers, these mononuclear cells maintain mitotic potential and respond to cellular signals by entering the cell cycle to provide the substrate for muscle regeneration and growth.73
The satellite cells proliferate and differentiate into multinucleated myotubes and eventually into myofibers, which mature and increase in length and diameter to span the muscle injury. Many of these myoblasts can fuse with existing necrosed myofibers and may prevent the muscle fibers from completely degenerating.73
The final stage in the regenerative process involves the integration of the neural elements and the formation of a functional neuromuscular junction.75,76 Provided that the continuity of the muscle fiber is not disrupted, and the innervation, vascular supply, and ECM are left intact, muscle will regenerate without loss of normal tissue architecture and function.77
Remodeling Phase
In this phase, the regenerated muscle matures and contracts with the reorganization of the scar tissue. There is often complete restoration of the functional capacity of the injured muscle. The tensile strength of the healing muscle tissue increases over time. However, whereas normal intramuscular collagenous tissue has a greater proportion of type I collagen than type III collagen, initially after injury, type III collagen demonstrates a significant increase over type I collagen in the area of repair. Over time, the proportion of type I to type III collagen returns to normal. Controlled mobility and stress are key considerations in the post-acute period to allow scar formation, muscle regeneration, correct orientation of new muscle fibers, and the normalization of the tensile properties of muscle.
Treatment
The intervention depends on the stage of healing (see Chapter 8). The following principles should guide the clinician when rehabilitating a muscle injury:53
Prevention is easier than treatment. Patient education is important to inform the patient about the expected duration and extent of symptoms, and any precautions or contraindications to prevent reinjury or disruption of the healing process.
Controlled mobility and activity are best.
Medications and modalities can be important adjuncts to care.
It is important to develop strong, flexible tissue using pain as the guiding factor.
The typical exercise progression involves PROM, then AAROM, then AROM, and then submaximal isometrics, initially in a protective range before progressing throughout the range. Once the patient can tolerate submaximal isometrics, a progression to maximal isometrics is made at multi-angles and then throughout the range, before progressing to progressive resistive exercises.
Muscle and Aging
With age, there is a reduction in the ability to produce and sustain the muscular power. This age-related phenomenon, termed senescence sarcopenia, can result in a 20–25% loss of skeletal muscle mass (see Chapter 30).78
Sarcopenia has important consequences. The loss of lean body mass reduces function, and loss of approximately 40% of lean body mass is fatal.79,80 Sarcopenia is distinct from wasting—involuntary weight loss resulting from inadequate intake, which is seen in starvation, advanced cancer, or acquired immunodeficiency syndrome.
While a variety of studies have investigated the underlying mechanisms and treatments of age-related muscle loss, very few epidemiologic studies have looked at the prevalence, incidence, pathogenesis, and consequences of sarcopenia in elderly populations. It is likely that the determinants of sarcopenia are multifactorial and include genetic factors, environmental factors, and age-related changes in muscle tissue.81
The effects of aging on muscle morphology have been studied. Aging causes a decrease in muscle volume,82 with type II fiber apparently being more affected by gradual atrophy.83 Specifically, there is a disproportionate atrophy of type IIa muscle fibers with aging. These losses of muscular strength and muscle mass can have important health consequences because they can predispose the elderly to disability, an increased risk of falls and hip fractures, and a decrease in bone mineral density.
TENDON BEHAVIOR, INJURY, HEALING, AND TREATMENT
As outlined in Chapter 1, tendons primarily function to transmit force between muscle and bone.84
Behavior
The organization of the tendon determines its mechanical behavior. As tendons have more parallel collagen fibers than ligaments, and less realignment occurs during initial loading, the toe region of the load–deformation curve is smaller in tendons than in ligaments.85 The compliance of tendons varies. Tendons of the digital flexors and extensors are very stiff, and their length changes very little when muscle forces are applied through them. In contrast, the tendons of some muscles, particularly those involved in locomotion and ballistic performance, are more elastic. For example, the Achilles tendon is stretched during the late stance phase in gait as the triceps surae is stretched as the ankle dorsiflexes. Near the beginning of the plantar flexion contraction, the muscle activation ceases and energy stored in the stretched tendon helps to initiate plantar flexion. The resting tendon has a slightly crimped or wave-like appearance. As force is applied to the tendon, a straightening of the crimp results in the toe region in the load–deformation curve (Fig. 2-2), where little force is required to change tendon length.84 Total tendon strains (percentage deformity) of 1–2% result in the straightening of the crimp pattern of unloaded tendon collagen. Strains of 2–6% are well tolerated by most healthy tendons. However, with a strain higher than 6%, incomplete tears start to occur within the tendon, and complete structural failure typically occurs in the range of 8–10%.86 As the load increases beyond the toe region, the collagen fibrils stretch, creating the linear region of the load–deformation curve (Fig. 2-2).84 Most tendons likely function in the toe and early linear regions under physiological loading conditions.87
Tendon tissue homeostasis is based on the ability of the tendon cells to sense and respond to mechanical load through mechanotransduction.88 As with all CT, tendons have a positive adaptive response to repeated physiologic mechanical loading, which results in biologic and mechanical changes. A tendon can resist tensile stress in the directional of its fibers orientation because of the collagen structure, and it can resist some compressive stress because of its proteoglycan content. The total amount of load the tendon can resist and the amount it stretches during loading depend on its cross-sectional area, composition, and length. However, tendons can adapt structurally or materially to changes in the mechanical environment as a result of load conditions.87 The exact level of mechanical and biological stimulation required to maintain normal tendon homeostasis is not currently known, but is widely believed that an abnormal level of stimulation (under load or overload) may play a role in the pathogenesis of tendinopathy.88 Strain injuries are common at the musculotendinous junction (MTJ), the weakest point in the muscle tendon unit.89
Injury
Tendinopathy is a clinical syndrome, often but not always implying overuse of a tendon, characterized by a combination of pain, diffuse or localized swelling, and impaired performance.93 Recently, it has been recommended that the term tendinopathy replace the traditional term tendinitis for describing tendon pathology.94 Tendinitis, which is seen to a much lesser extent (<3%), is associated with the classical signs of inflammation usually observed during the early reparative phase.95 However, confusing the issue is the fact that the noninflammatory etiology of tendinopathy has lately been questioned, as inflammation may play a role in the initial phase of the disease.96 This may be because the tendon insertion and bursa surrounding the tendon are common sites of classical inflammation as a response to repetitive stress, because of their greater density of blood vessels and nerves,97 whereas the tendon proper is mostly aneural and avascular and does not, under normal conditions, exhibit classical inflammatory responses.98,99 Tendon overuse injuries have been reported to account for 30–50% of injuries in sports and 30% of all general practitioner consultations for musculoskeletal injuries.100 Tendons that transmit large loads under eccentric and elastic conditions, such as the Achilles and patellar tendons, are more subject to injury.101 Thus, in the lower extremity, chronic tendon overuse accounts for 30% of all running-related injuries, typically involving the patellar or Achilles tendons.102 In the upper extremities, the supraspinatus, and extensor carpi radialis brevis tendons appear to be the most vulnerable.
The etiology of tendinopathy is still unclear; however, histological evidence consistently demonstrates an absence of prostaglandin-mediated inflammation.103 The common pathological conditions associated with tendinopathy are tendinosis and peritendinitis97:
Tendinosis. Tendinosis is an intratendinous degenerative lesion without an inflammatory component (the suffix “-osis” is indicative of a degenerative process rather than an inflammatory disorder).104 This condition is characterized by the histopathological findings of collagen disorganization and fiber separation, increase in mucoid ground substance, hypercellularity, and nerve and vessel ingrowth but mostly without signs of intratendinous inflammation (tendinitis).99
Peritendinitis. This condition is an acute or chronic inflammation of the thin membrane, paratenon, surrounding the tendon, often induced by repetitive exercise and characterized by local swelling and infiltration of inflammatory cells.95
Specimens taken from torn tendons show disorientation of collagen fibers, thinning of the fibers, myxoid degeneration, chondroid metaplasia, calcification, and vascular infiltration.105
Although sports activity is the most common source of tendinopathy, it can be work related, drug related (e.g., cortisone, cyclosporine, statins, and quinolone antibiotics), or due to a metabolic disorder such as disturbed glucose metabolism and atherosclerosis.97 Most tendon trauma tends to occur from loading (sudden overload or repetitive), or rapid unloading. This mechanical loading is anabolic by upregulating collagen gene expression and increasing synthesis of collagen proteins, which peaks around 24 hours after exercise and remains elevated for up to 70–80 hours.97 Simultaneously, there is a degradation of collagen proteins, although the timing of this catabolic peak occurs earlier than the anabolic peak, resulting in a net loss of collagen around the first 24–36 hours after training, followed by a net gain in collagen.99 This would tend to indicate that a certain restitution time interval in between exercise bouts is critical for the tissue to adapt and to avoid a net catabolic situation.
Mechanical overload does not seem to be the only factor to explain a tendon injury and may even be merely a permissive factor, allowing the tendon problem to become symptomatic.106
While it is easy to understand why a sudden overload could damage a tendon, it is more difficult to understand why the application of normal loads, repeated frequently, can cause tendon damage. Repetitive loading can occur as a result of external factors, such as anatomic predisposition resulting from inflexibility, weakness, or malposition, excessive compression, and friction.107–109
Muscle physiology experiments have shown the force increases as the velocity of active muscle lengthening (eccentric) increases while the opposite is true during concentric (shortening) muscle activations (see Chapter 1). Indeed, the tendon is exposed to larger loads during eccentric loading, especially if the movement occurs rapidly.111,112 Almost all concentric contractions are preceded by a lengthening (eccentric) while the muscle is active. This activation pattern stretches the elastic elements in the muscle–tendon unit and contributes to movement if the muscle is allowed to shorten immediately after being lengthened.87
Terminology regarding tendon pathology has been somewhat confusing. Tendon injuries are typically classified as either acute or chronic.
Acute injuries. Acute injuries include those in which the time and method of injury are known.87 Acute injuries include tendon rupture and partial tendon tears. Tendinopathy can be graded according to severity:
Grade I: Pain only after activity; does not interfere with performance; often generalized tenderness; disappears before the next exercise session.
Grade II: Minimal pain with activity; does not interfere with intensity or distance; usually localized tenderness.
Grade III: Pain interferes with activity; usually disappears between sessions; definite local tenderness.
Grade IV: Pain does not disappear between activity sessions; seriously interferes with the intensity of training; significant local signs of pain, tenderness, crepitus, and swelling.
Grade V: Pain interferes with sport and activities of daily living; symptoms often chronic or recurrent; signs of tissue changes and altered associated muscle function.
Chronic injuries. Chronic tendon injuries are not typically associated with a known onset or with inflammation but involve repetitive loading that damages the tendon.
A chronic tendon injury is characterized by the presence of dense populations of fibroblasts, vascular hyperplasia, and disorganized collagen.115 The disorganized collagen is termed angiofibroblastic hyperplasia.116 It is thought that this disorganization results from a failure of the ECM to adapt to changes, resulting in disordered healing with the absence of inflammatory cells. Degenerative tendinopathy occurs in approximately one-third of the population older than 35 years of age.117 Although it is commonly presumed that pain results from an inflamed structure, it is not clear why tendinosis is painful, given the absence of acute inflammatory cells, nor is it known why the collagen fails to mature. Necropsy studies have shown that these degenerative changes also may be present in asymptomatic tendons.118 The degree of degeneration increases with age and may represent part of the normal aging process.90 The degeneration appears to be activity related, as well (see Chapter 30).90
The typical clinical finding for tendinopathy is a strong but painful response to the resistance of the involved musculotendinous structure.
Healing
There are some factors that handle the poor healing potential of tendinous tissue, and these include:
The oxygen consumption by tendons (and ligaments) is 7.5 times lower than skeletal muscle, resulting in a lower metabolic rate.119
The blood vessels do not extend beyond the proximal third of the tendon.
Healing can create adhesion formation and even though remodeling occurs, the biochemical and mechanical properties are never the same as normal tendon tissue.
If an acute tendon injury disrupts vascular tissues within the tendon, it results in a well-studied healing process involving three overlapping phases120:
Inflammatory response: This occurs as a hematoma forms within erythrocytes and activated platelets. This is followed by the infiltration of inflammatory cells, including neutrophils, monocytes, and macrophages that migrate to the injury site to remove debris. Shortly after, chemotactic signals induce fibroblasts to start synthesizing collagen.104 Following injury, the initial inflammatory stage triggers an increase in glycosaminoglycans (GAG) synthesis within days, rapidly followed by synthesis of types I and III collagen, such that the healing wound can be subjected to low levels of force within a matter of days.87,121–124
Repair: This phase involves the deposition of collagen and tendon matrix components.
Remodeling: During this phase the collagen becomes more structured and organized, although the injured site never achieves the original histologic or mechanical features of a healthy uninjured tendon.
Unlike most soft tissue healing, healing tendons pose a particular problem as they require both extensibility and flexibility at the site of attachment.19,122–124 A judicious application of force is necessary to encourage collagen synthesis and collagen fiber cross-linking, which in turn facilitates the new collagen fibrils to align in the direction of force application (see Chapter 8).87
Treatment
While the treatment for tendinopathy appears relatively straightforward, tendinopathies are difficult to grade in terms of guiding treatment or prognosis. Since inflammatory infiltrates are largely absent in tendinopathy, it is recognized that anti-inflammatory strategies are ineffective for this condition. This shift in understanding of pathophysiology has prompted the use of interventions such as eccentric exercises to be considered as a viable option for rehabilitation.125 For example, the eccentric heel drop exercise is commonly used in clinical practice for Achilles tendinopathy although the mechanical properties are not clear. Table 2-3 outlines some guidelines for treatment.
TABLE 2-3 | Eccentric-Based Treatment for Chronic Tendinopathy |
Principles and Method | Description |
1. Warm-up | A generalized exercise such as cycling or like jogging that does not cause local pain or discomfort local heating modality, such as hot pack ultrasound |
2. Stretching to improve flexibility | A minimum of two 30-second static stretches of the involved musculotendinous unit and its antagonist(s) |
3. Completion of the specific eccentric exercise | Three sets of 10 repetitions, with a brief rest or stretch between each set. Symptoms should be felt after 20 repetitions (i.e., between 20 and 30 repetitions) at a level similar to that felt during activities. -If pain is felt before the 20th repetition, either the speed of movement is reduced, or the load is decreased -If no pain is experienced by 30 repetitions, the speed or load is increased. The intensity of subsequent treatments is based on the patient’s response to the previous treatment |
4. Repeat flexibility exercises | See step 2 |
5. Apply ice | Ice is applied for 10–15 minutes to the painful to palpation area |
Data from Curwin SL. Tendon pathology and injuries: Pathophysiology, healing, and treatment considerations. In: Magee D, Zachazewski JE, Quillen WS, eds. Scientific Foundations and Principles of Practice in Musculoskeletal Rehabilitation. St. Louis, MO: WB Saunders; 2007:47–78. |
Some biomechanical factors are important to consider during the treatment of tendinopathies, which include:4
Tendon mechanical properties in healthy individuals may respond to exercise by increasing stiffness if a specific threshold is exceeded.
Tendon mechanical properties are affected by a variety of common conditions (e.g., bed rest, tendinopathy, and rupture), usually by decreasing stiffness.
Exercises that targets tendon stiffness likely will need to hit a specific threshold, and possibly frequency, to be effective.
Treatment of tendinosis involves a multifaceted approach, which includes:87
The identification and removal of all negative internal and/or external forces/factors.
The establishment of a stable baseline for treatment.
The determination of the tensile load starting point
A progression of the loading program according to the patient’s symptoms. Eccentric training produces around 20% more load on the tendon compared with concentric training.126 Studies have shown that exercise, particularly eccentric-based exercise (Table 2-3), has positive effects on the mechanical and structural properties of tendons:
Nakamura et al.127 demonstrated that eccentric exercises contributed to stable angiogenesis in early tendon injury whereas concentric exercises did not. Daily eccentric exercises were clinically beneficial and not harmful to tendon microcirculation.
Knobloch et al.128 showed in a controlled study that in tendinosis lesions, an eccentric loading program resulted in a significant decrease in the paratenon vascularity and pain while not changing the oxygen saturation of the paratenon tissues.
Shalabi et al.129 demonstrated decreased tendon volume, decreased magnetic resonance imaging (MRI) signal in the tendinosis lesions, and improved clinical pain scores in patients with Achilles tendinosis who were treated with eccentric training regimens.
Dimitrios et al.130 reported that eccentric training and static stretching exercises combined were superior to eccentric training alone to reduce pain and improve function in patients with patellar tendinopathy.
Conversely, immobilization adversely affects the biomechanical properties of a tendon, resulting in decreased tensile strength, increased stiffness, and a reduction in total weight.85
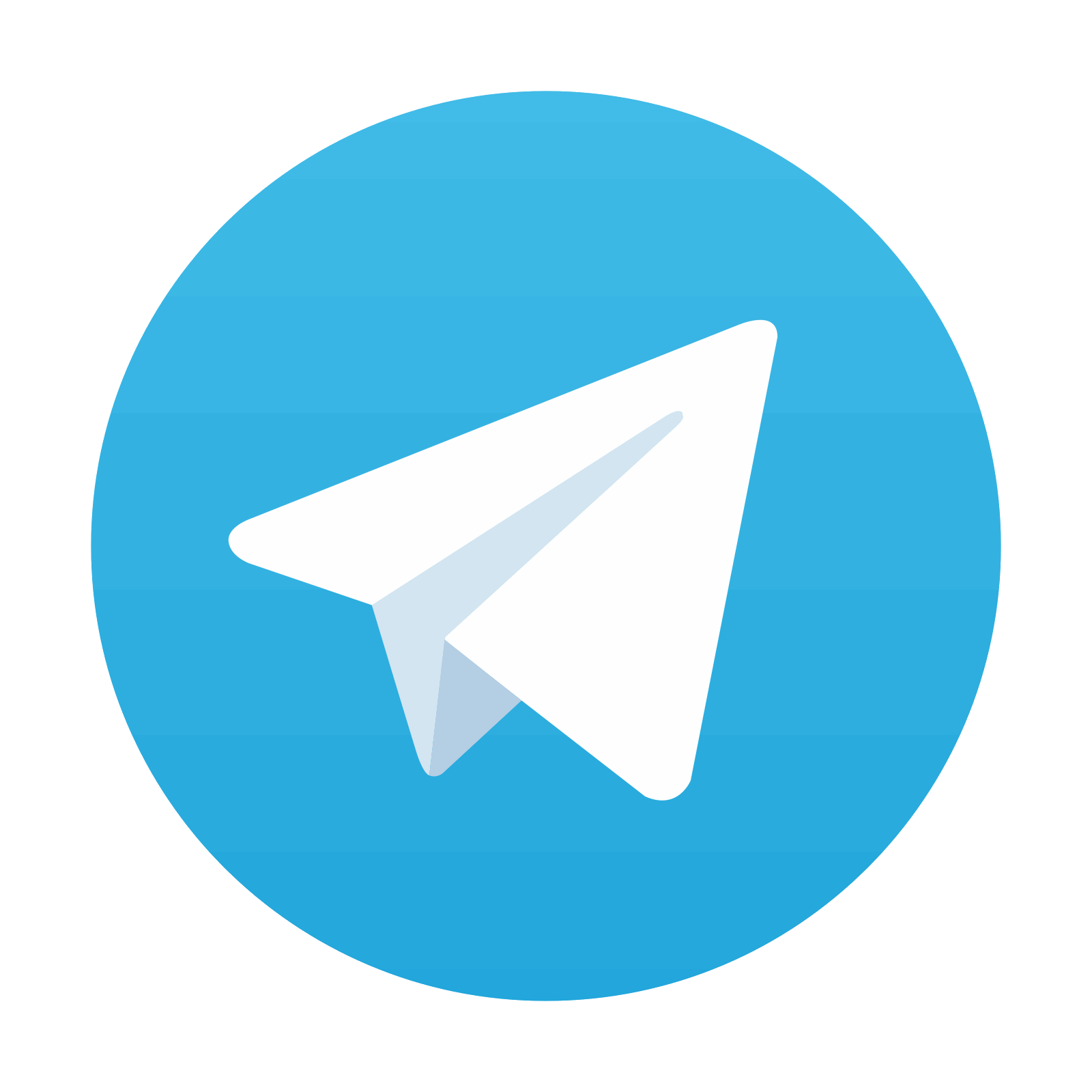
Stay updated, free articles. Join our Telegram channel

Full access? Get Clinical Tree
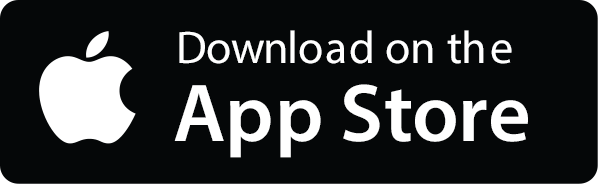
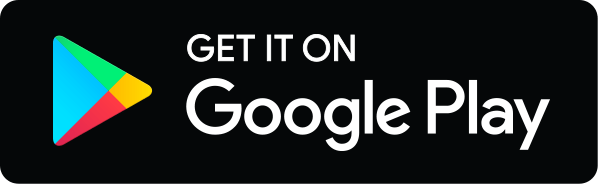
