The Wrist and Hand
David W. Stoller
Arthur E. Li
David M. Lichtman
Gordon A. Brody
In the evaluation of both normal anatomy and pathology of the wrist and hand, magnetic resonance (MR) imaging is a standard at hand and radiology society meetings. With MR imaging, it is possible for the radiologist to accomplish accurate, noninvasive imaging of specific ligamentous injuries, rendering the vague diagnosis of “wrist sprain” obsolete. As data on the biomechanics of the carpus are collected, applications are being developed for fast imaging techniques. As techniques for dynamic MR imaging of the carpus advance, these methods may become the standard for evaluating instability. This instability can best be defined as the inability of two bones or groups of bones to maintain a normal physiologic relationship.
Status of Imaging Techniques
Standard Radiography
Standard radiographic evaluation of the wrist and hand is restricted primarily to demonstrating the osseous structures. With certain localized pathologic processes, select views, such as a scaphoid and carpal tunnel view, may provide additional information. The scaphoid fat stripe, which can be identified radial to the scaphoid, and the pronator quadratus line, which is frequently obscured by fracture, are shown on posteroanterior (PA) and lateral radiographic views, respectively. However, the usefulness of the scaphoid fat stripe in diagnosing acute scaphoid fracture has been challenged. On a lateral radiograph, the static bony relationships of the radius, lunate, and capitate can be measured in longitudinal axes.
Arthrography
Wrist arthrography has been used to evaluate the integrity of the triangular fibrocartilage (TFC) and the scapholunate and lunotriquetral interosseous ligaments.1,2,3,4 The three-compartment (i.e., triple injection) arthrogram, in which contrast is introduced into the radiocarpal, distal radioulnar, and midcarpal joints, was considered the standard technique.5,6 Subsequently, single-compartment arthrograms of the radiocarpal joint have been shown to have a false-negative rate of only 2% for complete perforations and 10% for complete and partial perforations together, and no additional information was provided by selective second and third injections of the distal radioulnar and midcarpal joints.7
Although arthrographic findings correlate quite well with ulnar-sided wrist pain, the technique is far less effective for radial-sided problems. Manaster et al.8 found that although 88% of patients with ulnar pain had lunotriquetral ligament perforations, only 26% of patients with scapholunate dissociation had scapholunate ligament perforations.8 Arthrography, therefore, appears to be less useful in assessing the physiologic integrity of the interosseous ligaments on the radial side of the wrist.
Wrist arthrography studies (using triple-compartment technique) demonstrate poor correlation of the locations of unidirectional or bidirectional communicating defects, as well as noncommunicating defects, with the site of wrist pain.9,10 Another limitation of arthrography is related to the fact that because of the nature of the arthrography technique, it is impossible to differentiate small, pinhole perforations from those that are large and biomechanically significant. Anatomic studies have shown that degenerative perforations of both the interosseous ligaments and the TFC complex are quite common in people older than 35 years of age.11,12 Therefore, arthrography is less diagnostically useful in these patients. Kirschenbaum et al.13 have also shown the common arthrographic finding of TFC and intrinsic ligament perforations in young asymptomatic adults. This apparent lack of specificity of arthrography may limit its application, especially in comparison with MR imaging, in which the morphology of the TFC and intrinsic ligaments can be evaluated directly. When used, MR arthrography involves a single-compartment radiocarpal injection of contrast. We do not use conventional arthrography unless the arthrogram is part of an MR imaging evaluation.
Computed Tomography
Computed tomography (CT) has limited but well-defined applications in the wrist. It is primarily used to evaluate occult or complex fractures, fracture healing, and lucent defects and to provide improved definition of osseous detail.14 Although subtle differences in closely related soft-tissue attenuation values cannot be optimally resolved with CT, it is an excellent modality for defining the location and extent of carpal bone fractures and complex intra-articular fractures of the distal radius.15,16 Multidetector CT arthrography has also been used to visualize interosseous ligament tears.17
Magnetic Resonance Imaging
MR imaging of the wrist provides the high spatial and contrast resolution of soft-tissue and osseous components needed for evaluation of the small and complex anatomy of the wrist and hand.16,18,19,20,21,22,23,24,25,26,27 Supporting muscles, ligaments, tendons, tendon sheaths, vessels, nerves, and marrow are demonstrated on MR images with excellent spatial resolution using the small fields of view (FOVs) and uniform signal intensity penetration. MR imaging has replaced conventional wrist arthrography in diagnosing tears involving the intercarpal ligaments and TFC complex by allowing direct correlation of abnormalities in ligamentous and fibrocartilage morphology with the clinical presentation of pain. Multiplanar images permit direct anatomic and pathologic discrimination in the axial, coronal, sagittal, and oblique planes. Sagittal MR images display bone and ligamentous anatomy in a selective “tomography-like” section, without the overlapping of carpal bones seen on lateral radiographs. This facilitates more accurate assessment of carpal instability. Fat-suppressed PD-weighted fast spin-echo (FS PD FSE) techniques have significantly improved visualization of wrist joint fluid, increasing the accuracy of routine wrist MR evaluations relative to MR arthrography.28 Foo et al.29 have used high-resolution spin-echo, 2D, and 3D gradient-recalled acquisitions in the steady state (GRASS), and spoiled GRASS (SPGR) images to optimize trabecular bone detail and anatomy of the wrist (Fig. 10.1).29 These techniques may need to be used in conjunction with FS or intra-articular contrast to improve identification of ligament and cartilage pathology.30 Local gradient coils have been used in 3D gradient-echo imaging and phase-contrast angiography of the fingers.31 We routinely use a dedicated eight-channel phased-array coil at 1.5 Tesla. 3T imaging of the wrist has demonstrated an improved signal-to-noise ratio, allowing for improved assessment of osseous ligamentous structures, tendons, cartilage, and nerves (Fig. 10.2). The increased chemical shift artifact observed at 3T can be adequately addressed by increasing the receiver bandwidth. The signal-to-noise ratio will, however, decrease as bandwidth is increased. This is not an issue when using routine receiver bandwidths of 25 to 41 kH.
MR imaging is used for the evaluation of ligamentous pathology, trauma (e.g., fracture), avascular necrosis (AVN), and Kienböck’s disease, as well as for abnormalities of the TFC and carpal tunnel. In addition, the status of synovium, articular cartilage, and cortical and subchondral bone response in arthritis can be assessed and categorized. Ultra-high-frequency sonographic transducers may be useful for ultrasound assessment of the dynamic function of the superficial tendons of the wrist and hand and thus complement MR studies.32
Imaging Protocols for the Wrist and Hand
The wrist and hand are imaged using a dedicated circumferential design phased-array coil to optimize the signal-to-noise ratio and to obtain high-resolution images. Signal-to-noise and contrast-to-noise are improved when high-field-strength (3T) magnets are used, allowing better visualization at the TFC complex, intercarpal ligaments, and cartilage. With this
coil design, the patient’s arm may be positioned at his or her side. Anatomic symmetry of both extremities can be demonstrated in the same FOV by placing both hands in a large-diameter coil. When high-spatial-resolution images requiring smaller FOVs are necessary for the opposite wrist or hand, separate acquisitions can be performed in the area of suspected pathology and a comparison of normal and abnormal anatomy can be made. Proper positioning requires alignment of the long axis of the distal radius and central metacarpal axis with the wrist in neutral position. Oblique prescriptions are not required to produce orthogonal images with this colinear alignment of the distal radius and carpus. Radial or ulnar deviation and dorsal or volar angulation should be avoided to maintain consistent alignment of the carpus. The wrist is usually positioned in pronation, with the fingers held in extension. The position of the wrist may change relative to the design of the surface coil used. When the wrist is studied in the thumbs-up position, coronal images are obtained by prescribing a sagittal plane acquisition. In this case, oblique imaging may be required to produce orthogonal plane images through the plane of the TFC and intrinsic ligaments of the wrist.
coil design, the patient’s arm may be positioned at his or her side. Anatomic symmetry of both extremities can be demonstrated in the same FOV by placing both hands in a large-diameter coil. When high-spatial-resolution images requiring smaller FOVs are necessary for the opposite wrist or hand, separate acquisitions can be performed in the area of suspected pathology and a comparison of normal and abnormal anatomy can be made. Proper positioning requires alignment of the long axis of the distal radius and central metacarpal axis with the wrist in neutral position. Oblique prescriptions are not required to produce orthogonal images with this colinear alignment of the distal radius and carpus. Radial or ulnar deviation and dorsal or volar angulation should be avoided to maintain consistent alignment of the carpus. The wrist is usually positioned in pronation, with the fingers held in extension. The position of the wrist may change relative to the design of the surface coil used. When the wrist is studied in the thumbs-up position, coronal images are obtained by prescribing a sagittal plane acquisition. In this case, oblique imaging may be required to produce orthogonal plane images through the plane of the TFC and intrinsic ligaments of the wrist.
Pearls and Pitfalls
Imaging Protocols
MR or MR arthrography has replaced conventional single- or three-compartment arthrograms.
Dedicated four- or eight-channel phased-array coils are required for wrist and finger imaging.
Although TFC degeneration is best demonstrated on T2* gradient-echo images, FS PD FSE sequences are more frequently used in routine examinations to provide improved contrast between hypointense intrinsic ligaments and hyperintense fluid.
Some typical protocols include the following:
T1- and PD-weighted images are obtained in the axial, coronal, and sagittal planes. Coronal images are acquired with 2- to 2.5-mm sections, using a 6-cm FOV and a 512 × 256 or 256 × 256 matrix.
Pathology of the TFC and intrinsic ligaments is displayed on FS PD FSE coronal images, which create an arthrography-like effect by displaying the hyperintensity of fluid in contrast to the lower signal intensity of ligaments and fibrocartilage. The sequence may also be used in the axial and sagittal planes.
FS PD FSE sequences use a repetition time (TR) of 3,000 msec, an echo time (TE) between 40 and 60 msec, an 8-cm FOV, a 2- to 3-mm slice thickness, and a 256 × 256 matrix interpolated to 512. Higher matrix and TE values and lower echo train lengths produce images with less blurring.
T2*-weighted coronal images also produce excellent contrast between ligaments (the intercarpal ligaments and the TFC complex) and fluid. In fact, intrasubstance TFC degeneration is best demonstrated using T2* gradient-echo techniques, even though the intrinsic ligaments are better visualized on FS PD FSE images.
3D SPGR techniques are used to display detailed anatomy of the TFC complex and intrinsic ligaments. Using these sequences, it is possible to achieve higher-resolution MR images with a FOV between 4 and 6 cm and a lower receiver band, ± 8 kHz. Pixel resolution at a 4-cm FOV and 256 × 256 matrix is approximately 100 μm, which allows visualization of trabecular bone detail as well. FS is recommended, however, to increase the conspicuity of fluid in abnormal or injured articular cartilage.
An axial STIR or FS PD FSE sequence demonstrates tenosynovitis, ganglions, carpal tunnel syndrome and related changes in the median nerve, and neoplasms. The distinct dorsal, membranous, and volar components of the scapholunate ligament are separated on axial images through the proximal carpal row. The flexor digitorum superficialis and profundus tendons can be differentiated on gradient-echo axial images through the phalanges.
Sagittal images display the static alignment of the carpal bones, which is important in assessing the capitolunate angle
and tilting of the lunate or the degree of scaphoid flexion or extension. The anteroposterior location of TFC tears is determined on FS PD FSE sagittal images. Fluid in the dorsal or volar ligaments of the capsule is also shown in this plane.
and tilting of the lunate or the degree of scaphoid flexion or extension. The anteroposterior location of TFC tears is determined on FS PD FSE sagittal images. Fluid in the dorsal or volar ligaments of the capsule is also shown in this plane.
Administration of intravenous gadolinium DTPA produces enhancement of pannus tissue and subchondral hyperemia in inflammatory arthritides. Scaphoid and lunate vascularity is studied using STIR, FS PD FSE coronal, or FS T1-weighted intravenous gadolinium-enhanced sequences. Scapholunate ligament complex visualization may also be improved with indirect MR arthrography.33
MR arthrography with intra-articular administration of an MR contrast agent or intra-articular saline improves the accuracy of detection of ligamentous disruptions, including flap tears and intercarpal fluid communications (Fig. 10.3).34 MR identification of small perforations of the TFC and intercarpal ligaments, with characterization of ligament morphology, provides more specific information than simply documenting contrast extension between the radiocarpal and midcarpal joints or radiocarpal and distal radioulnar joint, as provided by conventional arthrography.
Coupled 7.5-cm (3-inch) circular surface coils positioned in a kinematic wrist device have been used with gradient-echo protocols to track distal and carpal row motion with radial and ulnar deviation of the wrist. This information is displayed in a cine loop format and can be recorded on video or photographed.
Dorsiflexion and plantarflexion motions are best studied in the sagittal plane and require either a greater degree of freedom from the surface coil or pivoting of the coil to accommodate the increased range of motion. It is important to incorporate image quality considerations, however, when designing a surface coil with an increased diameter or anatomic coverage. Separate axial imaging sequences in positions of pronation and supination may be useful in the evaluation of subluxation patterns in the distal radioulnar joint.
Related Muscles of the Wrist and Hand
The related muscles of the wrist include the superficial and deep groups of the volar forearm muscles and superficial and deep groups of dorsal forearm muscles.
The superficial group of volar muscles includes the flexor carpi radialis (Fig. 10.4), the palmaris longus (Fig. 10.5), the flexor carpi ulnaris (Fig. 10.6), and the flexor digitorum superficialis (Fig. 10.7). The pronator teres is discussed in Chapter 9 on the elbow. The deep group of volar muscles includes the flexor digitorum profundus (Fig. 10.8), the flexor pollicis longus (Fig. 10.9), and the pronator quadratus (Fig. 10.10).
The superficial muscles of the dorsal aspect of the forearm include the extensor carpi radialis longus (Fig. 10.11), the extensor carpi radialis brevis (Fig. 10.12), the extensor digitorum (Fig. 10.13), the extensor digiti minimi (Fig. 10.14), and the extensor carpi ulnaris (Fig. 10.15). The brachioradialis and anconeus are discussed in Chapter 9 on the elbow. The deep group of dorsal muscles includes the abductor pollicis longus (Fig. 10.16), the extensor pollicis brevis (Fig. 10.17), the extensor pollicis longus (Fig. 10.18), and the extensor indicis (Fig. 10.19).
The muscles of the hand include the abductor pollicis brevis (Fig. 10.20), the opponens pollicis (Fig. 10.21), the flexor pollicis brevis (Fig. 10.22), the adductor pollicis (Fig. 10.23), the palmaris brevis (Fig. 10.24), the abductor digiti minimi (Fig. 10.25), the flexor digiti minimi brevis (Fig. 10.26), the opponens digiti minimi (Fig. 10.27), the lumbricals (Fig. 10.28), and the dorsal (Fig. 10.29) and palmar (Fig. 10.30) interosseous muscles.
![]() FIGURE 10.24 ● PALMARIS BREVIS The palmaris brevis is a thin superficial muscle that connects the flexor retinaculum to the ulnar skin. Rarely it is hyperactive, resulting in spasm. |
MR Anatomic Atlas of the Wrist and Hand
Coronal Images
Coronal plane images (Fig. 10.31) are important in understanding the relationship between the cartilaginous and ligamentous structures of the wrist. Coronal images demonstrate the TFC and the intrinsic ligaments in the same plane:
On volar images, the flexor retinaculum is seen superficial to the flexor tendons as a transverse band.
En face, the hypointense bands of the flexor digitorum tendons are seen passing through the carpal tunnel between the hook of the hamate and the trapezium.
The intermediate-signal-intensity median nerve may also be discerned in this plane of section.
The pisohamate and pisometacarpal ligaments are shown in sections at the level of the hook of the hamate and pisiform.
The abductor pollicis longus and extensor pollicis brevis tendons border the volar radial aspect of the wrist in sections through the volar surfaces of the scaphoid and lunate.
The TFC is seen as a curvilinear bowtie band of hypointense, homogeneous signal intensity. The band extends horizontally to the base of the ulnar styloid process from the ulnar surface of the distal radius.
The meniscal homologue demonstrates intermediate signal intensity on T1- and T2*-weighted images.
The radioscaphocapitate ligament and the radiolunotriquetral ligament, also sometimes referred to as the long radiolunate ligament, are visualized volarly and extend from the radial styloid in an ulnar-distal direction. These fibers are seen as parallel bands of striations. The more ulnarly located radioscapholunate ligament is usually seen in the same plane as the radioscaphocapitate and radiolunotriquetral ligaments and is a less substantial structure compared with the other volar extrinsic carpal ligaments. The proximal portion of the radiolunotriquetral ligament is represented by obliquely directed fibers extending from the volar radius to the lunate, volar to the proximal pole of the scaphoid.
The distal radioulnar joint and compartment are separated from the radiocarpal compartment by the TFC.
The scapholunate and lunotriquetral interosseous ligaments are routinely visualized on 3-mm coronal T1- and T2*-weighted images.
The extensor carpi ulnaris tendon borders the ulnar aspect of the wrist on the same coronal sections that display the TFC and interosseous ligaments.
The radial collateral ligament may be partially visualized between the scaphoid and radial styloid.
The articular cartilage surfaces of the carpal bones demonstrate intermediate signal intensity on T1-weighted images and increase in signal intensity on T2*-weighted images.
On dorsal images through the carpus, the interosseous ligaments of the distal carpal row can be defined. Dorsally, the obliquely oriented extensor digiti minimi tendon on the ulnar side of the triquetrum and the extensor carpi radialis longus tendon are seen. Lister’s tubercle, which contains fatty marrow, is situated between and separates the ulnar aspect of the extensor pollicis longus from the radial aspect of the extensor carpi radialis brevis. The dorsal interossei muscles are demonstrated between the midcarpal shafts.
Axial Images
Axial images (Fig. 10.32) define the dorsal, membranous, and volar components of the scapholunate and lunotriquetral intrinsic ligaments. The axial plane demonstrates the extensor and flexor tendons and the carpal tunnel in cross-section:
The flexor digitorum superficialis and profundus tendons are seen as tubular hypointense structures with invested synovial sheaths.
In proximal sections, the flexor pollicis longus is seen deep to the median nerve. Distally, it is flanked by the adductor pollicis medially and by the thenar muscles laterally, toward the thumb.
At the level of the distal radioulnar joint, the volar distal radioulnar ligament is identified as a thin, hypointense band, deep to the flexor digitorum profundus tendons and Parona’s space. The position of the distal ulna in relation to the sigmoid notch is determined at this level.
The TFC complex is displayed on the ulnar aspect of the ulnar styloid.
The curve of the ulnolunate ligament is demonstrated at the level of the proximal lunate and distal radius, where it follows the contour of the ulnar and volar aspect of the lunate.
The palmaris longus tendon is superficial to the median nerve.
The thin hypointense flexor retinaculum spans the palmar border of the carpal tunnel. Its distal attachments to the hook of the lunate and tubercle of the trapezium are more reliably defined than the proximal attachments to the tubercles of the pisiform and scaphoid.
The separate extensor tendons of the extensor carpi ulnaris, extensor digiti minimi, extensor digitorum and indicis, extensor carpi radialis brevis, extensor pollicis longus, and extensor carpi radialis longus are displayed from the ulnar to the radial dorsal aspect of the wrist.
The lunotriquetral and scapholunate ligaments are usually demonstrated at the level of the proximal carpal row.
The arcuate ligament is seen volar to the capitate and deep to the flexor tendons.
The radial collateral ligament is closely applied to the radial surface of the scaphoid.
The palmaris longus tendon is superficial to the median nerve and the flexor retinaculum.
The two central tendons of the superficial flexor group are located superiorly within the carpal tunnel before they fan out to their insertions on the middle phalanx.
On axial plane images, it is possible to differentiate the four separate tendons of the flexor profundus group.
The lumbrical muscle origins are seen deep to the flexor tendons on axial sections through the distal carpal tunnel and demonstrate intermediate signal intensity.
The median nerve, also of intermediate signal intensity, can be identified in the superficial radial aspect of the carpal tunnel.
On axial images through the midmetacarpals, the flexor tendons are seen anterior to the palmar interossei muscles, whereas the dorsal interossei are seen lying between the metacarpal bones.
Blood vessels display low signal intensity, except in venous structures demonstrated by even-echo rephasing or paradoxical enhancement secondary to slow flow. With gradient-echo techniques, both arterial and venous structures demonstrate hyperintensity.
Sagittal Images
The sagittal imaging plane (Fig. 10.33) is routine in wrist protocols. It is especially useful in the evaluation of static instability patterns and wrist shortening (i.e., proximal migration of the capitate) and in viewing the volar-to-dorsal aspect of the TFC. Kienböck’s fracture and fracture deformity (i.e., humpback scaphoid) are seen on sagittal images, complementary to coronal or axial images:
The abductor pollicis longus and extensor pollicis brevis tendons can be seen on radial sagittal images.
The scaphoid is identified on sagittal sections through the trapezium and, more dorsally, the trapezoid.
The hypointense radioscaphocapitate ligament is represented by fibers seen along the volar aspect of the scaphoid between the volar distal radius and the distal pole of the scaphoid.
The extensor pollicis longus tendon is dorsal to the radioscaphoid articulation.
The pronator quadratus muscle extends along the volar surface of the radial metaphysis and distal diaphysis.
The low-signal-intensity tendon of the flexor carpi radialis is draped volarly over the distal pole of the scaphoid.
The long axis (i.e., vertical orientation) of the flexor pollicis longus tendon is seen at the ulnar aspect of the scaphoid.
The capitate, lunate, and radius are colinearly aligned in sagittal images through the third metacarpal axis.
The radial limb of the deltoid or arcuate ligament extends proximally from the volar aspect of the capitate to the scaphoid. In the sagittal plane, the deltoid ligament may appear to connect to the volar distal surface of the lunate.
The radiolunate ligament is located between the volar lunate surface and the distal radius at the radiolunate articulation, deep to the flexor digitorum profundus tendon.
The ulnolunate ligament is radial to the TFC.
The flexor digitorum superficialis and profundus tendons are best seen volar to the capitate and lunate. The flexor retinaculum is a thin hypointense line superficial to the flexor digitorum superficialis. The ulnar limb of the arcuate ligament is seen volar to the radial aspect of the triquetrum and the ulnar aspect of the lunate, ulnar to the plane of section through the capitate.
The fourth metacarpal, the hook of the hamate, and the triquetrum are seen in the same sagittal section at the ulnar-most aspect of the lunate or radial aspect of the ulna. The lunotriquetral interosseous ligament is also seen at this level.
The TFC complex is located between the lunate and the ulna and has a concave distal surface.
On ulnar sagittal images, the flexor carpi ulnaris extends in a volar direction to insert on the pisiform.
The pisohamate and pisometacarpal ligaments attach to the hook of the hamate and the base of the fifth metacarpal, respectively.
The intermediate-signal-intensity ulnar nerve is deep to the flexor carpi ulnaris.
The ulnar collateral ligament component of the TFC complex extends between the triquetrum and ulna, as can be seen on ulnar sagittal images out of the plane of the TFC.
The thick extensor carpi ulnaris tendon is seen as a groove in the posterior aspect of the distal ulna. In peripheral ulnar sagittal sections, it can be seen to extend dorsal to the triquetrum and insert onto the base of the fifth metacarpal.
Imaging Checklist for the Wrist and Hand
The checklist for reviewing an MR examination of the wrist begins in the coronal plane with examination of the following structures:
The intrinsic carpal ligaments, including the scapholunate and lunotriquetral ligaments
The triangular fibrocartilage, including the dorsal and volar margins
The radial and ulnar styloid (for fractures) and the scaphoid and lunate fossa of the distal radius for fractures and cartilage degeneration
The triscaphe articulation (for degenerative changes)
The distal radioulnar articulation, radiocarpal joints, intercarpal joints, and carpometacarpal joints for evidence of arthrosis or posttraumatic change
In the axial plane, the following structures are assessed:
The intrinsic ligaments, with the axial plane allowing assessment of the separate dorsal and volar components of the scapholunate and lunotriquetral ligament
The extensor tendons, including the extensor carpi ulnaris tendon on the ulnar aspect of the wrist, and the extensor pollicis brevis and abductor pollicis longus tendon on the radial aspect of the wrist, for tendinosis, tears, or tenosynovitis
The flexor tendons and the carpal tunnel on the volar aspect of the wrist
The median nerve and ulnar artery
The dorsal and volar capsule and ligaments, for the presence of ganglion cysts or sprain of the capsule
The hook of the hamate, for fracture
The TFC
The distal radioulnar joint, for instability, thenar and hypothenar atrophy (indicative of median neuritis), or strain, and to confirm or characterize fractures
Sagittal plane images are evaluated for the following:
Abnormal carpal alignment suggesting carpal instability
Fractures of the carpal bones, including the hook of the hamate, scaphoid, and lunate
Triangulation on abnormalities of the TFC, scapholunate, and triquetrolunate ligaments
Ganglion cysts
Capsular sprain
Fractures
Coronal Plane Checklist
(1) Scapholunate Ligament (Fig. 10.34) and Lunotriquetral Ligament (Fig. 10.35)
Both the scapholunate and lunotriquetral ligaments are composed of dorsal and volar components comprising the dorsal and volar margins of the ligaments and a membranous component sandwiched between the dorsal and volar components. By viewing successive coronal images dorsal to volar, these separate components of the ligament are visualized and can be examined for tears.
Dorsal images display the transversely oriented ligamentous fibers of the dorsal component of the scapholunate ligament. The dorsal ligament fibers attach firmly to bone on both sides of the scapholunate articulation. The dorsal component is the strongest of the three scapholunate ligament components and is the most important in maintaining carpal stability. The next two or three successive images demonstrate the triangular membranous portion of the scapholunate ligament. A thin band of gray articular cartilage is often interposed between the membranous scapholunate ligament and the underlying scaphoid and lunate articulations and should not be mistaken for a tear.
However, a fluid-filled gap interposed between the membranous scapholunate ligament and the cartilage or bones should be interpreted as a perforation or detachment. Such membranous scapholunate ligament perforations and detachments are more common with advancing age (similar to tears of the TFC central disc), and in isolation may not necessarily result in carpal instability or significant symptoms. The volar-most images demonstrate the volar scapholunate ligament, which courses obliquely and attaches to bone on either side of the ligament. Tears of the volar and radial aspects of the scapholunate ligament suspected in the coronal plane can be confirmed in the axial plane. After identifying a scapholunate ligament tear, the scapholunate interval is assessed for widening, reactive bone marrow changes on either side of the scapholunate articulation, and bony or cartilaginous avulsions at the site of tearing or detachment. In addition, in the setting of scapholunate ligament tears, associated patterns of carpal instability, such as dorsal intercalated segment instability (DISI) pattern, can be identified on corresponding sagittal images.
However, a fluid-filled gap interposed between the membranous scapholunate ligament and the cartilage or bones should be interpreted as a perforation or detachment. Such membranous scapholunate ligament perforations and detachments are more common with advancing age (similar to tears of the TFC central disc), and in isolation may not necessarily result in carpal instability or significant symptoms. The volar-most images demonstrate the volar scapholunate ligament, which courses obliquely and attaches to bone on either side of the ligament. Tears of the volar and radial aspects of the scapholunate ligament suspected in the coronal plane can be confirmed in the axial plane. After identifying a scapholunate ligament tear, the scapholunate interval is assessed for widening, reactive bone marrow changes on either side of the scapholunate articulation, and bony or cartilaginous avulsions at the site of tearing or detachment. In addition, in the setting of scapholunate ligament tears, associated patterns of carpal instability, such as dorsal intercalated segment instability (DISI) pattern, can be identified on corresponding sagittal images.
Similar to the scapholunate ligament, the lunotriquetral ligament also has a dorsal, membranous, and volar component. Compared to the scapholunate ligament, the lunotriquetral ligament may be more difficult to visualize on MR examination. The membranous lunotriquetral ligament is delta-shaped and also often has cartilage interposed between the ligament and bone. The radial and volar components attach directly to bone. Occasionally the only sign of lunotriquetral ligament injury is a subtle step-off in the alignment of the lunate and triquetrum on coronal images. Lunotriquetral ligament tears are not uncommonly seen as part of the spectrum of ulnar abutment syndrome.
(2) Triangular Fibrocartilage (Fig. 10.36)
The TFC is triangular in cross-section, so that the broadest portion of the triangle attaches to the radius and the apex of the triangle converges on the ulnar styloid. In a neutrally positioned wrist, the most dorsal image through the TFC demonstrates the dorsal radial ulnar ligament at the radial attachment. The next two or three successive images in a volar direction demonstrate the central disc and the ulnar attachments of the TFC. The most volar image displays the volar radial ulnar ligament at its radial attachment. The central disc of the TFC is made of fibrocartilage (like the menisci) and appears as an eccentric bowtie on coronal images, wider along the ulnar side, narrower near the radial attachment. When the wrist is imaged in pronation or supination, the orientation of the ulnar styloid is changed with respect to the radius, changing how the TFC appears on coronal slices.
TFC tears can either be degenerative (common in older populations and often asymptomatic) or posttraumatic (more often clinically significant and more commonly seen in younger patients). Degenerative tears and perforations most commonly are seen just proximal to the radial attachment of the TFC. According to the Palmer classification, posttraumatic tears can occur in the central portion of the TFC proximal to the radius or, less commonly, at the radial attachment or ulnar styloid attachment. The central disc attachment to the radius may attach to hyaline articular cartilage, and it is important not to mistake the gray cartilage signal at the attachment for a tear, which is usually of fluid signal intensity. The ulnar side of the TFC usually attaches to the ulna via two ligamentous fascicles, one to the base of the ulnar styloid and the other to the tip. These ulnar-sided attachments are less frequently torn. The ulnar-sided attachments, however, can be disrupted with ulnar styloid fractures that occur at the base of the ulnar styloid, leading to instability. Therefore, it is important to distinguish ulnar styloid tip fractures from fractures that occur at the base. Tears of the central disc are most easily seen on coronal images.
(3) Distal Radius and Ulna (Fig. 10.37)
Fractures of the distal radius and ulna are evaluated on coronal images. In particular, extension of radial fractures into the articular surface of the radius are characterized. The distal radius articular surfaces are divided into two concave cartilage-covered surfaces known as the lunate fossa (which is medial and articulates with the lunate) and the scaphoid fossa (which is lateral and articulates with the scaphoid). Fractures through the lunate fossa can further be characterized on sagittal images as extending through the dorsal or palmar medial
aspect of the lunate fossa. The distal lateral tip of the radius is known as the radial styloid. Fractures through the radial styloid may extend into the scaphoid fossa. Fractures through the lunate and scaphoid fossae can lead to subsequent radiocarpal degenerative arthrosis. Fractures of the ulnar styloid are characterized as occurring either at the distal ulnar styloid or at the base of the ulnar styloid. Fractures that occur at the base of the ulnar styloid can destabilize the ulnar attachments of the TFC, leading to subsequent distal radial ulnar joint instability.
aspect of the lunate fossa. The distal lateral tip of the radius is known as the radial styloid. Fractures through the radial styloid may extend into the scaphoid fossa. Fractures through the lunate and scaphoid fossae can lead to subsequent radiocarpal degenerative arthrosis. Fractures of the ulnar styloid are characterized as occurring either at the distal ulnar styloid or at the base of the ulnar styloid. Fractures that occur at the base of the ulnar styloid can destabilize the ulnar attachments of the TFC, leading to subsequent distal radial ulnar joint instability.
(4) Radiocarpal Joints (Fig. 10.38)
The cartilage surfaces and subchondral bone of the distal radius and the proximal carpal row are evaluated in the coronal plane. Radioscaphoid arthrosis is the most common site of degenerative arthrosis in the wrist. In its earliest stages, radioscaphoid arthrosis begins at the distal radial styloid-scaphoid articulation (stage I scapholunate advanced collapse [SLAC]) and progresses to involve the entire radioscaphoid articulation (stage IIA SLAC). Subchondral edema and sclerosis and overlying cartilage fissuring, fibrillation, or full-thickness erosion is also characterized on coronal images.
Ulnocarpal (ulnolunate) abutment syndrome is most commonly visualized as chondromalacia and subchondral edema or sclerosis at the proximal ulnar aspect of the lunate, opposite the TFC, and is classically associated with TFC tears, lunotriquetral ligament tears, and ulnar positive variance. Ulnar positive variance, however, is not required for the diagnosis. Also, proximal triquetral chondromalacia and subchondral changes can be seen with ulnar styloid impaction (a distinct entity from ulnocarpal abutment syndrome), in which a prominently enlarged
ulnar styloid can chronically impact the proximal triquetrum.
ulnar styloid can chronically impact the proximal triquetrum.
(5) Intercarpal Joints including the Triscaphe Joint (Fig. 10.39)
The triscaphe joint is examined in the coronal plane. The triscaphe joint consists of the distal pole of the scaphoid articulating with the trapezoid and trapezium and is considered the second most common site of wrist arthrosis. Triscaphe arthrosis is commonly seen concurrently with radioscaphoid arthrosis. Other intercarpal articulations examined in the coronal plane for arthrosis include the scaphocapitate, hamate-lunate, and trapeziotrapezoidal articulations. Particular attention should be paid to the hamate-lunate articulation in the presence of a medial lunate facet, as in one cadaveric study 44% of specimens with medial lunate facets demonstrated arthrosis at this articulation.
(6) Carpometacarpal Joints (Fig. 10.40)
The carpometacarpal articulations are examined for the presence of arthrosis, which most commonly occurs at the first carpal-metacarpal joint. A prominent protuberance (or ossicle) at the base of the dorsal third or second metacarpal (called a carpal boss) can also be identified in some patients on coronal images, and evidence of carpal boss impingement (bone marrow edema, degenerative arthrosis, adjacent ganglion cysts) may also be identified. An ossicle at the base of the dorsal third or second metacarpal is called an os styloideus and also can be associated with degenerative changes at the carpometacarpal joint.
(7) Distal Radioulnar Joint (Fig. 10.41)
The articular surface of the distal ulna articulates with the sigmoid notch of the distal radius. The coronal plane is optimal for evaluating arthrosis (as evidenced by spurring, reactive edema, or erosions at the joint), as well as associated distal radioulnar joint effusions and synovitis. The ulnar impingement syndrome, in which there is a short ulna that impacts and erodes the ulnar aspect of the radius, is also well displayed on coronal plane images. This is a distinct entity from the ulnocarpal (ulnolunate) abutment syndrome, which involves ulnar-carpal impaction. In addition, ulnar minus or positive variance is best assessed in the coronal plane, although pronation and supination can change the alignment of the ulnar articular surface with the radius, leading to false-positive diagnoses. Such ulnar variance can change the normal balance of load transfer across the wrist (normally 82% radial and 18% ulnar). Ulnar positive variance is thought to be associated with ulnocarpal
(ulnolunate) impaction syndrome, whereas ulnar negative variance may be associated with Kienböck’s disease of the lunate. With distal radioulnar joint instability, the ulna is dorsally or volarly subluxed with respect to the radius, usually due to severe TFC complex tears.
(ulnolunate) impaction syndrome, whereas ulnar negative variance may be associated with Kienböck’s disease of the lunate. With distal radioulnar joint instability, the ulna is dorsally or volarly subluxed with respect to the radius, usually due to severe TFC complex tears.
(8) Osseous Integrity and Fractures (Fig. 10.42)
Other osseous pathology affecting the distal radius and ulna and carpus, such as fractures, tumors, and AVN, are also evaluated in the coronal plane. Kienböck’s disease of the lunate is associated with ulnar negative variance. AVN commonly affects the proximal scaphoid as a result of fracture, or less commonly in the absence of fracture (Preiser’s disease).
Axial Plane Checklist
(1) Scapholunate and Lunotriquetral Ligaments (Fig. 10.43)
Axial images through the scapholunate and lunotriquetral ligaments may also aid in further characterizing the extent of tears through the dorsal, membranous, and/or volar aspects of the ligament.
(2) Extensor Tendons (Fig. 10.44)
There are six extensor compartments containing the dorsal extensor tendons. In the axial plane these tendons are displayed in cross-section, which makes axial plane imaging ideal for the demonstration of tendinosis, tears, and tenosynovitis (fluid and synovitis in the tendon sheath):
Compartment 1 lies along the lateral aspect of the radius and contains the extensor pollicis brevis and the abductor pollicis longus.
Compartment 2 contains the extensor carpi radialis brevis and longus.
Compartment 3 contains the extensor pollicis longus.
Compartment 4 contains the extensor digitorum tendons.
Compartment 5 contains the extensor digiti minimi.
Compartment 6 contains the extensor carpi ulnaris, which runs within the groove formed by the ulnar styloid.
The most common compartments to be associated with abnormalities are compartments 1, 2, 3, and 6. Tenosynovitis and tendinosis of the first extensor compartment, known as de Quervain’s disease, usually occurs secondary to chronic repetitive motion injury. Tenosynovitis of the second extensor compartment (called intersection syndrome) occurs along the dorsal
radial aspect of the distal forearm (proximal to the wrist), where the first compartment muscles cross over the second extensor compartment tendons. Tendinosis and tearing of the extensor carpi ulnaris (in compartment 6) is also common and presents as dorsal ulnar-sided pain. The extensor pollicis longus (in compartment 3) can also occasionally tear. The distally retracted, thickened, and frayed end of the torn extensor pollicis longus is often visualized at the level of the proximal carpal row, where the extensor pollicis longus tendon crosses dorsal to the extensor carpi radialis brevis and longus tendons.
radial aspect of the distal forearm (proximal to the wrist), where the first compartment muscles cross over the second extensor compartment tendons. Tendinosis and tearing of the extensor carpi ulnaris (in compartment 6) is also common and presents as dorsal ulnar-sided pain. The extensor pollicis longus (in compartment 3) can also occasionally tear. The distally retracted, thickened, and frayed end of the torn extensor pollicis longus is often visualized at the level of the proximal carpal row, where the extensor pollicis longus tendon crosses dorsal to the extensor carpi radialis brevis and longus tendons.
(3) Flexor Tendons (Fig. 10.45)
The flexor tendons are also evaluated on axial images for the presence of tenosynovitis, tendinosis, or tearing. On the ulnar aspect of the wrist, the flexor carpi ulnaris runs outside the flexor retinaculum and is separate from the carpal tunnel. The flexor digitorum superficialis and profundus tendons lie within the flexor retinaculum. The flexor pollicis longus tendon is the most radially located tendon within the carpal tunnel. The flexor carpi radialis tendon lies radial and volar to the carpal tunnel in its own fibroosseous tunnel and commonly demonstrates tenosynovitis at the level where the tendon abuts the medial aspect of the hook of the trapezium, a common area of tendon impingement.
(4) Median Nerve (Carpal Tunnel) and Ulnar Nerve (Guyon’s Canal) (Fig. 10.46)
The median nerve is imaged in cross-section on axial images and normally appears intermediate in signal, with an oval shape at the distal radius and an elliptical shape at the pisiform. The axial plane is well suited to assessment of median neuritis, including signs such as swelling and enlargement at the level of the pisiform and flattening at the level of the hamate. Secondary signs of median neuritis seen on axial images include palmar bowing of the flexor retinaculum and increased signal in the thenar muscles due to denervation. Although most often median neuritis is idiopathic, underlying causes are occasionally demonstrated, such as median nerve tumors, trauma, ganglion cysts, or tenosynovitis of the flexor tendon sheaths.
The ulnar nerve is also imaged in cross-section on axial images. The ulnar nerve is located in the volar medial aspect of the wrist within Guyon’s canal, which runs just palmar and lateral to the pisiform and hook of the hamate. Ulnar neuritis from pathology in Guyon’s canal is much less common than median neuritis.
(5) Carpus (Including the Hook of Hamate) (Fig. 10.47)
Successive axial images of the carpal bones allow detection and characterization of fractures, contusions, and other osseous
abnormalities. In particular, close attention should be paid to the hook of the hamate in the axial plane. Pisotriquetral arthrosis and synovitis are also evaluated on axial images.
abnormalities. In particular, close attention should be paid to the hook of the hamate in the axial plane. Pisotriquetral arthrosis and synovitis are also evaluated on axial images.
(6) Ganglion Cysts and Capsular Ligaments (Fig. 10.48)
Ganglion cysts are common, and although they are best detected on axial images, the sagittal and coronal planes are used for confirmation and further localization. On successive images through the wrist (preferably FS PD FSE images), the dorsal and volar capsular and ligamentous surfaces should be carefully inspected for the presence of fluid-signal ganglion cysts. Ganglions can be as small as 2 to 3 mm or can reach up to several centimeters in size. Ganglion cysts may protrude from nearly any wrist articulation, often with extension of a funnel-shaped neck back toward the ligament of origin. Common sites of origin are the dorsal scapholunate ligament (where even the smallest ganglions can cause symptoms by impinging the dorsal interosseous nerve), the volar radiocarpal joint, and the triscaphe joint. Volar ganglion cysts may compress the carpal tunnel, where they can contribute to median neuritis. Ganglion cysts are distinguished from free fluid in the joint space deep to the dorsal wrist capsule by looking for evidence of fluid loculation in a ganglion cyst, usually evidenced by a multilobulated, septated, and more localized appearance. In addition to causing symptoms, ganglion cysts may also be a clue to tears or perforations in the underlying ligaments from which they arise, presumably from fluid extending through a ligament perforation via a one-way valve mechanism.
The axial plane is also used in the assessment of synovitis and sprain or tears of the dorsal and volar capsular ligaments.
(7) Distal Radial Ulnar Joint (Fig. 10.49)
The distal radioulnar joint is examined in the axial plane to view the alignment of the radius with respect to the ulna. The ulna should lie within the concave groove in the medial aspect of the radius called the sigmoid notch, and the two bones lie grossly in the same plane. Mild apparent dorsal shift of the ulna with respect to the radius is normal when the wrist is scanned in full pronation, and mild volar shift of the ulna is normal in full supination. Studies show that the radius is actually moving with respect to a stationary ulna. The position of the ulnar styloid in the axial plane is used to assess the position of the wrist:
In a neutral position the ulnar styloid is located medially.
In pronation the ulnar styloid points volarly.
In supination the ulnar styloid points dorsally.
One easy way to remember this rule is that in supination, the ulnar styloid points dorsally, with the ulnar styloid groove also pointing dorsally, resembling a bowl of soup (hence, supination).
The dorsal radioulnar ligament and palmar radioulnar ligament are the primary ligamentous stabilizers of the distal radioulnar joint and on axial plane images are seen coursing on both the dorsal and volar sides of the TFC, at the level of the base of the ulnar styloid, where the ligaments insert. Tears of the dorsal radioulnar ligament are associated with volar subluxation of the ulna. Tears of the volar radioulnar ligament are associated with dorsal subluxation of the ulna. Distal radioulnar joint instability is suggested when the ulnar head is abnormally subluxed or dislocated with respect to the radius, beyond the normal range of motion allowed for pronation and supination. In addition to ligamentous injury, osseous injuries such as fractures at the base of the ulnar styloid also may lead to distal radioulnar joint instability.
The dorsal radioulnar ligament and palmar radioulnar ligament are the primary ligamentous stabilizers of the distal radioulnar joint and on axial plane images are seen coursing on both the dorsal and volar sides of the TFC, at the level of the base of the ulnar styloid, where the ligaments insert. Tears of the dorsal radioulnar ligament are associated with volar subluxation of the ulna. Tears of the volar radioulnar ligament are associated with dorsal subluxation of the ulna. Distal radioulnar joint instability is suggested when the ulnar head is abnormally subluxed or dislocated with respect to the radius, beyond the normal range of motion allowed for pronation and supination. In addition to ligamentous injury, osseous injuries such as fractures at the base of the ulnar styloid also may lead to distal radioulnar joint instability.
Sagittal Plane Checklist
(1) Hook of Hamate, Scaphoid, and Lunate (Fig. 10.50)
The length of the hook of the hamate is also visualized in the sagittal plane, and fractures of the hook of the hamate are also evaluated in the sagittal plane. Similarly, fractures and AVN of the scaphoid and lunate are also further characterized on sagittal images.
(2) Carpal Alignment (Fig. 10.51)
The alignment of the lunate and capitate is evaluated on sagittal images. DISI is suggested when the capitate lunate angle
exceeds 30°. When DISI is present, the scapholunate ligament is evaluated for associated tears. The position of the scaphoid with respect to the rest of the carpus is also assessed. An anteriorly tipped or “flexed” scaphoid is an additional sign of DISI. When the lunate is tipped in a volar direction, with palmar translocation of the carpus, volar intercalated segmental instability (VISI) is suggested. VISI is associated with lunotriquetral ligament tears and dorsal extrinsic ligament injuries.
exceeds 30°. When DISI is present, the scapholunate ligament is evaluated for associated tears. The position of the scaphoid with respect to the rest of the carpus is also assessed. An anteriorly tipped or “flexed” scaphoid is an additional sign of DISI. When the lunate is tipped in a volar direction, with palmar translocation of the carpus, volar intercalated segmental instability (VISI) is suggested. VISI is associated with lunotriquetral ligament tears and dorsal extrinsic ligament injuries.
(3) Ganglion Cysts and Capsular Ligaments (Fig. 10.52)
Ganglion cysts suspected on axial images are confirmed and further characterized in the sagittal plane. In addition, strain or tears of the dorsal and volar extrinsic capsular ligaments can be demonstrated.
(4) Flexor and Extensor Tendons (Fig. 10.53)
The flexor and extensor tendons and the median nerve are seen in their long axis on sagittal images. Many of the tendons are seen along their entire course through the FOV on only one or two sagittal images. However, tendons are sometimes incompletely imaged in the sagittal plane due to slice gaps.
(5) Triangular Fibrocartilage, Scapholunate, and Lunotriquetral Ligaments (Fig. 10.54)
On sagittal images, the ulnar aspect of the TFC is the apex of the triangle and appears as a relatively thin band of fibers. On successive images toward the radius, the TFC fans out in a dorsal to volar direction. Near the radius, the normal TFC appears bowtie-shaped,
similar to the meniscus. Tears of the TFC are visualized as defects or gaps in the substance of the TFC. Tears of the membranous portion of the TFC manifest as a gap with a diastasis between the two ends of the bow-tie. The scapholunate and lunotriquetral ligaments are harder to visualize on sagittal images. However, tears of the dorsal or volar components of these ligaments, or ganglion cysts extending through these ligaments, are occasionally seen and further characterized on sagittal images.
similar to the meniscus. Tears of the TFC are visualized as defects or gaps in the substance of the TFC. Tears of the membranous portion of the TFC manifest as a gap with a diastasis between the two ends of the bow-tie. The scapholunate and lunotriquetral ligaments are harder to visualize on sagittal images. However, tears of the dorsal or volar components of these ligaments, or ganglion cysts extending through these ligaments, are occasionally seen and further characterized on sagittal images.
Sample MRI Report, Wrist Injury
Clinical Information: Wrist pain, evaluate for TFC complex tear
Technique: Coronal and axial T1-weighted and FS PD FSE images and sagittal FS PD FSE images
Findings: Dissociation of the scapholunate interval is demonstrated with a diastasis of more than 4 mm (Fig. 10.55A). This is associated with disruption of all components of the scapholunate ligament (Fig. 10.55B). Superimposed mild proximal migration of the capitate with arthrosis between the lunate and capitate (Fig. 10.55C) is visualized on coronal images. There is cystic change within the distal pole of the capitate (Fig. 10.55C). Mild sclerosis of the radial styloid scapho-lunate articulation is consistent with a component of SLAC arthritis (Fig. 10.55D).
Degenerative change of the triangular fibrocartilage is shown with fraying of both proximal and distal surfaces of its radial aspect (Fig. 10.55E). Mild dorsal capsular synovitis (Fig. 10.55F) is seen. Small cystic fluid collections consistent with small ganglions communicating with the pisiform triquetral joint are seen (Fig. 10.55G). There is mild tenosynovitis of the second extensor compartment and mild tenosynovitis of the flexor carpi radialis (Fig. 10.55H).
A DISI pattern exists with dorsal tilting of the capitate on sagittal images (Fig. 10.55I).
Impression:
Scapholunate ligament diastasis with greater than 4 mm of diastasis of the scapholunate ligament. Superim-posed arthrosis of the capitolunate articulation.
Mild sclerosis of the radial styloid scapholunate articulation consistent with a component of SLAC arthritis.
Fraying of the proximal and distal aspects of the triangular fibrocartilage.
Mild degenerative change in the lunotriquetral ligament.
Synovitis and ganglion cyst in communication with the pisiform triquetral articulation.
DISI instability of the wrist with dorsal tilting of the lunate.
Functional Anatomy of the Wrist and Hand
Osseous Structures
The osseous elements of the wrist consist of the distal radius and ulna, the proximal and distal carpal rows, and the bases of
the metacarpals. There are three major compartments of the wrist as defined by arthrographic studies:
the metacarpals. There are three major compartments of the wrist as defined by arthrographic studies:
The radiocarpal compartment
The midcarpal compartment
The distal radioulnar joint compartment
Distal Radioulnar Joint
On its medial side, the distal radius forms a shallow depression for articulation with the ulnar head (Fig. 10.56). The sigmoid notch acts as a seat for the rotating pole of the distal ulna and provides some bony stability to the distal radioulnar joint. The distal radioulnar joint is inclined 20° distally and ulnarly, and this angle of inclination is thought to be important in maintaining forearm rotation. The stabilizing ligaments for the joint include the TFC and the dorsal and volar capsular ligaments (Fig. 10.57). These capsular ligaments are poorly defined and cannot be visualized as distinct anatomic structures. The TFC connects the ulna and radius at their most distal edges and separates the distal radioulnar joint from the radiocarpal joint. The TFC runs from the ulnar-most edge of the lunate facet and sigmoid notch to the base of the ulnar styloid, where it inserts into a small depression in the distal ulna known as the fovea. The ulnar insertion consists of two limbs: one distal and one proximal. However, traumatic loss of the soft-tissue stabilizers of the distal radioulnar joint, primarily the TFC complex, may cause subluxation of the radius on the fixed unit of the ulna.35 The distal radioulnar joint and synovial cavity are identified between the distal radius and ulna and extend across the distal ulna deep to the TFC.36
Pearls and Pitfalls
Anatomy of the Wrist
The carpal bones absorb stress from the palm to the radius, change geometric shape in response to motion, and form a proximal row intercalary segment.
The extrinsic ligaments, which connect the radius or ulna to the carpal bones or the metacarpal to the carpal bones, provide gross stability.
The intrinsic ligaments include the intercarpal ligaments, which provide intermediate stability, and the interosseous ligaments, which provide for “fine-tune” stability.
Radiocarpal Joint
The radiocarpal joint is defined by the TFC and the distal radial surface proximally and the lunate, triquetrum, and scaphoid distally.
At the site of the radiolunate articulation, the distal articular surfaces of the radius and ulna are usually at the same level (i.e., neutral ulnar variance). Alternatively, the ulna may be relatively long (positive ulnar variance), leading to an ulnar abutment syndrome, or relatively short (negative ulnar variance), as is often seen in Kienböck’s disease. The distal radius forms two facets that articulate with the scaphoid and lunate of the proximal carpal row. This articulation of the proximal pole of the scaphoid in the scaphoid fossa is quite congruent, and even a small degree of malrotation of the scaphoid may cause incongruent loading of the articular cartilage and subsequent degeneration (such as that which accompanies a SLAC wrist, as described by Watson and Ryu37). The lunate facet commonly becomes incongruent following distal radius fractures, especially die-punch-type fractures. The interosseous ligaments join the proximal carpal bones at their proximal edges.36
At the site of the radiolunate articulation, the distal articular surfaces of the radius and ulna are usually at the same level (i.e., neutral ulnar variance). Alternatively, the ulna may be relatively long (positive ulnar variance), leading to an ulnar abutment syndrome, or relatively short (negative ulnar variance), as is often seen in Kienböck’s disease. The distal radius forms two facets that articulate with the scaphoid and lunate of the proximal carpal row. This articulation of the proximal pole of the scaphoid in the scaphoid fossa is quite congruent, and even a small degree of malrotation of the scaphoid may cause incongruent loading of the articular cartilage and subsequent degeneration (such as that which accompanies a SLAC wrist, as described by Watson and Ryu37). The lunate facet commonly becomes incongruent following distal radius fractures, especially die-punch-type fractures. The interosseous ligaments join the proximal carpal bones at their proximal edges.36
![]() FIGURE 10.57 ● The articular surface of the distal end of the radius and the adjacent triangular cartilage are exposed by removal of the carpal bones. |
![]() FIGURE 10.58 ● Lister’s tubercle (dorsal tubercle) of the distal radius on a coronal PD FSE image. Lister’s tubercle functions as a pulley for the extensor pollicis longus. |
Lister’s tubercle, the most prominent dorsal radial ridge, separates the extensor pollicis longus tendon (ulnar side) from the extensor carpi brevis tendon (radial side) (Fig. 10.58). This is the site of formation of bone spurs and attrition ruptures in rheumatoid arthritis.
Carpus and Midcarpal Joint
The proximal carpal row consists of the scaphoid, the lunate, and the triquetrum. It is thought that with a congenital bipartite scaphoid, the proximal row should include only the proximal pole of the scaphoid. The distal pole should be thought of as a component of the distal row. The scaphoid, lunate, and triquetrum are linked by strong interosseous ligaments that work together to form a flexible socket or acetabulum that cradles the distal row. Occasionally, anatomic imperfections in this socket lead to arthritic degeneration. The lunate may have a medial facet, which measures 1 to 6 mm in diameter. This facet is present in approximately two thirds of cadaver hands studied, and 44% of these had arthritic degeneration in the proximal pole of the hamate.38 Hamate arthritis is not seen unless the medial facet was present on the lunate.38 The distal carpal row consists of the trapezium, the trapezius, the capitate, and the hamate (Figs. 10.59 and 10.60).
The midcarpal joint is formed between the proximal and distal carpal rows (see Fig. 10.60). The midcarpal joint cavity is located primarily between the distal aspects of the scaphoid, lunate, and triquetrum and the proximal aspect of the distal row. Proximal extension of the midcarpal joint between the scaphoid and lunate and between the lunate and triquetrum is limited by the interosseous ligaments.36 Three distal extensions of the midcarpal joint are located between the four bones of the distal carpal row. The trapezium-trapezoid or trapezoid and capitate joint spaces may communicate with the second and third carpometacarpal joints. The first carpometacarpal joint does not communicate with the midcarpal joint. The separate joint space between the hamate and the fourth and fifth metacarpals may communicate with the midcarpal joint.
Ligamentous Anatomy
Much of the interest in and appreciation of the ligamentous anatomy of the wrist derives from the advent of wrist arthroscopy. Arthroscopy allows direct examination of the ligaments of the wrist and testing of their physiologic integrity.
All arthroscopic portals are, of necessity, dorsally placed, making examination of the volar ligaments especially easy. As a result, the dorsal ligaments initially received less attention. Definition of pathologic conditions naturally followed elucidation of the ligamentous anatomy, resulting in the development of a variety of treatment procedures.39
All arthroscopic portals are, of necessity, dorsally placed, making examination of the volar ligaments especially easy. As a result, the dorsal ligaments initially received less attention. Definition of pathologic conditions naturally followed elucidation of the ligamentous anatomy, resulting in the development of a variety of treatment procedures.39
![]() FIGURE 10.59 ● The bones of the hand. Adjacent bones, particularly in the carpus, have been slightly separated to reveal their articular surfaces. |
The ligaments of the wrist are classified into intrinsic and extrinsic groups (Table 10.1).40 The extrinsic ligaments extend from the radius, ulna, and metacarpals; the intrinsic ligaments originate and insert within the carpus. In general, the role of the intrinsic ligaments is to maintain the relationships among the individual carpal bones, whereas the extrinsic ligaments are important in the relationship of the carpus as a whole to the distal radius and ulna, as well as the bases of the metacarpals. The extrinsic ligaments, as well as the intrinsic ligaments, are crucial in maintaining the intercarpal relationships. Many investigators object to this classification scheme because it is possible for imbalances of the intrinsic ligaments to cause carpal instability, a condition that was formerly thought to occur only through dysfunction of the intrinsic ligaments. However, this scheme remains useful, if only as an anatomic guide.
Extrinsic Ligaments
Radiocarpal Ligaments
The volar extrinsic ligaments (Fig. 10.61) are the most constant and the strongest of the extrinsic ligaments. Several mechanically important ligaments originate from the region of the radial styloid and distal radius, including the radial collateral and palmar radiocarpal ligaments. The latter consists of the radioscaphocapitate ligament, the radiolunotriquetral ligament (also sometimes referred to as the long radiolunate ligament), the radioscapholunate ligament, and the short radiolunate ligament.41
By virtue of their orientation and mechanical properties, the radiocarpal ligaments maintain the carpus within its radial articulation. Loss of these ligaments allows the carpus to move down the inclined plane of the distal radius and undergo ulnar translation. This condition is not uncommon in rheumatoid arthritis, in which synovitic degeneration of these soft-tissue supporting structures occurs. With ulnar translation, the distance between the radial styloid and the scaphoid increases and the scaphoid and lunate are displaced from their articular fossae. The lunate comes to rest where it articulates with the distal ulna, and the scaphoid becomes perched on the ridge between its own articulation and the lunate fossa. This incongruent loading leads to degeneration of the cartilaginous surfaces and ulnolunate impingement. Overexuberant surgical resection of the radial styloid can destroy the origin of these ligaments and may cause this type of instability. Distal ulna resection (the Darrach procedure) in the rheumatoid wrist may also lead to ulnar translation of the carpus with the loss of the ulnar buttress.
Radial Collateral Ligament
Although the radial collateral ligament is not a true collateral ligament (because ulnar and radial deviation are normal motion arcs in the wrist, and, by definition, a collateral ligament resists only pathologic or abnormal motion), it has been shown to be mechanically significant and to play a role in the mechanism of midwaist scaphoid fractures by compressing the bone along its longitudinal axis.42 This ligament originates on the tip of the styloid and inserts onto the radial aspect of the scaphoid at its waist. Fibers of the radial collateral ligament also extend from the scaphoid to the trapezium, blending with the transverse carpal ligament and dorsal capsular ligament.36
Radioscaphocapitate Ligament
The radioscaphocapitate ligament is a very stout ligament, readily identified through the arthroscope. It originates from the radial styloid, has a minor insertion into the radial aspect of the waist of the
scaphoid, and also inserts on the center of the capitate.43 The radioscaphocapitate ligament forms a supporting sling at the waist of the scaphoid. As the fibers cross the proximal pole of the scaphoid, there is a fold of synovium that separates them from the bone.44 In this position, the ligament can be interposed between the fragments of a scaphoid fracture and contribute to nonunion. The radioscaphocapitate ligament, which has a striated appearance on volar coronal MR images, is located distal to the radiolunotriquetral ligament, which has a similar ulnodistal obliquity (Fig. 10.62). Sagittal images demonstrate the volar location of the radioscaphocapitate in cross-section relative to the waist of the scaphoid.
scaphoid, and also inserts on the center of the capitate.43 The radioscaphocapitate ligament forms a supporting sling at the waist of the scaphoid. As the fibers cross the proximal pole of the scaphoid, there is a fold of synovium that separates them from the bone.44 In this position, the ligament can be interposed between the fragments of a scaphoid fracture and contribute to nonunion. The radioscaphocapitate ligament, which has a striated appearance on volar coronal MR images, is located distal to the radiolunotriquetral ligament, which has a similar ulnodistal obliquity (Fig. 10.62). Sagittal images demonstrate the volar location of the radioscaphocapitate in cross-section relative to the waist of the scaphoid.
TABLE 10.1 Extrinsic and Intrinsic Ligaments of the Wrist | ||||||||||||||||||||||||||||||||||||||||||||||||||||||
---|---|---|---|---|---|---|---|---|---|---|---|---|---|---|---|---|---|---|---|---|---|---|---|---|---|---|---|---|---|---|---|---|---|---|---|---|---|---|---|---|---|---|---|---|---|---|---|---|---|---|---|---|---|---|
|
Radiolunotriquetral Ligament or Long Radiolu-nate Ligament
Progressing ulnarly, the radiolunotriquetral ligament (also referred to as the long radiolunate ligament) is the next ligament seen. It is the largest ligament of the wrist43 (Fig. 10.63) and originates ulnar to the radioscaphocapitate from the volar lip of the radial styloid process. The radiolunotriquetral ligament has an oblique course attached to the volar aspects of the lunate and triquetrum (Fig. 10.64). On volar coronal MR images it displays a striated band-like appearance,
similar to the radioscaphocapitate ligament. There is an interligamentous sulcus between the radioscaphocapitate and the radiolunotriquetral ligaments on sagittal images (Fig. 10.65). The radiolunotriquetral is a strong ligament that stabilizes the proximal carpal row on the radius and should be differentiated from the radioscapholunate ligament.
similar to the radioscaphocapitate ligament. There is an interligamentous sulcus between the radioscaphocapitate and the radiolunotriquetral ligaments on sagittal images (Fig. 10.65). The radiolunotriquetral is a strong ligament that stabilizes the proximal carpal row on the radius and should be differentiated from the radioscapholunate ligament.
Short Radiolunate Ligament
The short radiolunate ligament has been described by Berger and Landsmeer.44 This ligament originates from the radius in the region of the lunate facet and inserts distally onto the volar surface of the lunate (Fig. 10.66). At its insertion, its most radial fibers merge with those of the long radiolunate ligament. It acts as a volar tether to the lunate and plays a major role in preventing the development of DISI deformities with lunate extension.
Radioscapholunate Ligament
The radioscapholunate ligament is interposed dorsally between the long radiolunate and the short radiolunate ligaments. It arises at the level of the interfacet prominence of the distal radius and inserts onto the scapholunate articulation (Fig. 10.67).43 This structure was first described in detail by Testut and is often called by his name (i.e., the ligament of Testut or the ligament of Testut or Kuenz).45 The radioscapholunate ligament has been studied extensively, and it has been shown to contain the most elastic tissue of any ligament in the wrist. It does not appear to provide any mechanical support to the carpus.
From his work on fetal wrists, Landsmeer described a vascular pedicle that supplies the radioscapholunate ligament.46 It has since been shown that this structure is a neurovascular umbilical cord that may provide a clinically significant blood supply to the proximal pole of the scaphoid via the scapholunate interosseous ligament and a sensory or proprioceptive pathway to the scapholunate joint.47 The radioscapholunate ligament receives its neurovascular supply from the anterior interosseous artery and nerve.
On volar coronal MR arthrographic images, the radioscapholunate ligament can be seen as a short ligament with
a straight course or minimally convex radial border directed toward the scapholunate interval. The radioscapholunate ligament does not have the striations previously described for the radioscaphocapitate and radiolunotriquetral ligaments on coronal images. The proximal attachment of the radioscapho-lunate ligament should never be confused with the normal articular cartilage ridge that separates the scaphoid and lunate fossa of the distal radius. This articular cartilage ridge has a broad-based attachment to the distal radius.
a straight course or minimally convex radial border directed toward the scapholunate interval. The radioscapholunate ligament does not have the striations previously described for the radioscaphocapitate and radiolunotriquetral ligaments on coronal images. The proximal attachment of the radioscapho-lunate ligament should never be confused with the normal articular cartilage ridge that separates the scaphoid and lunate fossa of the distal radius. This articular cartilage ridge has a broad-based attachment to the distal radius.
Ulnocarpal Ligaments
The ulnar portion of the extrinsic volar ligaments of the wrist is formed by the TFC complex (Figs. 10.68 and 10.69). The TFC complex consists of the TFC (the articular disc, the dorsal and volar radioulnar ligaments), the meniscus homologue, and the ulnolunate and the ulnotriquetral ligaments (Fig. 10.70). The term TFC complex was coined to describe all of the ligamentous and cartilaginous structures that were thought to play a role in suspending the distal radius and the ulnar carpus from the distal ulna,48 including the subsheath of the extensor carpi ulnaris tendon. Clinical and laboratory data support the role of the TFC complex in maintaining both the stability of the distal radioulnar joint and the stability of the carpus as a whole (preventing pronosupination of the carpus). The TFC complex also contributes to stability within the carpus by preventing nondissociative carpal instabilities (see discussion below on carpal instabilities).
Dorsal Ligaments
Although the palmar or volar radiocarpal ligaments have attracted a great deal of attention in the past, the biomechanics of the wrist also relies on the dorsal ligaments.
The dorsal ligaments do not exist as discrete anatomic entities, and they vary considerably from subject to subject. Two major components can be discerned:
The dorsal ligaments do not exist as discrete anatomic entities, and they vary considerably from subject to subject. Two major components can be discerned:
The first component is the dorsal radioscapholunotriquetral ligament, a thickening of the dorsal capsule that courses from the dorsal lip of the radius and inserts on the dorsal surfaces of the scaphoid, lunate, and triquetrum. This ligament acts as a checkrein on the proximal carpal row and prevents it from assuming a position of excessive volarflexion. Biomechanical studies have shown that in the final stage of ulnar-sided perilunate instability, it is the radioscapholunotriquetral ligament that is injured.49 Laxity of the radioscapholunotriquetral ligament has been implicated in palmar midcarpal instability patterns in which the lunate is allowed to go into volarflexion, leading to instability.
The second major component of the dorsal ligamentous structure is the scaphotriquetral (or triquetroscaphoid) ligament. This is a transversely oriented thickening of the dorsal capsular fibers that runs from the scaphoid to the triquetrum.
Using multiplanar reconstructions with 3DFT MR imaging, Smith50,51 has demonstrated and described the dorsal carpal ligaments of the wrist. The radiotriquetral ligament can be seen to consist of a single band that arises from the distal radius, adjacent to Lister’s tubercle. The dorsal intercarpal ligament is seen either as a broad fused band (a branched structure) with separate triquetroscaphoid and triquetrotrapezoid fascicles, or as completely separate triquetroscaphoid and triquetrotrapezoid fascicles (Figs. 10.71 and 10.72).
Intrinsic Interosseous Ligaments
Scapholunate and Lunotriquetral Ligaments
The interosseous ligaments are of paramount importance in maintaining the biomechanical relationship among the carpal bones, especially those of the proximal row (Fig. 10.73). For the proximal carpal row to function properly, the bones must be associated or linked together, and the interosseous ligaments provide this flexible linkage. The scapholunate and lunotriquetral ligaments are comparable in strength with the anterior cruciate ligament of the knee. They connect the bones at the level of the proximal articular surface and consist of thick dorsal and volar components with thinner membranous portions in between. Most commonly, perforations occur in the thin, membranous portions and may not be mechanically significant. Specific characteristics of the scapholunate and lunotriquetral ligaments include:
The scapholunate ligament is triangular on coronal section and is peripherally attached at the scapholunate interval. The inner apex of the triangular ligament is not attached to bone and is free within the scapholunate joint (Fig. 10.74).52
The dorsal fibers of the scapholunate ligament are oriented transversely, or perpendicular to the joint, and form a thick bundle. The dorsal portion of the scapho-lunate ligament is considered to be the most important component in maintaining carpal stability.
The membranous scapholunate ligament fibers course peripherally and obliquely from the scaphoid downward to the lunate. The membranous scapholunate ligament fibers attach to both bone and articular cartilage, whereas the dorsal and volar portions of the scapholunate ligament attach directly to bone.
The volar scapholunate ligament fibers course obliquely between the volar aspects of the lunate and scaphoid.
The lunotriquetral ligament is usually visualized as a thin horseshoe-shaped structure that may appear more lax than the scapholunate ligament on MR imaging.43 The lunotriquetral ligament does not extend as far distally into the lunotriquetral joint as the longer proximal distal portion of the scapholunate ligament does within the scapholunate joint.
The volar and dorsal portions of the lunotriquetral ligament attach directly to bone, whereas its midportion attaches to the hyaline articular cartilage of the lunotriquetral joint.43
Smith and Snearly53 have shown that on coronal MR images the lunotriquetral ligament is most commonly delta-shaped (triangular) or linear.
Arcuate Ligaments
More distally, there is a set of ligaments that stabilizes the distal carpal row on the proximal row. These ligaments have been referred to as the deltoid ligaments 54 or arcuate ligaments (Fig. 10.75)55 and include the following:
The ulnar arcuate ligament extends from the volar surface of the lunate and triquetrum to the neck of the capitate
and plays a role in preventing the proximal row from volarflexion (see Fig. 10.75). Progressing radially, the substance of this structure becomes quite thin in the region of the capitolunate articulation.
The radial limb (i.e., radial arcuate ligament) of this V-shaped ligament runs from the capitate to the distal pole of the scaphoid.
![]() FIGURE 10.68 ● A coronal section of the wrist joint shows the articular surfaces and triangular cartilage. |
![]() FIGURE 10.69 ● A T2*-weighted image showing the TFC complex. Black arrows, radial attachments of TFC; white arrows and UC, ulnar collateral ligament; M, meniscus homologue; pr, prestyloid recess. |
The thin tissue between the limbs of the arcuate or deltoid ligament is known as the space of Poirier. This weak area in the ligamentous floor of the carpus may function as a trap door through which the lunate or capitate may dislocate. The particular functions of these ligaments with respect to midcarpal instabilities are discussed below in the section on carpal instabilities. The proximal fibers of the radioscaphocapitate and ulnar arcuate ligament merge volar to the head of the capitate and are joined by reflected fibers distally from the TFC ligament. This creates a sling-like support for the head of the capitate and ham-ate.56 This sling can be appreciated as a thick hypointense band extending from the triquetrum to the scaphoid on axial images.
Distal Carpal Row Interosseous Ligaments
Within the distal row, the trapezius and trapezium, trapezoid and capitate, and capitate and hamate are connected by interosseous ligaments. In contradistinction to the interosseous ligaments of the proximal row, these ligaments do not extend from the dorsal to the volar surface; and there is normally communication between the midcarpal space and carpometacarpal joints.
Tendons of the Wrist and Hand
Palm of the Hand
The flexor retinaculum and palmar aponeurosis represent thickened deep fascia of the wrist (Figs. 10.76, 10.77, and 10.78). Important anatomic structures and features include the following:
The superficial palmaris longus tendon is fused to the midline of the flexor retinaculum and expands distally into the palmar aponeurosis.
Guyon’s canal, a site of potential compression of the ulnar nerve, is formed by an ulnar extension of the flexor retinaculum superficial to the ulnar nerve and artery.
The concave volar surface of the carpus and the flexor retinaculum form the anatomic boundaries of the carpal tunnel for passage of the long flexor tendons of the fingers and thumb (Fig. 10.79).
The four flexor digitorum superficialis tendons are arranged in two rows, with the tendons to the third (i.e., middle) and fourth (i.e., ring) digits superficial to the tendons for the second (i.e., index) and fifth (i.e., little) digits (Fig. 10.80). After entering their respective fibrous flexor sheaths, the tendons of the flexor digitorum superficialis divide into two halves opposite the proximal phalanx and partially decussate around the flexor digitorum profundus tendons. Distal to the site of perforation by the flexor digitorum profundus, the superficialis tendons pass deep to the flexor digitorum profundus and send slips to attach to the sides of the middle phalanx.
The tendons of the flexor digitorum profundus are arranged in the same plane and pass deep to the flexor digitorum superficialis (Fig. 10.81). The tendons of the flexor digitorum profundus attach distally to the base of the terminal phalanx and change from a deep to a superficial location at the partial decussation of the superficialis at the level of the middle phalanx (Fig. 10.82).
Dorsum of the Hand
The extensor digitorum tendons extend across the metacarpophalangeal joints and contribute to the posterior capsule of this joint, and then they broaden out onto the dorsum of the proximal phalanx (Fig. 10.83). The extensor expansion represents the joining of the extensor tendons and posterior fascia. The extensor tendons and expansion divide into a central slip, which inserts onto the base of the middle phalanx, and two lateral or marginal slips, which diverge around the central slip and then converge to insert onto the base of the distal phalanx. The interosseous (Fig. 10.84) and lumbrical tendons insert onto the dorsal extensor expansion from each of its sides and from its lateral side, respectively.
![]() FIGURE 10.76 ● The palmar aponeurosis is revealed by removing the skin and superficial fascia. The investing fascia has been removed proximal to the flexor retinaculum. R, radius. |
Pathology of the Wrist and Hand
Carpal Instabilities
Pearls and Pitfalls
Carpal Instabilities
Carpal instabilities can be grouped as perilunar, midcarpal, or proximal.
Perilunar instabilities include lesser arc injuries (primarily ligamentous [e.g., scapholunate or lunotriquetral injuries]) and greater arc injuries (primary osseous [e.g., scaphoid fracture]).
The terms stability and instability, as used in reference to conditions affecting the carpus, must be rigidly defined:
Stability refers to the ability of two structures to maintain a normal physiologic spatial relationship under applied physiologic loading.
Similarly, two structures are said to be unstable if they cannot maintain this normal relationship under physiologic loading conditions.
Carpal instabilities represent deviations from the normal spatial relationships of the carpal bones to each other and their surrounding structures, such as the radius, the ulna, and the metacarpals. Since the wrist, like the hip, is virtually always under some load condition, this definition includes instabilities that are seen on routine radiographs as well as those seen on motion studies.
MR imaging offers the advantage of revealing associated carpal ligament disruptions when characterizing instabilities in any specified plane of section. Fixed instabilities seen on routine radiographs and coronal or sagittal MR images are often referred to as static, and those that are revealed only by provocative maneuvers in motion studies are referred to as dynamic. Kinematic MR imaging represents a series of static evaluations displayed in a cine loop without true dynamic motion.
In many cases, the difference between dynamic and static instability is a matter of the degree of pathology in the structures that maintain the spatial relationship between the bones. A static instability that is present at all times implies contracture of the soft-tissue constraints. This condition may not be amenable to surgical repair and treatment may require arthrodesis. On the other hand, a dynamic instability that is revealed only by a provocative maneuver may be due to a relatively minor ligament tear or laxity that can be repaired with a soft-tissue procedure, allowing preservation of joint motion.
Stable and Unstable Equilibrium in the Wrist
The normal spatial relationships of the individual components of the wrist (e.g., carpal bones, radius, ulna, metacarpals) can be thought of as an example of a stable equilibrium condition. Definitions of stable and unstable equilibrium include:
A stable equilibrium exists when displacement of a body from its position results in a restoring force that tends to return the body to its equilibrium position. When all of the supporting constraints of the wrist are normal and intact, any load within physiologic limits may change the spatial relationship of the components, but there will be a simultaneous increase in the tension within these constraining structures that counteracts the deforming force and tends to return the components to their normal spatial relationship. Neutral, dorsiflexion, and palmarflexion motions can be used as an example of the different colinear relationships among the capitate, lunate, and radius (Figs. 10.85, 10.86, and 10.87).
Conversely, an unstable equilibrium exists when displacement of a body from its position results in a force that tends to push the body further from the equilibrium position.57 This condition occurs in the wrist when the constraining structures are incompetent. Constraining or supporting structures about the wrist include not only the ligamentous structures discussed earlier but also the tendons that cross the joint, as well as the geometry of the carpal bones and their surrounding articular surfaces.
The tendency for the wrist to assume a condition of unstable equilibrium when the constraining structures are damaged can be seen as an extension of the normal motions of the carpal bones. To understand these motions, the wrist can be thought of as a flexible spacer interposed between the hand (i.e., metacarpals) and the distal radius/ulna. The purpose of this deformable spacer is to maintain a constant distance or space between the base of the third metacarpal and the articular surface of the radius. This theory is supported by the fact that, with radial or ulnar deviation, the carpal height index does not change from its value as measured in the neutral position.58 The carpal height index or ratio is defined as the carpal height measured from the distal capitate surface to the proximal lunate surface, divided by the length of the third metacarpal. In normal individuals, the value is 0.54 ± 0.03. In pathologic conditions, such as SLAC wrist or rotatory subluxation of the scaphoid as seen in scapholunate dissociation, this ratio is decreased to less than 0.49.
For this ratio to remain constant with radial and ulnar deviation, there must be a change in the dimensions of the ulnar and radial borders of the flexible spacer between the metacarpals and distal radius and ulna. Since the bones of the distal carpal row are rigidly held in place by their ligamentous restraints and do not move, the normal motions of the three bones of the proximal row must account for the changes in the dimensions of the radial and ulnar borders of the wrist:
With radial deviation the radial border must shorten, and this is accomplished by rotation of the scaphoid into a flexed position.
The ulnar border is lengthened as the triquetrum slides out from beneath the hamate.
On plain radiographs or coronal MR images in this position, the scaphoid is foreshortened and the joint space is evident between the hamate and the triquetrum; no superimposition of these bones occurs.
The lunate is linked or associated with the scaphoid and triquetrum through the interosseous ligaments, which are displayed as homogeneous hypointense structures on coronal MR images. The scapholunate ligament has a triangular morphology, whereas the lunotriquetral ligament is more linear in shape. Lunate motion is thus a reflection of this proximal carpal row linkage, as well as the compressive forces placed on it by the capitate.
At extreme radial deviation, the summation of these forces produces slight volarflexion of the lunate. Since the wrist is in a condition of stable equilibrium, the bones of the proximal carpal row return to their neutral position when the force causing radial deviation is removed. Thus, in radial deviation, a flexion torque predominates, and compression of the scaphotrapeziotrapezoid joint and proximal carpal row flexion produce a physiologic VISI pattern.
With ulnar deviation, the radial side of the flexible spacer must lengthen and the ulnar side must shorten.
The scaphoid becomes more horizontal or extended to lengthen the radial side, and the triquetrum slides beneath the hamate to shorten the ulnar side.
PA radiographs show an elongated scaphoid and superimposition of the hamate on the triquetrum.
Coronal MR images demonstrate the triquetral movement in an ulnar direction on the slope of the hamate.
Palmar movement of the triquetrum in relationship to the hamate results in a palmar position of the lunate axis, relative to the capitate.
Compression forces transmitted by the capitate produce dorsal rotation or dorsiflexion of the lunate. Associated volar shift of the lunate maintains colinear alignment of the capitate and radius.
During lunate dorsiflexion, there is elevation of the distal pole of the scaphoid (i.e., scaphoid extension). Thus, in ulnar deviation, an extension torque predominates, and interaction at the triquetrohamate helicoid slope and proximal carpal row extension produces a physiologic DISI pattern.
Interosseous Ligament Pathology
MR imaging is a valuable tool in evaluating interosseous ligament pathology, with excellent sensitivity, specificity, and accuracy. For evaluation of the lunotriquetral ligament, 3DFT coronal images may be needed to improve the sensitivity of diagnosis. Thin-section axial images may also improve the identification
of both scapholunate and lunotriquetral ligament pathology. MR imaging is superior to conventional arthrography, allowing identification of the size, morphology, and location of a scapholunate or lunotriquetral ligament tear. This information is important because communication across a pinhole or small perforation or deficiency of the thin membranous portion of the ligament may not be significant in the presence of grossly intact dorsal and volar ligaments. In fact, evaluations of communicating perforations in cadaver wrists have shown that 28% of cadaver wrists have degenerative perforations of the central membranous scapholunate ligament.59 Degenerative perforations of the membranous lunotriquetral ligament can be seen in 36% of cadaver wrists. In our experience, degenerative perforations occur twice as often in the lunotriquetral ligament as in the scapholunate ligament.
of both scapholunate and lunotriquetral ligament pathology. MR imaging is superior to conventional arthrography, allowing identification of the size, morphology, and location of a scapholunate or lunotriquetral ligament tear. This information is important because communication across a pinhole or small perforation or deficiency of the thin membranous portion of the ligament may not be significant in the presence of grossly intact dorsal and volar ligaments. In fact, evaluations of communicating perforations in cadaver wrists have shown that 28% of cadaver wrists have degenerative perforations of the central membranous scapholunate ligament.59 Degenerative perforations of the membranous lunotriquetral ligament can be seen in 36% of cadaver wrists. In our experience, degenerative perforations occur twice as often in the lunotriquetral ligament as in the scapholunate ligament.
![]() FIGURE 10.81 ● Removal of the tendons of the flexor digitorum superficialis reveals the attachments of the lumbrical muscles to the tendons of the flexor digitorum profundus. |
![]() FIGURE 10.83 ● (A) The extensor tendons are arranged into six compartments on the dorsum of the wrist. (B, C) Extensor tendon anatomy on T1-weighted coronal MR images (A is dorsal to B). |
![]() FIGURE 10.84 ● The dorsal interossei is exposed by removing the deep fascia and the tendons of the extensor digitorum. |
MR arthrography has increased the accuracy of evaluation of scapholunate and lunotriquetral ligament tears, especially in peripheral ligament avulsions where the ligament has not lost its normal morphology. These tears may be difficult to detect on routine MR gradient-echo, STIR, or FS PD FSE images unless there is a fluid interface identified between the torn ligament and corresponding osseous attachment.
Scapholunate Ligament Tear
As discussed earlier, the scapholunate ligament complex consists of both intrinsic and extrinsic ligaments. The extrinsic ligaments provide gross stability and the intrinsic ligaments provide fine-tuning for stability. The dorsal component of the scapholunate interosseous ligament is trapezoidal, the membranous component is wedge-shaped, and the volar component consists of thin slightly oblique fibers. The separate scaphotrapeziotrapezoid ligament complex provides significant volar constraint and consists of the following structures:
Scaphotrapezial ligament
A thin palmar scaphotrapeziotrapezoid capsule/thick fibrous floor of the flexor carpi radialis tendon sheath
Scaphocapitate capsular ligament
Dorsal capsular ligament (minimal contribution to stability).
Pearls and Pitfalls
Scapholunate Ligament Tear
All three components of the scapholunate ligament should be evaluated on coronal and axial images.
Dorsal component scapholunate ligament tears are more likely to be symptomatic.
Scapholunate ligament failure represents stage I perilunar instability.
Scaphotrapeziotrapezoid articular injuries may be required for more advanced scapholunate instability patterns to exist.
Tears of the scapholunate ligament are the most common type of carpal instability and may occur as a residual of a perilunate injury or as an isolated injury. They usually occur in adults, with an equal distribution between men and women, and are often associated with scaphoid and distal radial fractures. Sports that place dorsal stress on the carpus (loading the wrist in palmar flexion) put the scapholunate ligament at risk.
Etiology and Clinical Features
The mechanism of injury is axial compression/hyperextension with intercarpal supination or ulnar deviation, as would occur in a forward fall onto an outstretched hand or a backward fall on a pronated hand. Scapholunate dissociation requires injury to both the scapholunate intrinsic ligament and the extrinsic radioscaphocapitate ligament.
If an injury to ligamentous constraints occurs, such as a tear of the scapholunate ligament, the linkage between the scaphoid and lunate is removed and these bones become dissociated. A scapholunate ligament tear, however, may exist without a static instability (Fig. 10.88). In this case, the lunate is no longer under the influence of the scaphoid and instead follows the triquetrum, and the loading force of the capitate is not opposed by torque transmitted through the scapholunate ligament from the flexed scaphoid. Similarly, the lunate no longer exerts force on the scaphoid, and there is less opposing force to its flexion.
Radial deviation produces an exaggeration of the normal motions of the bones of the proximal row. With scapholunate interosseous ligament disruption, the scaphoid becomes more flexed in relation to the lunate, and the scapholunate angle, normally less than 30° to 60°, increases to more than 70° (Fig. 10.89). The scapholunate angle is determined from two sagittal images to demonstrate the separate lunate and scaphoid axes, which are not shown together in the same sagittal image. The lunate, free of the influence of the scaphoid, tips into a dorsiflexed position in relation to the axis of the capitate. As the scaphoid flexes, a gap appears between the scaphoid and the lunate, and in time the capitate will fall into this gap, contributing to a reduction in carpal height.
Rotatory subluxation of the scaphoid, which begins as an scapholunate dissociation, may also occur. In its final stages, as the lunate is dorsiflexed, a DISI pattern is established. On lateral radiographs, there is 10° or more of lunate dorsiflexion relative to the radius. On sagittal MR images, dorsal tilting of the lunate is associated with proximal migration of the capitate and loss of colinear alignment of the capitate, lunate, and radius. The capitolunate angle, normally 0° to 30°, can be directly measured on sagittal images and may be increased in dorsiflexion ligamentous instability.
Clinically patients present with pain and tenderness at the anatomic snuffbox. The Watson test (scaphoid shift test) for scapholunate ligament instability is positive, with pain on ulnar to radial deviation. and there is often an audible click at the scapholunate interval.
Pathologic Changes and MR Appearance
In evaluating scapholunate interosseous ligament pathology, MR imaging has an overall sensitivity rate of 93%, specificity of 83%, and accuracy of 90% when compared with arthrography.23 In the diagnosis of ligament tears, with arthroscopy as the gold standard, MR imaging is 86% sensitive, 100% specific, and 95% accurate. The scapholunate ligament is uniformly visualized on coronal T2*-weighted images with 3-mm sections. In 90% of coronal plane images, the scapholunate ligament displays a triangular morphology; in 10% of cases, it demonstrates a linear morphology.51,59 In 63% of cases studied by Smith,51 the scapholunate ligament was seen as a homogeneous low- or low- to intermediate-signal-intensity structure. In 37% of cases, there were intermediate-signal-intensity areas traversing portions of the scapholunate ligament (part or all of the ligament), which could be potentially mistaken for a tear.
The following findings are characteristic of scapholunate ligament pathology:
Disruption of the scapholunate ligament is shown on T2*-weighted or STIR images as either complete ligamentous disruption or as a discrete area of linear hyperintensity in a partial or complete tear (Fig. 10.90).
In complete tears, synovial fluid communication between the radiocarpal and midcarpal compartments may be identified. Associated stretching (i.e., redundancy) or tearing of the radiolunate ligament and the radioscaphocapitate ligament is shown on sagittal images.
A flap tear may not be appreciated without the use of MR arthrography. MR arthrography is also helpful in evaluation of perforations and integrity of the dorsal component of the scapholunate ligament.
A perforation, identified by communication of fluid across a focal discontinuity, constitutes a communicating defect or tear. Small membranous perforations may exist in the presence of intact dorsal and volar portions of the scapholunate ligament. In fact, most degenerative perforations occur in the thin membranous portion of the scapholunate ligament, which is not thought to be biomechanically significant.59,60
Partial-thickness perforations or noncommunicating defects may be associated with ligamentous tissue degeneration or sprains and may be difficult to appreciate on MR images.61
A complete scapholunate ligament tear may not be associated with scapholunate interval diastasis or static carpal instability as assessed on sagittal images, especially when the volar extrinsic ligaments are intact.
Axial MR images are used to distinguish among tears of the dorsal, membranous, and volar portions of the scapholunate ligament. The location of the tear can then be directly correlated with dorsal or volar coronal images.
The scaphoid attachment of the scapholunate ligament is more likely to avulse than is the stronger lunate attachment.59 The insertion of the scapholunate ligament into hyaline cartilage covering the scaphoid is thus relatively weak. In fact, a scapholunate ligament
tear may be associated with a scaphoid avulsion fracture (Fig. 10.91).
Potential sites of injury of the intrinsic scapholunate ligament complex (the scapholunate interosseous ligament) (Fig. 10.92) include its dorsal component (an important key stabilizer), a membranous component, and a volar component.
Less frequently injured is the extrinsic radiocarpal ligament complex, composed of the radioscaphocapitate, the long radiolunate (radiolunotriquetral), the short radiolunate, and the radioscapholunate (ligament of Testut). Associated synovitis, ganglions, or ligamentous hyperintensity may be seen in trauma.
The size of the tear varies depending on associated osseous or ligamentous disruption of major carpal links; the scaphotrapeziotrapezoid is the radial link and the triquetrohamate is the ulnar link.
Acute injuries (less than 6 weeks) may be classified as either stable injuries (partial ligament disruption) (Fig. 10.93) or dynamic or static unstable injuries (complete ligament disruption) (Fig. 10.94).
Chronic injuries are considered to be stable, unstable, or fixed. They are associated with a variety of secondary changes, including capsular contractures, degenerative arthritis, pancarpal arthrosis, intercarpal collapse, and SLAC wrist (progressive proximal capitate migration, radioscaphoid and capitolunate arthrosis in untreated scapholunate dissociation). DISI deformity with midcarpal collapse may also be seen.
The scaphoid attachment of the scapholunate ligament has fewer Sharpey’s fibers than the lunate attachment and is therefore more susceptible to tearing (Fig. 10.95).
In review, typical findings include:
Dissociation (Fig. 10.96), indicated by an increased scapholunate gap (more than 3 mm), comparable to the Terry Thomas sign on radiography
Volar or palmar flexion of the scaphoid on sagittal images (Fig. 10.97)
DISI with dorsal tilting of lunate, an increased capito-lunate angle (more than 30°), and an increased scapho-lunate angle (more than 80°) (Fig. 10.98)
Linear signal in a partial or complete tear
Complete ligamentous disruption with a fluid-filled gap and synovial fluid communication between the radiocarpal and midcarpal compartments
Disruption of the dorsal component of the scapholunate ligament
Associated synovitis of the extrinsic volar radiocarpal ligaments
Degenerative perforations in the membranous portion with intact volar and dorsal components (Fig. 10.99)
Associated dorsal ganglions in the dorsal or dorsal/membranous scapholunate ligament pathology (Fig. 10.100).
![]() FIGURE 10.95 ● Preferential disruption of the dorsal and membranous scapholunate ligament from the scaphoid, which has a weaker attachment to the interosseus ligament. Coronal FS PD FSE image. |
![]() FIGURE 10.96 ● Scapholunate (SL) dissociation (A) associated with disruption of all three components of the scapholunate ligament. (B). (A) Coronal FS PD FSE image. (B) Axial FS PD FSE image. |
![]() FIGURE 10.97 ● Scaphoid flexion (A) associated with disruption of the dorsal, membranous, and volar fibers of the scapholunate ligament (B). (A) Sagittal T1-weighted image. (B) Axial FS PD FSE image. |
![]() FIGURE 10.99 ● Absence of membranous fibers of the scapho-lunate ligament allows direct extension of fluid from the radiocarpal joint. Coronal FS PD FSE image. |
Classification
An arthroscopic classification scheme divides scapholunate ligament injury into four grades: grades I and II are partial tears and grades III and IV are complete disruptions. Perilunar instability (PLI) is divided into four stages:
Stage I: scapholunate failure
Stage I: capitolunate failure
Stage III: triquetrolunate failure
Stage IV: dorsal radiocarpal ligament failure with volar rotation of the lunate
Treatment
Conservative treatment with cast immobilization may be used, but surgery may be necessary. Surgical approaches for acute injury include close reduction and internal fixation or open reduction and primary repair or reconstruction. In chronic injuries, surgery includes open reduction and reconstruction or even arthrodesis.
The lunotriquetral ligament has dorsal, volar, and membranous (a fibrocartilaginous membrane) components. The lunate and triquetrum are stabilized by intrinsic and extrinsic carpal ligaments.
A loss of linkage (i.e., dissociation) between the triquetrum and the lunate, due to a tear of the lunotriquetral ligament, allows the lunate to follow the scaphoid. Under this influence, volarflexion of the lunate occurs and gives rise to a VISI pattern. VISI may be defined as a carpal instability characterized by proximal and volar migration of the bones of the distal row, associated with flexion of the lunate. The scapholunate angle is decreased to less than 30°, and the capitolunate angle may measure up to 30°.
Lunotriquetral ligament tears are less common than scapholunate ligament injuries and are considered part of the perilunar injury pattern. Membranous perforations are found in 13% of individuals over 40 years of age.
Etiology, Pathology, and Clinical Features
Pro-gressive perilunar injury/instability is caused by a palmar radial force with dorsiflexion and ulnar deviation. Lunotrique-tral ligament injury represents stage III PLI (triquetral dislocation with radiotriquetral ligament failure). It often occurs after scapholunate ligament injury or fracture (stage I PLI) and lunocapitate dissociation (stage II PLI). Perilunar instability results in DISI unless the scapholunate ligament heals, in which case the VISI pattern predominates (the forme fruste of stage III PLI). A perilunar pattern of injury is associated with intercarpal supination and injury from a radial to ulnar direction. An isolated lunotriquetral ligament sprain secondary to ulnar-sided injury is more common than concomitant radial injury. Reverse perilunar injury, which originates on the ulnar side of the wrist instead of the radial side, is caused by intracarpal pronation and radial deviation that force the capitate into the lunotriquetral joint. Isolated lunotriquetral ligament injury may also be seen with a palmarflexion mechanism of wrist injury. A combined scapholunate dissociation and lunotriquetral ligament injury is less common. Lunotriquetral ligament lesions may also be associated with inflammatory arthritis, increased load transmission across the ulnar side of the wrist, and ulnar positive variance (in particular, membranous lesions with ulnocarpal [ulnolunate] impaction are seen in positive ulnar variance).
Lunotriquetral Ligament Tear
Pearls and Pitfalls
Lunotriquetral Ligament Tear
Lunotriquetral tears may be associated with ulnocarpal impaction syndrome.
The carpal arc offset between the triquetrum and lunate should be assessed.
Lunotriquetral ligament injury occurs as part of stage III PLI, which includes triquetral dislocation and radiotriquetral ligament failure.
Pathologic examination reveals a partial to complete lunotriquetral ligament tear and palmarflexion of the lunate. Fractures of the radial styloid or scaphoid may be seen as well as disruption of the scapholunate joint as part of stage III PLI. There is associated synovitis, joint wear/degeneration, abnormal ligament tension, and variable amounts of collagen fibers, connective tissue, and elastic fibers.
Symptoms vary depending on whether the tear is partial or complete. There may be a painful wrist click in radial or ulnar deviation, and stiffness, weakness, and instability are common. The history may include a traumatic event such as a fall on an outstretched hand or a twisting/rotatory injury. In complete lunotriquetral dissociation, there is static carpal collapse. Stress tests for lunotriquetral mobility, pain, and crepitus include the ulnar-side compression test, the lunotriquetral ballottement test, and the dorsal-palmar shear test.
Radiographic findings include disruption of the normal convex arc (Gilula’s lines) of the proximal carpal row and a step-off between lunate and triquetrum.
MR Appearance
In the diagnosis of lunotriquetral interosseous ligament tears, MR imaging is 56% sensitive, 100% specific, and 90% accurate when compared with arthrography, and 50% sensitive, 100% specific, and 80% accurate when compared with arthroscopy.23 Since the lunotriquetral ligament is less substantial than the scapholunate ligament, 3DFT coronal images may be needed to improve the sensitivity of diagnosis. Axial images are used to separate the volar, membranous, and dorsal components of the ligament since they are more difficult to appreciate on coronal images. In addition, unlike scapholunate dissociations, osseous widening of the lunotriquetral articulation is uncommon, which may make the detection of lunotriquetral ligament pathology more difficult, especially in the absence of adjacent fluid (secondary to an effusion or its introduction in MR arthrography). FS PD FSE sequences or MR arthrography is used for detection and characterization of insertional site tears, flap tears, perforations, separation of the individual lunotriquetral ligament components,
associated TFC complex lesions, and associated chondral lesions of proximal lunate, triquetrum, and distal ulna in cases of positive ulnar variance. Improved visualization of radiocarpal and midcarpal communication is also afforded by these sequences.
associated TFC complex lesions, and associated chondral lesions of proximal lunate, triquetrum, and distal ulna in cases of positive ulnar variance. Improved visualization of radiocarpal and midcarpal communication is also afforded by these sequences.
Characteristic MR findings include the following:
Disruption of the lunotriquetral ligament is most frequently identified by a fluid-filled gap on coronal images (Fig. 10.101).
An insertional site tear or perforation is often appreciated on MR arthrography. FS PD FSE sequences provide sufficient contrast to produce an arthrogram-like effect without injection of contrast (Fig. 10.102).
Lunotriquetral ligament tears may be associated with TFC degenerative tears.43
A VISI pattern usually requires disruption of both the lunotriquetral intrinsic and dorsal extrinsic ligaments (i.e., dorsal radiocarpal ligament).
Smith and Snearly53 described the intact lunotriquetral ligament as linear in 63% and delta-shaped in 35% of asymptomatic individuals (Fig. 10.103).
The intact lunotriquetral ligament demonstrates homogeneous hypointensity in 73% and linear intermediate signal intensity within its substance in 25% of wrists studied.53 Insertional signal intensity varies with the distribution of hyaline articular cartilage on either side of the lunotriquetral ligament.
Asymmetric hyaline articular cartilage signal intensity should not be mistaken for an eccentric tear unless fluid signal intensity communication is seen across the ligament cartilage interface. Linear fluid signal intensity communicating between the radiocarpal and midcarpal compartments through lunotriquetral space indicates tearing.
Sagittal MR images are used to assess static VISI instability with palmar tilting of the lunate and scaphoid (Fig. 10.104).
There may be discontinuity of the normal lunotriquetral ligament across the lunotriquetral interval (Fig. 10.105)
All lunotriquetral ligament components may be affected (Fig. 10.106), including the dorsal, membranous, and volar components.
The size of the tear varies based on associated injuries, including distal radioulnar joint subluxation and arthritis, chondromalacia of the distal ulna, TFC complex injury, and triquetrohamate instability.
There may be flap tears at the triquetral attachment or complete absence of the horseshoe-shaped ligament (Fig. 10.107).
Loss of the normal smooth convexity of the proximal carpal row is not uncommon.
Disruption of the lunotriquetral interval is pronounced with ulnar deviation.
There may be dorsiflexion of the triquetrum relative to the lunate on sagittal images.
On coronal images, triquetral offset may be distal relative to the lunate convexity.
An increased distance between the lunate and the triquetrum is sometimes seen, although it is not common (Fig. 10.108).
Extension of fluid is possible with membranous degeneration but intact dorsal and volar lunotriquetral ligament components.
The scapholunate angle is less than 30° to 40° and the capitolunate angle is greater than 10°.
Associated findings of ulnocarpal (ulnolunate) impaction are frequently seen with lunotriquetral ligament tears (Fig. 10.109).
Ganglions (Fig. 10.110) are less commonly associated with lunotriquetral ligament tears than with scapholunate ligament injury.
Treatment
Progression from minimal symptoms to ulnar wrist pain, a sensation of instability, ulnar nerve paresthesias, and eventual VISI deformity is typical. Conservative treatment includes immobilization, anti-inflammatory agents, and wrist splinting for acute injuries. Acute lunotriquetral dissociation with static deformity and chronic symptoms not responsive to immobilization may require surgery. Surgical treatment of lunotriquetral dissociations can be approached in two ways. If there is no VISI and positive ulnar variance is present, ulnar shortening unloads the lunotriquetral interval and provides excellent symptomatic relief. The torn lunotriquetral ligament should be repaired if possible. Lunotriquetral intercarpal fusion is also an option, although meticulous technique is required to avoid pseudoarthrosis. Lunotriquetral fusion is an attractive concept because this is the most common carpal coalition. These coalitions are usually asymptomatic and are
often diagnosed in radiographs taken for other reasons. However, achieving a stable lunotriquetral fusion has proven difficult, and most series show significant rates of nonunion (i.e., up to 40%). Techniques using larger bone grafts and compression fixation devices have improved the success of this procedure. We have had 100% success in achieving fusion using the Herbert bone screw with precompression of the fusion site.
often diagnosed in radiographs taken for other reasons. However, achieving a stable lunotriquetral fusion has proven difficult, and most series show significant rates of nonunion (i.e., up to 40%). Techniques using larger bone grafts and compression fixation devices have improved the success of this procedure. We have had 100% success in achieving fusion using the Herbert bone screw with precompression of the fusion site.
![]() FIGURE 10.102 ● Flap tear of the lunotriquetral ligament associated with TFC tear and proximal lunate chondromalacia. Coronal FS PD FSE image. |
Classification of Wrist Instabilities
There are numerous classification schemes for instability of the carpus. The most useful classification reflects the anatomy and pathophysiology of the condition and indicates treatment. Four characteristics are described in classification of carpal instabilities:
Severity (categorized as dynamic, static subluxation, or static dislocation)
Direction of displacement: dorsal (DISI), volar (VISI), or radioulnar/proximodistal translation (e.g., ulnar, radial, distal, proximal)
Location of injury
Type of injury
Proximal or distal displacements are represented by axial carpal dislocations.62 These are rare traumatic injuries, usually associated with a crush or blast mechanism. There is a longitudinal transarticular derangement of both the carpal and metacarpal transverse arches, with complete loss of the normal relationship between the two columns of the carpus. The radiologic hallmarks are an abnormal widening of any joint between the bones of the distal carpal row, a disruption of Gilula arc III defined by the proximal articular surfaces of the bones of the distal row, and an abnormal gap between the bases of two adjacent metacarpals.63 Gilula’s arcs are used to demonstrate parallelism of the articular alignment of the carpal bones. A disrupted arc with widening or offset of joint spaces is associated with ligamentous, osseous. or joint injury.
Carpal instabilities can be grouped into perilunar (perilunate) instabilities, midcarpal instabilities, and proximal carpal instabilities.
Perilunate instabilities may be divided into lesser and greater arc injuries. Lesser arc injuries involve disruptions that follow the contour of the lunate itself, whereas greater arc injuries are transscaphoid, capitate, hamate, or triquetral. Perilunar instability includes dorsal perilunate dislocation and palmar lunate dislocation.
Lesser arc injuries involve scapholunate ligament, lunotriquetral ligament, and complete perilunate instabilities.
Greater arc injuries are divided into scaphoid fractures, naviculocapitate syndrome (scaphoid fracture plus perilunate dislocation), and transscaphoid transtriquetral perilunate dislocation.
Mayfield’s spectrum of progressive lunate instability is shown in four stages.64 The stages were defined experimentally in cadaver wrists by in vitro simulation of a fall on an outstretched hand. The wrist was forced into progressive dorsiflexion, ulnar deviation, and intercarpal supination, and progressive injuries were noted:
Stage I: The scapholunate ligament fails, leading to an scapholunate ligament instability.
Stage II: There is progression to failure of the midportion of the radioscaphocapitate ligament.
Stage III: There is failure of the capitolunate and triquetrolunate joints with failure of the radiotriquetral ligaments.
Stage IV: There is failure of the dorsal radioscapho-lunotriquetral ligament, resulting in complete palmar dislocation of the lunate.
Midcarpal instabilities
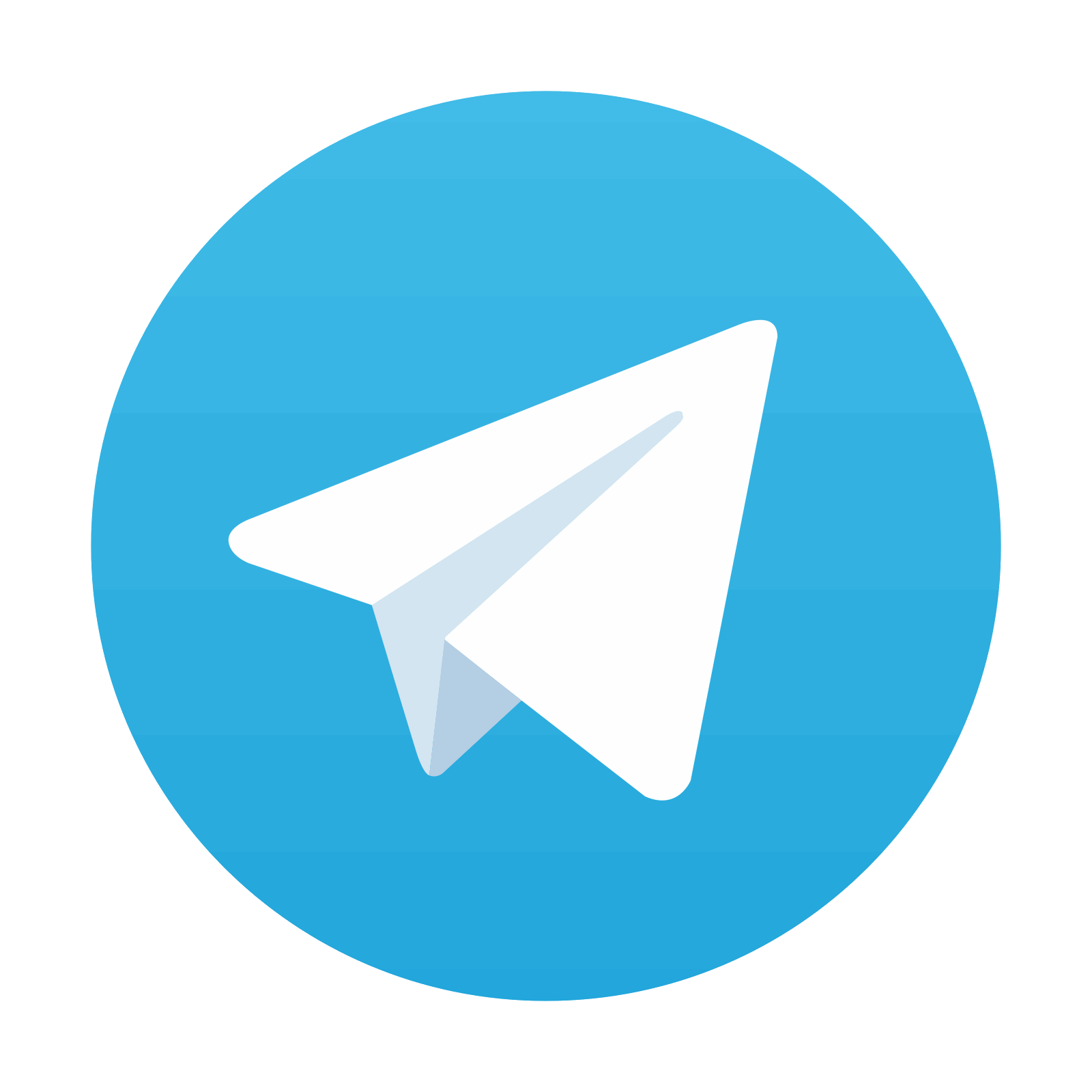
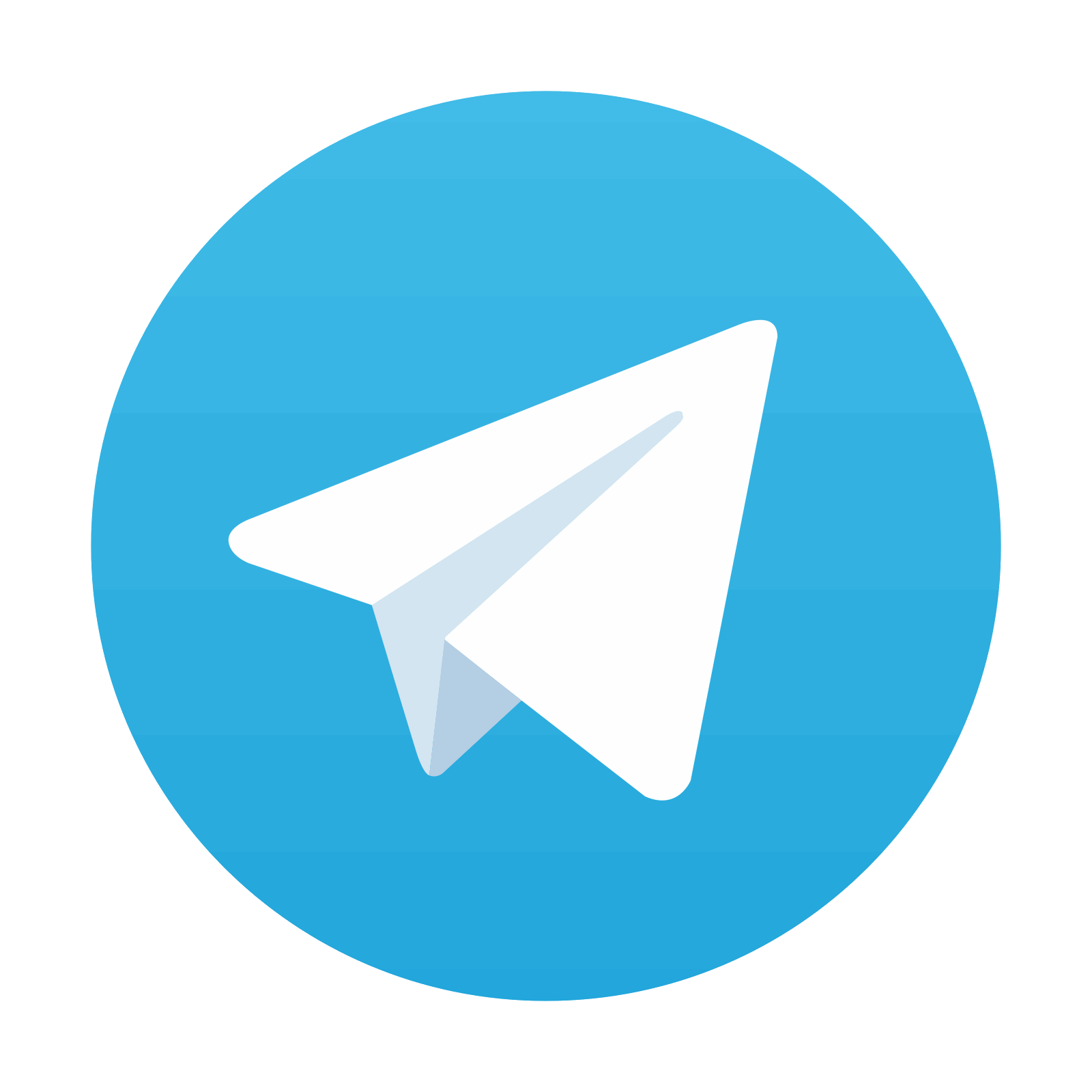
Stay updated, free articles. Join our Telegram channel

Full access? Get Clinical Tree
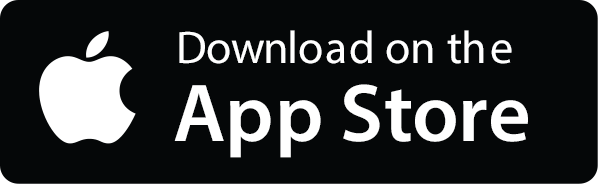
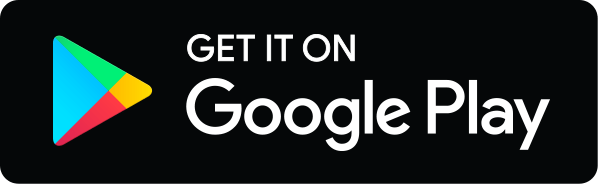
