Thoracolumbar Spine and Lower Extremity Fractures
Ernest L. Sink
John M. Flynn
FRACTURES OF THE THORACIC AND LUMBAR SPINE
Clinical Features.
Spine fractures in children represent 1% to 2% of all pediatric fractures (1). Most of these injuries involve the cervical spine and are discussed in Chapter 21. Causes of spine injury include falls, athletic activities, and battering, but trauma due to motor vehicle accidents is most common (2, 3 and 4). Thoracolumbar spine injuries are more common in older children and adolescents but less common than in adults (5). The true incidence is difficult to determine and the reported incidence may be too low because some children with trauma severe enough to cause spinal fracture may die from associated injuries (6). Approximately two-thirds of thoracolumbar spine fractures in adults are in the region of T12-L2, but the distribution of pediatric and adolescent spine fractures is more uniform throughout the thoracic and lumbar spine (7).
There is a 50% incidence of associated injuries in children who sustain spine trauma from motor vehicle crashes (3). Complete examination is essential when evaluating a child with multiple injuries because spine fractures are occasionally overlooked (8, 9). Physical examination has a high sensitivity when diagnosing spine fractures (5). Examination may reveal tenderness, swelling, ecchymosis, or a palpable defect posteriorly along the spinous processes. A seat belt mark across the abdomen or injury of an abdominal organ should increase the index of suspicion. Any loss of sensory or motor function should be accurately documented.
Spinal cord injury is less frequent in children than in adults. Perhaps this is because the pediatric spine is much more flexible than the adult spine, allowing greater deformation without fracture. This increased musculoskeletal elasticity is not shared by the spinal cord and may lead to a clinical entity known as spinal cord injury without radiographic abnormality (SCIWORA) (10). The disproportionately large head size and other structural features in children place the cervical and upper thoracic regions at greatest risk for spinal cord injury. Trauma to the lower thoracic or lumbar spine in children is rarely associated with spinal cord injury. The prognosis for recovery from incomplete neurologic injury is better in children than in adults, but complete lesions rarely improve (3).
Plain radiographs should be obtained when spine trauma is suspected, but these may be difficult to interpret. Multilevel injuries are common so imaging of the entire spine is recommended (2). A computed tomography (CT) scan or an MRI or both are indicated for evaluation of most patients when thoracolumbar injuries are suspected or known to be present (9). A CT scan is especially helpful to evaluate the bony structures. Sagittal and coronal reconstruction can be used to evaluate alignment and spinal canal encroachment. MRI is more useful than CT scan to evaluate the spinal cord, intervertebral discs, and other soft-tissue structures (9). An MRI is indicated in all cases with neurologic deficit.
Anatomy and Classification.
The thoracic and lum bar vertebrae develop form three main ossification centers, one each for the left and right sides of the neural arch and one for the body. The junction of the arches with the body occurs at the neurocentral synchondrosis. This junction is visible radiographically until the age of 3 to 6 years. It lies just anterior to the base of the pedicle and can be misinterpreted as a congenital anomaly or a fracture in younger children. Secondary centers of ossification occur in flattened, disc-shaped epiphyses superior and inferior to each vertebral body. These centers provide longitudinal growth but do not cover the entire vertebral
body (11). Ossification of these growth plates at the age of 7 to 8 years creates the radiographic impression of a groove at the corner of each vertebral body. This groove is circumferential around the upper and lower end plates of each vertebra. The ligaments and discs attach to this groove, which is therefore an apophyseal ring. The ring apophysis develops its own ossification center by the age of 12 to 15 years and fuses with the remainder of the vertebra at skeletal maturity (12).
body (11). Ossification of these growth plates at the age of 7 to 8 years creates the radiographic impression of a groove at the corner of each vertebral body. This groove is circumferential around the upper and lower end plates of each vertebra. The ligaments and discs attach to this groove, which is therefore an apophyseal ring. The ring apophysis develops its own ossification center by the age of 12 to 15 years and fuses with the remainder of the vertebra at skeletal maturity (12).
Classification systems for thoracolumbar spine fractures in children have not been proposed. The three-column theory of Denis (13) allows classification of adult fractures and also has relevance for the pediatric population. According to this theory, the thoracolumbar spine consists of anterior, middle, and posterior columns. The anterior column includes the anterior longitudinal ligament, the anterior half of the vertebral body, and the anterior portion of the annular ligament. Middle column structures are the posterior half of the vertebral body, the posterior annulus, and the posterior longitudinal ligament. The posterior column includes the neural arch, the ligamentum flavum, the facet joint capsules, and the interspinous ligament. Spinal stability is primarily dependent on the status of the middle column (14).
Denis (13) applied this three-column theory to classify minor or major thoracolumbar fractures. Minor injuries include isolated fractures of the posterior elements. Major fractures are subdivided into compression fractures, burst fractures, seatbelt-type injuries, and fracture dislocations. Compression of the anterior column is usually stable and results from axial loading in flexion. Lateral compression fractures of the vertebral body may also occur. Further compression results in a burst fracture that is unstable because the middle column becomes involved. Lap-belt injuries (Chance fractures) are unstable because they disrupt the posterior and middle columns by flexion and distraction forces. Fracture dislocations usually involve all three columns and result from various combinations of forces.
Certain types of thoracolumbar injuries are unique to children; these include most cases of SCIWORA, posterior limbus or apophyseal fractures, and fractures associated with child abuse.
Treatment of Thoracolumbar Fractures.
Older adolescents sustain injuries similar to those seen in adults and should be managed accordingly (15). Most thoracolumbar spine fractures in children and younger adolescents are minor, stable, and without neurologic deficit. Symptomatic treatment and gradual resumption of activities are generally sufficient for management of these injuries. In the active athlete with an acute fracture of the pars intra-articularis, a thoracolumbarsacral orthosis is recommended for 6 to 8 weeks in an attempt to obtain union.
Compression Fractures.
Most compression fractures in children occur in the thoracic spine. Underlying causes of bone fragility, such as leukemia, should be considered when trauma has been minimal. Multiple compression injuries are not uncommon. Remodeling with restoration of anterior vertebral height has been observed in children younger than 13 years (4, 7). When wedging of a thoracic or lumbar vertebra is <10 degrees, symptomatic treatment is recommended until the patient is comfortable, followed by gradual resumption of activities. When wedging is >10 degrees and the Risser sign is <3, immobilization in hyperextension is recommended for a period of 2 to 3 months (4). Surgical stabilization is recommended when compression is >15 degrees, or approximately 50% compression of the anterior vertebra, compared to posterior vertebral height. Surgical stabilization is also recommended when lateral compression is >15 degrees (6, 15). The authors follow these guidelines, although prolonged bracing after initial treatment is usually avoided.
Burst Fractures.
Treatment guidelines for burst fractures are similar to those for compression fractures in children and adolescents. These injuries may be managed nonoperatively when the posterior column is intact, deformity is minimal, and there is no neurologic injury (4, 16, 17 and 18). However, progressive kyphosis has been noted in some patients treated nonoperatively (18). Nonsurgical treatment usually consists of hyperextension casting for 2 to 3 months and bracing for an additional 6 to 12 months. Surgical decompression and instrumentation are recommended for patients with greater degrees of deformity or with neurologic compromise (16, 17). Posterior distraction and instrumentation may achieve decompression by ligamentotaxis with reduction of the retropulsed fragments (19). Anterior decompression has been recommended in the presence of multiple nerve root paralysis, but the role of anterior decompression and instrumentation remains controversial (20).
Lap-belt Fractures (Chance-type Fracture).
This flexion distraction injury has been associated with the use of lapbelt restraints, when the lap belt slides up the torso and rests over the abdomen instead of the proximal thighs and hips (20). The incidence of Chance fractures in children has increased since the introduction of mandatory seat-belt laws. Fortunately, this injury has a better prognosis in children than in adults (21). Neurologic deficits are infrequent, but intra-abdominal injury is common and obscures the diagnosis of spine trauma.
A flexion distraction injury is unstable in most patients. Treatment consists of cast immobilization for 8 to 10 weeks when there is minimal displacement and the fracture line goes through bone. Posterior surgical stabilization is indicated in the presence of displacement, neurologic deficits, or when there is a significant ligamentous disruption. Instrumentation and fusion one level above and one level below the fracture may be required. In some patients one-level posterior fusion is sufficient (8, 20), typically with pedicle screw fixation in older children (Fig. 34-1).
Limbus Fracture (Apophyseal Fracture).
This fracture is typically seen in the adolescent or young adult and presents clinically like a herniated nucleus pulposus. It often results from the patient’s lifting a heavy object, but may result from falls or twisting injuries. The patient may describe a “pop” at the time of injury, followed by radiculopathy. Delayed diagnosis is common (22). Takata et al. (23) described four types of growth-plate injuries to the spine. Nonoperative management is rarely successful regardless of the type (17, 22). MRI, CT scan, or both should be used to determine the exact location and configuration of the lesion. Surgical excision is then performed by piecemeal excision of the limbus fragment. In order to completely remove bony impingement, the authors recommend laminectomy with direct exposure rather than relying on minimally invasive techniques.
Complications.
Complications of thoracolumbar fractures in children are uncommon unless there is an accompanying neurologic deficit. Inadequate stabilization or late deformity may be problematic when there is an associated neurologic deficit, or after wide laminectomy (24, 25). In the absence of spinal cord injury, remodeling is more likely in younger children, particularly when the iliac apophysis is incompletely ossified (Risser sign <3) (4, 7). However, remodeling can occur in older children. Spontaneous remodeling and redevelopment of the spinal canal has been observed in adults after burst fractures with canal encroachment (26). End-plate injury has been correlated on MRI with disc degeneration, but back pain is uncommon after spine fractures in children (27).
PELVIC FRACTURES
Clinical Features.
Fractures of the pelvis are less common in children than in adults and represent only 0.2% of all pediatric fractures (28). The immature pelvis is more malleable than that of an adult, largely because of the lower modulus of elasticity of pediatric bones, and the greater flexibility of adjacent joints. More energy is required to cause a fracture in the immature pelvis. The greater energy absorption also means that associated injuries are common and the greatest cause of morbidity. Motor vehicle-related accidents are the most common cause of pediatric pelvic fractures (29, 30 and 31). Most unstable pelvic fractures are caused when a motor vehicle strikes a pedestrian (31, 32). Pediatric pelvic fractures include avulsion fractures (usually sports injuries, covered in Chapter 31, stable and unstable pelvic ring fractures, and acetabular and triradiate cartilage injuries. Most pediatric pelvic fractures are stable and minimally displaced.
Approximately 20% of polytraumatized children have pelvic fractures (33), and approximately 58% to 87% of children with pelvic fractures have associated injuries (31, 32). These associated injuries include head injuries, intra-abdominal trauma, urologic disruptions, and fractures. There is little to no difference in mortality rates or injury severity as measured by the ISS between adults and children (34). Death occurs in 3% to 5% of children with juvenile pelvic trauma (28, 31, 32). Death is most frequently related to head injury and mortality related to exsanguination due to the pelvic fracture alone is rare (34). Yet, exsanguination from fractures or visceral injuries can occur, and the risks of hemorrhage and associated visceral injuries correlate with fracture patterns. Patients with bilateral anterior and posterior fractures are at greatest risk, whereas isolated pubic ramus fractures have the lowest risk of hemorrhage and intra-abdominal injury (35, 36).
Evaluation includes a careful physical examination for associated injuries, including neurologic deficits. Any laceration should be inspected to determine whether an open fracture has occurred. Rectal examination is indicated to look for hemorrhage signifying bone penetration into the rectum and to verify intact perineal sensation (i.e., sacral plexus function). Pelvic stability should be tested with anterior and lateral compression of the pelvis. Peripheral arterial circulation should also be noted. A single anteroposterior plain radiograph can provide key information about the pelvic ring and is useful for initial screening. If there are indications of a more unstable injury, these other views should be obtained once the patient is stable: pelvic inlet (40 degrees caudal), outlet (40 degrees cephalad), and Judet (45 degrees oblique) views. These views can help define the fracture pattern and the potential involvement of the acetabulum and triradiate cartilage injury. These views have largely been replaced with CT scan, with or without three-dimensional reconstruction. CT scan, often routinely obtained looking for visceral injury, can diagnose minor fractures in the pelvic ring and assist with classification and decision making when operative intervention is indicated.
Anatomy and Classifications of Pelvic Fractures.
The pelvis is formed from three ossification centers: the ischium, the pubis, and the ilium. These come together at the acetabulum to form the triradiate cartilage. Secondary ossification centers can be confused with fractures. These appear at the apophyses in patients between 13 and 16 years of age. The apophyses that are principally associated with avulsion injuries are located on the ischial tuberosity, the anterior inferior iliac spine, and the anterior iliac crest. Secondary centers of ossification can also develop along the pubis and the ischial spine. Several other normal variants can also be confused with fractures. An area of particular confusion is at the junction of the inferior pubic ramus and the ischium. Before ossification, this junction can have the appearance of a fracture, especially when ossification is asymmetric. A swelling may also occur in this area and can simply be observed when asymptomatic.
Several classifications have been proposed for pelvic fractures (37); however, the key features for decision making include (i) whether the pelvis is mature or immature (38) and (ii) if the fracture is stable or unstable. Mature patients with a closed triradiate are managed according to adult treatment classification and guidelines. Plain radiographs can allow reliable determination of fracture types, although CT scanning may be
helpful in questionable cases or when surgical intervention is anticipated (39). The authors prefer the classification proposed by Watts (40):
helpful in questionable cases or when surgical intervention is anticipated (39). The authors prefer the classification proposed by Watts (40):
Avulsions
Fractures of the pelvic ring (stable and unstable)
Fractures of the acetabulum
Pelvic fracture stability can be subclassified using the AO/ASIF classification of adult pelvic fractures (30). This classification is based on both the mode of injury and the resulting characteristics of the fracture.
Type A: Stable Injury.
Stable injuries include fractures of the pubic ramus (Fig. 34-2) or iliac wing. The ramus fractures may be isolated or may involve both the superior and the inferior rami. It should be noted that, because of the elasticity of the child’s pelvis, diastasis of the pubic symphysis can occur in children without instability of the sacroiliac joint posteriorly. In young children, this fracture usually represents separation at the bone-cartilage junction rather than joint disruption. This “single ring” fracture that is stable without anterior symphysis or ramus injury may also occur near the sacroiliac joint.
Type B: Rotationally Unstable Fractures.
This is a pelvic ring disruption that is stable in the vertical plane but unstable in the transverse plane. Mechanisms include lateral compression causing, for instance, pubic and ischial ramus fractures with contralateral sacral fracture. Alternatively, anterior compression may cause an “open-book” type of injury with pubic diastasis.
Type C: Rotationally and Vertically Unstable Fractures.
This group includes bilateral pubic rami fractures (straddle injuries), which rarely displace in children; vertical shear fractures through the ipsilateral anterior and posterior pelvic rings; and anterior ring fractures with acetabular disruption.
Treatment of Pelvic Fractures
Pelvic Ring Fractures.
Regardless of how the fracture is classified using the many different classification schemes, the assessment and treatment of pediatric pelvic fractures is dependent on two factors: whether the fracture is stable or unstable. A stable fracture is a single ring injury or multiple fractures with displacement of <1 cm. An unstable injury has an anterior and posterior ring fracture with displacement >1 cm.
Most pelvic fractures in children are stable injuries and have a favorable result with symptomatic treatment and weight-bearing restrictions with close follow-up. Unstable pelvic fractures with minimal displacement (<1 cm), which do not involve the acetabulum, can be managed with weight-bearing restrictions and close radiographic follow-up. There has been a trend toward a more aggressive operative approach in displaced unstable fractures. Historically, a nonoperative approach to unstable fractures has been acceptable due to the belief that the pelvis would remodel and there was minimal long-term morbidity. Recent literature suggests that residual deformity can lead to long-term morbidity of leg-length discrepancy, back pain, pelvic asymmetry, and sacroiliac arthritis (41).
The authors recommend fracture reduction of unstable fractures that are displaced >1 cm. The challenges include determining instability and reduction and stabilization in the pediatric pelvis. Anterior stabilization can be performed with external fixation or symphysis plating (Fig. 34-3). Unstable fractures may require internal fixation of posterior injury, in addition to anterior stabilization with external fixation or other means. Posterior stabilization can be achieved with a combination of
plates and screws through a posterior or an iliac fossa approach. Sacroiliac screws can be used in the immature pelvis as long as the S1 body is large enough. Careful fluoroscopy, surgeon expertise, and CT scanning can facilitate SI screw fixation (Fig. 34-3) (30, 37, 42). Traction and spica casting is an option in the patient where size may limit internal fixation. A multispecialty approach is recommended to improve outcomes. In the urgent situation, all the specialties should work together to stabilize the patient. Temporary pelvic wrapping or anterior external fixation can help the hemodynamic status and assist with patient mobilization.
plates and screws through a posterior or an iliac fossa approach. Sacroiliac screws can be used in the immature pelvis as long as the S1 body is large enough. Careful fluoroscopy, surgeon expertise, and CT scanning can facilitate SI screw fixation (Fig. 34-3) (30, 37, 42). Traction and spica casting is an option in the patient where size may limit internal fixation. A multispecialty approach is recommended to improve outcomes. In the urgent situation, all the specialties should work together to stabilize the patient. Temporary pelvic wrapping or anterior external fixation can help the hemodynamic status and assist with patient mobilization.
Acetabular Fractures.
Watts (40) classified acetabular fractures into four types. This classification has been used to guide
treatment (43). Precise restoration of joint congruity is recommended except for stable posterior fracture dislocations with concentric reductions (Watts type I fractures). Nondisplaced acetabular fractures are managed by closed reduction (Watts type II fractures). Multiple fragments with instability (Watts type III) are best managed by open reduction with stable internal fixation (30, 43). Early motion is also recommended (30). Comminution is often less severe in children than in adults, and the results of surgical management are generally satisfactory. Fractures with central dislocation of the hip have a poor prognosis regardless of surgical or nonsurgical management. Traction may be utilized for these fractures, or surgical management may be attempted when comminution is minimal. Poor results after anatomic reduction may still occur with all types of acetabular fractures due to the magnitude of the initial trauma (44).
treatment (43). Precise restoration of joint congruity is recommended except for stable posterior fracture dislocations with concentric reductions (Watts type I fractures). Nondisplaced acetabular fractures are managed by closed reduction (Watts type II fractures). Multiple fragments with instability (Watts type III) are best managed by open reduction with stable internal fixation (30, 43). Early motion is also recommended (30). Comminution is often less severe in children than in adults, and the results of surgical management are generally satisfactory. Fractures with central dislocation of the hip have a poor prognosis regardless of surgical or nonsurgical management. Traction may be utilized for these fractures, or surgical management may be attempted when comminution is minimal. Poor results after anatomic reduction may still occur with all types of acetabular fractures due to the magnitude of the initial trauma (44).
Premature closure of the triradiate cartilage is a potential complication that is unique to the immature skeleton (Fig. 34-4) (45). Fractures that cause premature closure are usually nondisplaced and do not require open reduction. Children younger than 10 years are at the greatest risk for this complication. Disturbance in growth leads to the development of a shallow acetabulum because the triradiate cartilage is responsible for growth in the height and width of the acetabulum. Hip subluxation may follow, necessitating redirectional pelvic osteotomy.
Complications.
Immediate management of pelvic fractures and associated injuries can be challenging, but long-term complications are rarely attributable to the bony structures. Residual morbidity is more often due to associated injuries, especially traumatic brain injury. Untreated, displaced fractures can result in a limp, back pain, rotational deformity, or limb-length discrepancy. Acetabular injuries are at risk for developing traumatic arthritis in spite of anatomic reduction. Premature closure of the triradiate cartilage can be problematic in younger children.
FRACTURES AND DISLOCATIONS OF THE HIP
Hip Dislocation.
Traumatic dislocation of the hip in children is uncommon, representing only 5% of all pediatric dislocations. Most hip dislocations are posterior, but anterior and obturator dislocations can occur (46, 47). The mechanism of
injury depends somewhat on the age of the child. Hip dislocations in children younger than 8 years are frequently the result of mild trauma because joint laxity is common and the acetabulum is largely cartilaginous (Fig. 34-5) (47). Dislocations in children older than 8 years are more often the result of moderate or severe trauma. Dislocation from moderate trauma may result in spontaneous, incongruous reduction and capsular interposition (48). This is rare but easily misdiagnosed in children and adolescents. Any suggestion of joint-space widening should be investigated with a CT scan. An MRI may be valuable to visualize any cartilage and labrum that may be an impediment to reduction in the immature nonossified acetabular rim (Fig. 34-6).
injury depends somewhat on the age of the child. Hip dislocations in children younger than 8 years are frequently the result of mild trauma because joint laxity is common and the acetabulum is largely cartilaginous (Fig. 34-5) (47). Dislocations in children older than 8 years are more often the result of moderate or severe trauma. Dislocation from moderate trauma may result in spontaneous, incongruous reduction and capsular interposition (48). This is rare but easily misdiagnosed in children and adolescents. Any suggestion of joint-space widening should be investigated with a CT scan. An MRI may be valuable to visualize any cartilage and labrum that may be an impediment to reduction in the immature nonossified acetabular rim (Fig. 34-6).
Treatment.
Treatment consists of closed reduction with adequate muscle relaxation and analgesia. Whenever possible, closed reduction within 6 hours of injury is recommended. Reduction is rarely difficult in children, but gentle reduction is especially important in the adolescent age group. Reduction with image guidance in the operating room should be considered in the adolescent age group because occult epiphyseal injury may be present, and epiphyseal separation can occur during attempted reduction (49). The reduction technique for posterior dislocation requires hip and knee flexion, usually with adduction of the hip. Longitudinal traction is then applied while an assistant stabilizes the pelvis. The limb is then extended, internally rotated, and abducted. After the hip is reduced, the stable arc of motion should be assessed. Postreduction radiographs should include the opposite hip to confirm a concentric reduction with symmetrical joint spaces. A CT and possibly an MRI scan are recommended after hip reduction in children older than 10 years or in younger children if instability or joint-space widening is noted. A CT scan is not necessary in the younger child with a stable concentric reduction and no evidence of acetabular fracture on plain radiographs. The child younger than 8 years should be immobilized in a spica cast for 4 to 6 weeks to reduce the risk of recurrent dislocation. Stable, closed reductions in older children can be managed with activity restriction and decreased weight bearing for 6 weeks to allow capsular healing and reduction of posttraumatic inflammatory response.
The indications for open reduction include unstable closed reduction, nonconcentric reduction, bone or soft-tissue fragments within the joint, or a large acetabular rim fragment with instability. Open reduction is recommended from the direction of the dislocation, such as the posterior interval (i.e., Kocher-Langenbeck approach) for posterior dislocations. Postoperative management after open reduction is the same as that after closed reduction.
Complications.
The reported incidence of avascular necrosis in children is 3% to 10%, approximately half in the rate of adults. The risk of avascular necrosis is diminished when reduction is achieved within 6 hours of injury (46, 47). Nerve injury has been reported in 5% of children with hip dislocation, but recovery occurs spontaneously in 60% to 70% of these patients (50). The long-term risk of arthritis is related to the severity of the trauma, associated fractures, and treatment delays beyond 24 hours. However, some satisfactory results have been reported when neglected traumatic dislocations have been treated by traction and open reduction (51). Coxa magna in the absence of avascular necrosis has been observed in many children after traumatic hip dislocation. The cause is probably reactive hyperemia secondary to extensive soft-tissue injury. Coxa magna does not seem to influence clinical outcome.
Hip Fractures.
Hip fractures in children represent <1% of all pediatric fractures (1). In contrast to adult hip fractures, pediatric and adolescent hip fractures are usually the result of high-energy trauma because considerable force is required
to produce a fracture in this age group. The exceptions to this are infants who have been subjected to child abuse, and fracture through a pathologic lesion of the femoral neck (e.g., bone cyst). Complications are frequent and have been reported in 15% to 60% of patients (52, 53). Prompt and appropriate management may reduce the risk of subsequent complications.
to produce a fracture in this age group. The exceptions to this are infants who have been subjected to child abuse, and fracture through a pathologic lesion of the femoral neck (e.g., bone cyst). Complications are frequent and have been reported in 15% to 60% of patients (52, 53). Prompt and appropriate management may reduce the risk of subsequent complications.
Anatomy and Classification.
In the infant, the proximal femur is composed of a single large cartilaginous growth plate (54). The medial portion becomes the epiphyseal center of the femoral head, ossifies at around 4 months of age, and forms the proximal femoral physis. The lateral portion of the proximal femur forms the greater trochanter physis, with ossification of the epiphysis by 4 years of age. Injury to the proximal femur can affect one or both of these centers of growth. The proximal femoral physis is responsible for the metaphyseal growth of the femoral neck and provides approximately 15% of the total length of the femur. The greater trochanter helps shape the proximal femur, and damage to this apophysis in children younger than 8 to 10 years may produce an elongated, valgus femoral neck (55, 56).
The vascular supply of the growing child’s proximal femur is jeopardized by these fractures, and the extent of damage greatly affects the final outcome. The dominant arterial source for the femoral head is the lateral epiphyseal vessels, which are the terminal extension of the medial femoral circumflex artery. These vessels penetrate the capsule at the base of the neck near the piriformis fossa and run along the lateral periosteum giving off several branches to the femoral neck before entering the femoral head just proximal to the epiphyseal cartilage (Fig. 34-7) (57, 58). The lateral circumflex system can supply blood to a portion of the anterior femoral head until 2 to 3 years of age, after which it primarily supplies the metaphysis. In children older than 14 to 18 months, the proximal femoral physeal plate becomes an absolute barrier to the metaphyseal blood supply and prevents direct vascular penetration of the femoral head (57, 58). Thus, the epiphyseal and metaphyseal circulation remain separate until complete physeal closure occurs. The vessels of the ligamentum teres do not contribute a significant portion of the blood supply to the femoral head, especially in children younger than 8 years.
It is postulated that some displaced fractures may leave the vascular leash intact but kinked and occluded until realignment is established (59). This has been demonstrated by arteriography before and after reduction of an unstable slipped capital femoral epiphysis (60). Vascular disruption as a cause of avascular necrosis is supported by the fact that the magnitude of displacement is a prognostic factor for the development of necrosis (61). It has also been suggested that prompt decompression of the intracapsular hematoma contributes to the restoration of normal vascular flow and reduces the incidence of femoral head necrosis (62, 63, 64 and 65).
A study of nondisplaced hip fractures in adults confirmed high intracapsular pressures with decreased blood flow on bone scan. Following aspiration and fixation, repeat bone scans demonstrated restoration of blood flow (66). Other studies have also reported high intracapsular pressures that are reduced by joint decompression (66, 67 and 68). Soto-Hall et al. (69), in 1964, noted that intra-articular pressures increased when intracapsular hip fractures were manipulated by placing the leg in internal rotation and extension. The increased joint pressure during reduction of fractures in this position has been confirmed by other authors (66, 68). Reduction of intracapsular fractures may improve vascularity by restoring normal arterial position. However, fracture reduction may also lead to increased intracapsular pressure unless the hip is decompressed. Prompt anatomic reduction, internal fixation, and decompression are recommended in order to restore circulation in a timely manner. This approach to the management of hip fractures and unstable slipped capital femoral epiphyses in children has been associated with a decreased risk of avascular necrosis (62, 63, 65, 70).
Delbet’s classification (Fig. 34-8) offers a useful system for the treatment and prognosis of proximal femur fractures (71). Type I fractures are transphyseal separations. Physeal separation in infants is occasionally seen as a birth fracture or as a result of intentionally inflicted injury. Obstetric fracture separations have excellent clinical results, without avascular necrosis, although diagnosis and treatment may be delayed (72). Children younger than 2 years with type I fracture also have a good prognosis without surgical management (73). Transepiphyseal separations in older children result from more severe trauma, but separation has been reported during reduction of hip dislocation in the adolescent age group (74). When the epiphyseal fragment is dislocated from the acetabulum, the risk of avascular necrosis approaches 100%. However, the incidence of avascular necrosis is variable when the femoral head remains within the joint (71).
Type II fractures occur in the neck of the femur between the epiphyseal plate and the base of the neck. These injuries constitute approximately 50% of all fractures of the proximal femur (71). Complications are frequent with type II fractures. The incidence of avascular necrosis approaches 50% to 60%, and the nonunion rate is 15%. Premature physeal closure may also occur, but because growth of the proximal femur is approximately 15% of the total limb (75), clinically important leg-length discrepancy is unlikely to occur in older children.
Type III fractures occur in the cervicotrochanteric, or basal neck, region of the femoral neck. This is the second most common type of hip fracture in children. Avascular necrosis occurs in 30% of displaced fractures. Malunion has been reported in 20%, and nonunion occurs in 10%, of these patients. These problems may be lessened by precise fracture reduction, combined with compression across the fracture site by means of cancellous bone screws (i.e., lag technique) (71, 76).
Type IV fractures occur in the intertrochanteric region and are associated with the least risk of damage to the femoral head vascular supply. The incidence of avascular necrosis is between 0% and 10%. Varus deformity is the most likely complication, but this may correct with growth in younger children (71, 76, 77).
Treatment.
Proximal femur fractures should be treated as urgently as possible. The risk of avascular necrosis may be lower with reduction, joint decompression, and stable fixation within 24 hours of injury (53, 62, 64, 78). Delay in treatment may be necessary because of associated injuries or other considerations. A recent review of pediatric femoral neck fractures concluded that the quality of reduction and timing of surgery (<24 hours) were associated with decreasing the risk of AVN (79).
Type I Fractures.
Treatment with closed reduction and casting is appropriate for minimally displaced fractures in children younger than 2 years (73). In children aged 2 to 12 years, stabilization of the reduced fracture may be accomplished with two smooth pins supplemented with spica casting. In older children, fixation across the physis is recommended. Open reduction is often necessary if the epiphysis is dislocated. This is performed through a posterior approach for posterior fracture dislocations. At the time of open reduction, curettage of the physeal plate has been recommended in an attempt to encourage revascularization of the femoral head (74).
Type II and III Fractures.
If the fracture is stable and nondisplaced, and the patient is younger than 6 years, a spica cast alone can yield good results (80). Displaced fractures can usually be reduced by closed methods, but a capsulotomy may decrease the risk of avascular necrosis (62, 63, 64 and 65). Ng and Cole (64) studied the effect of early hip decompression on the frequency of avascular necrosis. It had no apparent value in type I fractures. For the type II and III fractures, 41% of 54 patients treated without hip decompression developed avascular necrosis, whereas only 8% of 39 patients with hip compression developed avascular necrosis. As previously discussed, a prompt anatomic reduction, internal fixation, and decompression are recommended by the present authors in an attempt to restore circulation in a timely manner. Fixation is achieved by the percutaneous insertion of two or three cannulated bone screws into the metaphyseal portion of the proximal fragment. If the proximal metaphyseal fragment is too small for secure fixation, smooth pins can be placed across the physis to allow subsequent growth. Stable fixation of the fracture should be given priority over preservation of the proximal femoral physis (71). Spica cast immobilization is used to augment fixation in children, especially when smooth pins have been used. In patients aged 12 years or older, threaded screws may be placed across the physis for better fixation and to avoid the use of a spica cast. Alternatively, a hip screw with a supplemental pin to control rotation may be used in older children. Caution is advised when using compression hip screws in children because dense bone may generate heat necrosis of the femoral neck during reaming. An additional smooth pin is recommended to improve rotational stability. The authors recommend an open reduction if suitable alignment is not obtained by closed means. The quality of reduction is associated with outcomes (79). An anterolateral (Watson-Jones) approach is recommended for type II fractures (Fig. 34-9).
Type IV Fractures
This fracture does not require prompt stabilization except when surgical fixation improves general management. Nondisplaced fractures in this region can be managed by spica cast immobilization and close follow-up in younger children. Displaced fractures in infants and toddlers may be treated with early closed reduction and casting so long as the neck-shaft angle does not decrease to <115 degrees. Displaced fractures in older children can also be managed by skeletal traction followed by cast immobilization. However, the authors recommend surgical stabilization in children older than 6 years to reduce the risk of malunion and avoid prolonged immobilization. Interfragmentary screws may provide sufficient stability when combined with cast immobilization, but a pediatric-sized hip screw with side plate, or an angled blade plate, is preferred (Fig. 34-10). Adolescents are treated in the same manner as adults, with stable fixation across the physis using a sliding hip screw or an angled blade plate. This avoids the need for a supplemental spica cast for adolescent patients.
Complications.
The most frequent complications after fracture of the proximal femur in children are osteonecrosis of the femoral head, malunion, and nonunion. Other complications include infections, premature closure of the proximal femoral growth plate, and chondrolysis. Exact complication rates are difficult to determine because of changing patterns of treatment. Prompt treatment, accurate reduction, joint decompression, and appropriate internal fixation and immobilization reduce all of these complications (53, 64).
Avascular necrosis in children may involve a portion of the femoral head, just the portion of the femoral neck between the fracture and the physis, or the entire femoral neck and head (81). Necrosis of the femoral head occurs in approximately 30% of all hip fractures in children and often leads to poor results (71). Diagnosis of avascularity can be reliably determined by MRI as early as 2 weeks following the fracture (82), or by isotope bone scan 4 months after the fracture. Children with osteonecrosis who are younger than 12 years can be treated with containment, with or without prolonged non-weight-bearing activities. These patients have a possibility of recovering satisfactory function (83, 84). The outcome for older children with osteonecrosis of the femoral head is poor. The authors recommend treating adolescents in a manner similar to that for adults, with early detection and core decompression before the occurrence of subchondral fracture (85).
Nonunion occurs in 6% to 10% of pediatric fractures of the proximal femur (71). Treatment is recommended as soon as the diagnosis is established. Subtrochanteric valgus osteotomy is preferred, with bone grafting, internal fixation, and application of a spica cast (71, 86). Supplemental vascularized bone grafting with a vascular-pedicle graft from the iliac crest should be considered when there is a large defect in the femoral head or neck (87).
Malunion and coxa vara occur in approximately 20% of reported patients, but this complication has a lower incidence when internal fixation is used (71). Remodeling may occur in younger patients (77). Subtrochanteric osteotomy is recommended for persistent deformity.
FEMORAL SHAFT FRACTURES
Femoral shaft fractures account for 1% to 2% of all fractures in childhood (1, 88). There is a bimodal age distribution, with a peak incidence at 2 to 3 years of age and another peak in adolescence. The cortical thickness of the femur increases rapidly after 5 years of age, and this may explain the decreasing incidence of femur fracture in late childhood. Intentional injury should always be considered in children who are not yet walking, but there are no distinguishing age, clinical parameter, or fracture patterns to help determine which injuries are inflicted and which are accidental (89). In infants younger than 1 year, child abuse has been identified as a cause in 65% of patients when obvious causes such as motor vehicle accidents are eliminated (89). Abuse should be indicated in any child with a femur fracture from 0 to 3 years with the greatest incidence in those younger than 1 year or walking age. Children in the toddler age group may sustain fractures with relatively minor trauma from causes such as falling from a low height or tripping while running. In the 4- to 7-year age group, approximately half of the femoral shaft fractures are caused by bicycle accidents. In the adolescent age group, motor vehicle accidents account for most of the femur fractures.
As stated by Rang (90), “It does not require a physician to diagnose a fractured femur.” However, the physician must carefully examine the patient completely. Children and adolescents with femur fracture have a 35% to 40% incidence of associated injuries. Some of these injuries are occult, such as femoral neck fracture, hip dislocation, ligamentous instability of the knee, and visceral injuries (91, 92). Hemodynamic instability or steadily declining hematocrit does not occur because of an isolated, closed femur fracture. Other sources of blood loss must be sought in these patients (92).
Treatment of Femoral Shaft Fractures
Principles of Management.
A wide variety of management options are currently available for femur fractures in children and adolescents. Each of these options can yield satisfactory results when used properly (93). The surgeon managing femoral fractures in children and adolescents is expected to select and perform the technique that is most appropriate under a variety of circumstances. As long as proper technique is used, there may be more than one acceptable option for treatment.
The age of the child and the fracture characteristics are the principal determinants of management. At one end of the spectrum, there are low-energy injuries in young children that are managed with closed reduction and immediate spica casting. At the other end of the spectrum, high-energy injuries in adolescents are managed with early surgical stabilization. Occasionally, however, infants with severe trauma require surgical stabilization of femoral fractures to facilitate management.
Anatomical Considerations and Remodeling Potential.
Proximal fractures in all age groups are more difficult to control, but residual deformity is better tolerated because of multidirectional hip motion. Deformity is also less obvious with proximal fractures because thick thigh muscles hide residual angulation. Proximal fragments tend to flex, abduct, and externally rotate because of the unopposed action of the iliopsoas, hip abductor, and external rotator muscles. The proximal fragment of a midshaft fracture also tends to flex, abduct, and externally rotate, but the deformity is less extreme because of adductor and hamstring attachments to the proximal fragment. More distal fragments are easier to control and produce little proximal fragment angulation, except for supracondylar fractures, which tend to hyperextend because of the posterior pull of the gastrocnemius muscle on the short distal fragment. Fractures of the distal femur require more precise alignment because the deformity is more visible and remodeling in the coronal plane is limited. Supracondylar fractures of the femur require management that is similar to that for distal femoral physeal separations (94).
Children have remarkable remodeling potential until approximately 10 years of age in girls and 12 years of age in boys. Long-term studies have demonstrated that up to 25 degrees of midshaft angulation in any plane can be expected to correct satisfactorily in children younger than 13 years (95). Remodeling continues for up to 5 years following fracture. Approximately 25% of remodeling occurs at the fracture site, whereas 75% is attributed to physeal reorientation and longitudinal growth (96, 97). Fracture translation may also contribute to realignment of the mechanical axis. Rotational remodeling may also occur, but the precise amount is unpredictable (98, 99). Clinically significant rotational deformity is uncommon, even when failure of remodeling has been documented (100, 101).
Stimulation of growth after femoral fracture occurs routinely in the 2- to 9-year age group (102, 103). Unfortunately, most authors reporting the overgrowth phenomenon do not distinguish between “catch-up growth,” which compensates for fracture overriding; and true overgrowth, which results in the fractured femur being longer than normal. Growth stimulation is greater when overriding is greater and may continue for 5 years after fracture. Average total growth stimulation is approximately 1 cm. In a prospective study of children younger than 12 years, Hougaard observed that all fractures of the femur healing with 3 cm or less discrepancy with the contralateral leg spontaneously recovered to <2 cm discrepancy (103). Therefore, any femoral fracture that heals in a shortened position should be observed for several years to determine the final outcome. True overgrowth has been reported after reduction with internal fixation, but the risk of overgrowth in this circumstance has not been clearly defined (93).
Management Guidelines by Age.
Age or weight is the first determinant of treatment choices. Age is used here with the understanding that the children are of average weight and maturity.
Infants to 6 months.
Infants younger than 1 year often sustain femur fractures because of birth trauma or abuse. The incidence of child abuse in femur fractures is up to 14% in this age group (104, 105 and 106). Osteogenesis imperfecta and other metabolic disorders should also be suspected. Thick periosteum
and rapid hematoma consolidation usually prevent worrisome shortening or angulation. Healing is rapid, and remodeling potential is great. Infants may be treated with application of a Pavlik harness or a conventional spica cast (107). Both methods provide patient comfort and minimal complications (107). Immobilization for 2 to 3 weeks is usually sufficient for infants <4 months, and 4 to 6 weeks for infants aged 6 to 12 months.
and rapid hematoma consolidation usually prevent worrisome shortening or angulation. Healing is rapid, and remodeling potential is great. Infants may be treated with application of a Pavlik harness or a conventional spica cast (107). Both methods provide patient comfort and minimal complications (107). Immobilization for 2 to 3 weeks is usually sufficient for infants <4 months, and 4 to 6 weeks for infants aged 6 to 12 months.
Age 6 months to 5 Years.
Isolated femur fractures in children between the ages of 6 months and 5 years are usually treated with early spica cast application. In low-energy fractures with minimal shortening, a walking spica (cylinder long leg cast, 30 to 40 degrees of hip and knee flexion, with a pelvic band and the uninjured leg free) works well and is easier on the patient and the family. All aspects of spica cast treatment are easier for preschool children than for older children (108). Children in this age group also heal rapidly; thus, immobilization time is brief. The spica cast may be applied in the operating room or emergency department under conscious sedation. Splinting may be used for comfort until a spica cast can be applied within 48 hours after injury. Careful cast application is indicated to prevent compartment syndrome. Compartment syndrome can occur if significant pressure is placed on the calf with excessive traction especially if a short leg cast applied first and used to pull 90/90 traction while applying the remainder of the spica (109). Alignment in the cast should be as close to normal as possible, but full functional recovery can be expected in this age group if shortening at the time of union is >3 cm and angulation is <20 degrees in any plane. Fractures in the distal third should be angulated no more than 15 degrees. Close radiographic follow-up is required in the first 2 to 3 weeks to evaluate for unacceptable shortening or angulation. Other techniques should be considered if the fracture shortens >3 cm and angulation cannot be corrected with cast wedging. These techniques may be traction, temporary external fixation, or elastic nails. Other treatments such as elastic nails may be chosen in these younger preschool years dependent on fracture shortening and social concerns (110, 111).
Age 5 to 11 Years.
Children between the ages of 5 and 11 years may be managed by a wide variety of methods depending on the fracture characteristics and surgeon preference. Flexible intramedullary nails are a common and proven successful method for treatment in this age group especially for stable transverse diaphyseal fractures. Historically, skeletal traction followed by spica casting has been used and is still an acceptable option for treatment. However, children treated with flexible elastic nails have a more rapid return to walking and school (112) and less hospital costs than traction and casting (113). High-energy unstable fractures with severe displacement and comminution are more challenging to treat. Flexible nails can be used with good technique, yet other methods such as submuscular plating (Figs. 34.11, 34.12, 34-13, 34-14, 34-15, 34-16 and 34.17) or external fixation are good alternatives in the unstable fracture patters (114). A final option in unstable fractures that is rarely used is incorporating a traction pin in the spica cast or by traction for 2 to 3 weeks before cast immobilization to keep the fracture aligned and out to length during the period of early callus formation (115, 116 and 117). Although it has been demonstrated that up to 25 degrees of angulation in any plane will remodel in this age group (118), recommended guidelines for alignment in this age group are up to 15 degrees varus or valgus, 15 degrees of anterior and/or posterior angulation, and up to 20 mm of shortening.
Age 11 Years to Maturity.
Children older than 11 years are generally managed by surgical stabilization with internal or external fixation (93). Accurate restoration of length and alignment is desirable in older children because of limited remodeling potential. No more than 10 degrees of varus or valgus, 10 degrees of anterior or posterior angulation, and 15 mm of shortening should be accepted in this age group. Surgical stabilization permits early mobilization and return to school and social activities. The various techniques for stabilization include trochanteric entry nailing, submuscular plating, and flexible intramedullary nails. Flexible intramedullary nails (Figs. 34-18, 34-19, 34-20, 34-21 and 34-22) have a slightly higher complication rate in this age group than the younger ages (119). It is generally agreed that rigid, reamed intramedullary nailing through a piriformis fossa should be avoided because of the risk of iatrogenic avascular necrosis of the femoral head (120). The risk of this complication is present as long as the proximal femoral physis remains open.
This procedure is indicated for patients ≥5 years until skeletal maturity. Submuscular plating is ideally suited for comminuted or long-oblique length unstable femur fractures. Submuscular plating is also a good option for proximal or distal one-third femur fractures. In the proximal and distal one-third fractures, there needs to be enough room for two to three screws in the proximal or the distal diaphysis.
FLEXIBLE INTRAMEDULLARY NAILING OF FEMORAL SHAFT FRACTURES (FIGS. 34-18, 34-19, 34-20, 34-21, 34-22, 34-23 and 34-24).
The idea of internal stabilization of femoral shaft fractures in children is not new, having been performed by Kuntscher more than 50 years ago. Several factors, however, have brought about a renewed interest in this technique: realization of the factors that lead to a bad result with closed treatment, successful application and improvement of internal fixation of these fractures in adults, and inexperience in casting techniques among orthopaedic physicians. Cost, however, is not a factor that merits consideration. There is little difference in the cost of treating a child with flexible intramedullary rods, external fixation, or traction for 2 weeks in the hospital followed by spica cast (93, 121).
Factors that lead to a bad result with closed reduction and casting form the basis for understanding the indications for closed reduction and flexible intramedullary nailing of femoral shaft fractures in children. In children older than 9 or 10 years of age, it is often difficult to maintain acceptable alignment of the fracture because of the child’s size, and this has led to the preferable use of some alternative to cast treatment in the adolescent (122, 123 and 124).
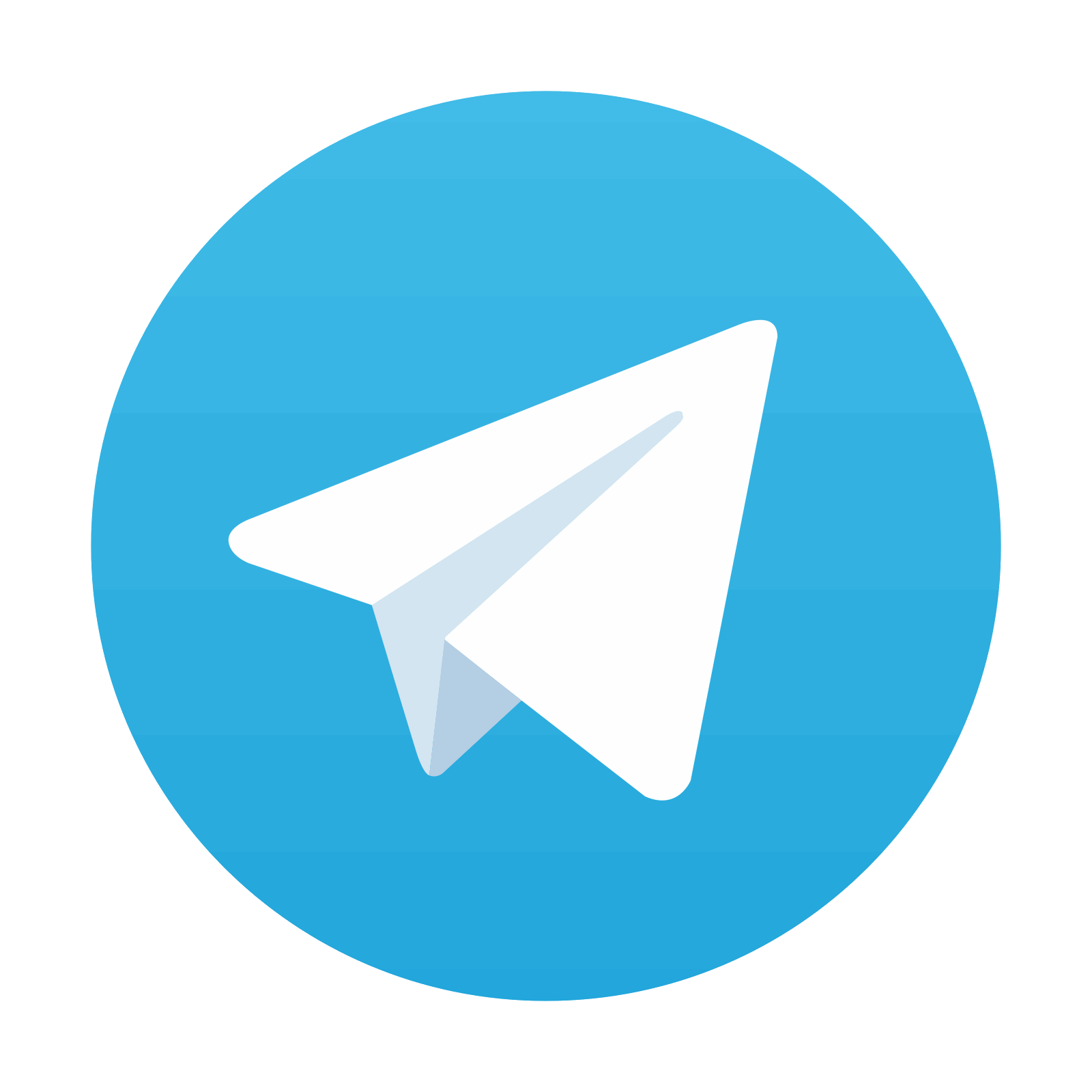
Stay updated, free articles. Join our Telegram channel

Full access? Get Clinical Tree
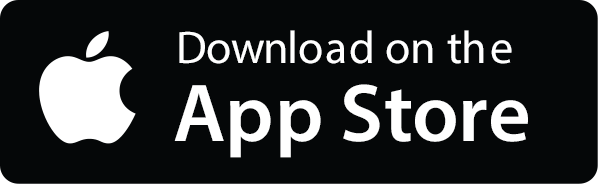
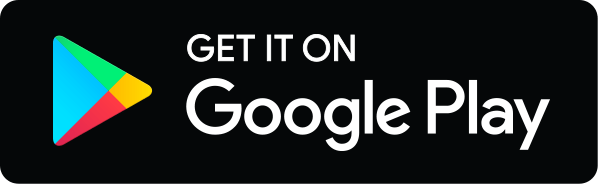
