The Principles of Pediatric Fracture and Trauma Care
Martin J. Herman
James J. McCarthy
INTRODUCTION
Fractures are common in children. Nearly one of three children will have at least one fracture during childhood (3). Orthopaedic surgeons, pediatricians, emergency room, personnel and primary care physicians will all be commonly exposed to fractures and other musculoskeletal trauma in children and, therefore, must have an understanding of the basic diagnostic and treatment principles. The general assumption is that fractures in children heal with little intervention and any deformity will remodel. Although this may be true much of the time, it is critical to be able to determine which injuries require greater attention and need intervention. For example, lateral condyle fractures may be more likely to go on to a nonunion, while physeal fractures are more likely to cause a growth arrest. It is also important to challenge the diagnosis and recognize that other disorders may first present as an acute injury, such as bone tumors diagnosed after a sports-related injury (1) or osteomyelitis seen within several days of a bone contusion from a fall (2). The purpose of this chapter is to provide a practice-based overview of fracture care in children. Fracturespecific information will be provided in the following chapters.
The specific management of each of the various pediatric fracture types is beyond the scope of this chapter. The emphasis here is on the general principles of fracture management in the pediatric age group. The specific management of the individual fracture types is very adequately covered in the two major textbooks devoted specifically to Fractures in Children.
Incidence.
Fractures make up about 25% of injuries in children. The patterns and incidence depend on a number of variables including age, sex, climate, time of year, and environmental and cultural differences. Several studies have reported on the incidence of fractures in children (3, 4 and 5). In children from birth to 16 years of age, 42% of boys and 27% of girls suffer a fracture and about 2% of children sustain at least one fracture per year (3). The vast majority of these do not require an inpatient admission (5).
The number of fractures increases with age in boys into their late teen years. Girls have a similar pattern of incidence of fractures when young, but fracture occurrence decreases prior to the teenage years (3, 5). These injury patterns differ from the incidence of other types of childhood injures, such as head and soft-tissue injuries, which peak by age 2 (6). Child abuse as an etiology of fractures is less common as children age but must always be considered when fractures occur in young children, especially prior to walking age.
Fracture incidence varies by location. Upper extremity fractures account for two-thirds of childhood fractures, with the forearm being the most common location. The most commonly fractured bone in children is the radius (4). Physeal fractures make up about 20% of fractures in children (7). Open fractures are uncommon in children, occurring in only 2% of fractures, and multiple fractures occur in 4% of injuries (5).
Prevention.
Worldwide, over 830,000 children die each year from accidental injuries such as motor vehicle accidents, drowning, falls, and firearm injuries. Accidents are the leading cause of death in children 1 to 14 years of age (www.safekids.org). These also result in 1.5 million bone and muscle injuries in the United States that require medical treatment among children 5 to 14 years of age. Injuries, including fractures, occur under many different circumstances. Although not all fractures and childhood injuries can be prevented, there are many ways in which the rate and severity of injuries can be decreased. An understanding of the causes and prevention strategies of childhood injuries is important for the health care providers, so they can share this with their patients and their caregivers. Several national and international organizations focus on injury prevention and serve as excellent sources of information for physicians and patients, such as the Pediatric Orthopaedics Society of North America (http://www. posna.org), which is maintained in cooperation with the American Academy of Orthopaedic Surgeons (http://www.aaos.org) and the American Academy of Pediatrics (http://www.aap.org).
Injuries and fractures most commonly occur at home, especially in the younger child. Most of these injuries are
low-energy injuries, such as falls from low heights. Falls from windows can be much more serious, especially in an urban environment with high-rise buildings and concrete sidewalks. Serious injury or mortality occurs most frequently in falls from heights greater than three stories (8). Considering the amount of time that children spend at school, proportionally few injuries occur there. Fewer than 10% of all injuries occurring in this age group happen at school. Most of these injuries are minor sprains and contusions and are associated with athletic activities; fractures infrequently occur at school (9).
low-energy injuries, such as falls from low heights. Falls from windows can be much more serious, especially in an urban environment with high-rise buildings and concrete sidewalks. Serious injury or mortality occurs most frequently in falls from heights greater than three stories (8). Considering the amount of time that children spend at school, proportionally few injuries occur there. Fewer than 10% of all injuries occurring in this age group happen at school. Most of these injuries are minor sprains and contusions and are associated with athletic activities; fractures infrequently occur at school (9).
Playground.
About 1% of children who use playgrounds sustain injuries, mostly from activities on playground equipment, according to one study. While most injuries are fractures (39%) and soft-tissue injuries, such as contusions and sprains (48%), traumatic brain injuries are not uncommon (8.5%). Most children, however, do not require hospital admission for playground injuries (10). Changing playground surfaces from concrete to more impact-absorbing surfaces, such as bark or sand, can reduce the incidence and severity of head injury and potentially other injuries. Fracture risk, however, may be more related to the height of the fall than to the playground surface (11).
Sports.
The four sports activities associated with the most injuries to bones and muscles are bicycle riding, basketball, football, and roller sports (12). Over 400,000 injuries occur to children in the United States while bike riding. While most are minor soft-tissue injuries, over one-third are fractures. More significantly, bicycle accidents are the most common causes of serious head injury in children (13). A well-fitting helmet is the best source of protection. Unfortunately not all children use helmets. Recently, however, evidence suggests that the use of helmets is increasing over the last several years (14). Roller sport injuries include those from skateboarding, roller skating, and inline skates. These activities commonly involve fractures, most often to the upper extremity. Skateboarding tends to result in the most severe injuries (15), with a high incidence of fractures. Inline skating also frequently results in musculoskeletal injury. In one report, as many as 60% of inline skaters sustained a traumatic injury, mostly contusions but occasionally fractures (16). Injuries often occur early in the child’s roller sport experience (17). Ice skating has a higher incidence of head injury than roller skating, making use of head protection for these athletes particularly important (18). Appropriate instruction, supervision, and protective equipment, including helmets, wrist guards, and elbow and knee pads, should be mandated (18). Trampoline-related injuries are becoming much more common and, like skateboard injuries, are frequently high-energy injuries. These injuries typically occur in the home setting often under the supervision and with the knowledge of the parents, most of whom know of the potential dangers of trampoline use (21, 22). Jumping with more than one person on the trampoline increases the risk of injury significantly. It is unclear if trampolines at home or on playgrounds can ever be used safely, and some advise that trampolines be used only in supervised training programs (20). Motorized recreational sports, such as motocross and use of all-terrain vehicles, are also associated with serious musculoskeletal injuries when safety guidelines are disregarded. Vehicles that are too heavy or powerful to be handled properly and poor driver judgment are important factors in injury occurrence.
Motor Vehicle Accidents.
Motor vehicle accidents are the most common cause of death for children and adolescents in the United States. Vehicle-versus-pedestrian accidents are about twice as common as injuries sustained by children who are occupants in a car involved in a crash. Child safety seats can reduce fatal injuries by 71% for infants younger than 1 year old and reduce fatal injuries by 54% for toddlers 1 to 4 years old. The character of injuries in restrained children is different compared to those unrestrained, with fewer fractures occurring but with higher proportion of femur, spinal, and pelvic injuries. Appropriate use of child safety car seats, combined with sitting the child in the rear seat (center position ideally) and never in the front seat with an airbag, is critical for the prevention of injury in a motor vehicle accident. All parents should be made aware of the safety guidelines for child restraints in motor vehicles established by the American Academy of Pediatrics, not only to minimize the risk of injuries in motor vehicle crashes, but also to facilitate adherence to state and federal guidelines for safe transportation of children.
CHARACTERISTICS OF GROWING BONE
The immature skeleton differs from that of adults in many ways that must be appreciated because these characteristics directly impact the diagnosis and treatment of musculoskeletal injuries.
Anatomy Unique to the Growing Bone.
Important anatomical and physiological features distinguish children’s bones from those of adults. Like adult bone, the diaphysis of long bones is comprised of dense cortical bone and the metaphysis is comprised of spongy, cancellous bone. Toward the ends of long bones, however, is where the child’s bone differs from that of an adult. There are four distinct areas that are associated with growth and remodeling processes. These include the epiphysis, physis, metaphysis, and diaphysis. Each area has its own unique structural and biomechanical characteristics.
Epiphysis.
The epiphysis is the site of secondary bone ossification that determines the size and shape of the articular surface. The articular surface enlarges via the small area of endochondral ossification that is located in the subchondral area. The epiphysis is also the resting site of osteoprogenitor cells, or chondrocytes, that develop into the components of the physis. Ligaments take their origin from or insert into the epiphyses at many joints, such as at the knee or ankle.
Apophysis.
An apophysis is smaller site of secondary ossification located at a tendon origin or insertion, such as the anterior
superior iliac spine (origin of the sartorius muscle) or the olecranon apophysis (insertion of triceps). Apophyses enlarge bones by appositional growth but do not significantly contribute to longitudinal growth. Physeal growth and the appearance of the secondary ossification centers occur in a predictable manner as the child matures. The growth potential of the child and the constant change in skeletal composition with the addition and maturation of growth centers can at times make the diagnosis and care of children’s fractures more challenging than adults.
superior iliac spine (origin of the sartorius muscle) or the olecranon apophysis (insertion of triceps). Apophyses enlarge bones by appositional growth but do not significantly contribute to longitudinal growth. Physeal growth and the appearance of the secondary ossification centers occur in a predictable manner as the child matures. The growth potential of the child and the constant change in skeletal composition with the addition and maturation of growth centers can at times make the diagnosis and care of children’s fractures more challenging than adults.
Physis.
The physis, or growth plate, is a narrow band of cartilage that lies between the epiphysis at the end of the bone and the metaphysis. Because it contains an expandable matrix, it can permit longitudinal bone growth. The physis or growth plate is the most important feature that differentiates bones of children from that of an adult. Longitudinal bone growth occurs primarily through the replacement of a cartilage anlage by a process termed endochondral ossification. The physis is highly organized at the cellular level into columns that span the epiphyseal end and the metaphysis. The germinal zone is closest to the articular surface and contains the resting chondrocytes, the precursors of new bone. Guided by local and systemic growth factors, these cells divide, forming the proliferating zone (27). In the hypertrophic zone, adjacent to these cells, the chondrocytes enlarge and mature. As these cells begin to degenerate, vascular budding from the adjacent metaphysis triggers the dystrophic calcification of the matrix producing the so-called zone of provisional calcification. Normally, the Ca and PHO4 ions are in a state of super-saturation in many areas of the body. Living cells produce a substance that prevents the Ca and PHO4 ions from precipitating. Because the cartilage cells in this area of the physis are degenerating, they can no longer prevent the precipitation of the calcium and phosphate salts in the matrix. It needs to be emphasized that this process of calcification is degenerative and does not contribute directly to the ossification process.
Metaphysis.
As the metaphyseal vessels migrate into this dead cartilage and its degenerated matrix, they bring in either chondroclasts or osteoclasts that reabsorb the dead cartilage cells and replace them with osteoclasts to produce osteocytes and a truly ossified tissue. With further vascular ingrowth, this zone remodels rapidly and becomes mature metaphyseal (lamellar) bone to form the diaphysis.
Diaphysis.
The main portion of the bone is the diaphysis. This is composed of mature cortical bone which, in children, has remodeling potential.
Vascular Supply.
Healthy bone growth requires normal vascularity of the physis. Because vessels do not traverse the cartilage cell layer of the physis, a strict separation exists between circulation to the epiphysis and metaphysis. Two patterns of blood flow to the epiphysis have been identified (28). Intracapsular epiphyses, such as the proximal femur and proximal radius among others, receive blood through vessels that enter around the base of the growth plate and span a narrow gap between the articular cartilage and the physis. Extracapsular physes are more richly vascularized by vessels that penetrate directly into the periosteum and the capsular attachments that surround them. Because of this, intracapsular epiphyses are more susceptible to vascular disruption from physeal fractures or osteoarticular infections. Serious growth disturbances, manifesting as articular surface deformity, angular deformation, or limb-length inequalities, may occur in younger children from these etiologies.
It must be also remembered that underlying the articular cartilage is a small area of endochondral ossification that contributes to the growth of the cartilage of the articular surface. Any injury to this area of endochondral ossification can result in the development of a defect in the articular surface.
Periosteum.
Another unique characteristic of growing bone is its thick, vascular, and highly osteogenic periosteum. The child’s periosteum is densely adherent to bone at some sites, notably along the linea aspera of the femur and at the periphery of the physes surrounding the perichondrial ring of Lacroix. With the exception of these sites, fracture displacement in children causes muscles and ligaments to separate from the outer fibrous layer of periosteum, leaving behind the inner osteogenic layer. Additionally, this thick periosteum is rarely torn circumferentially and frequently is left intact on the compression side of an injured bone. The partially intact periosteal sleeve limits fracture displacement to some degree and may be exploited by the surgeon to achieve and maintain fracture reduction. Periosteum, however, may also inhibit fracture reduction if a torn end becomes interposed between the fragments or if the bone ends button-hole through a longitudinal tear in the periosteum. Failure of closed reduction is sometimes the result of entrapment of the bone end outside the periosteal hole when reduction with longitudinal traction is attempted.
CLASSIFICATION OF CHILDREN’S FRACTURES
General Considerations.
Fractures to the immature skeleton, like in the adult, are described by location and fracture pattern. Many of these fracture patterns are similar to adult fractures, but others are unique to the growing child. Children’s bone is less mineralized, more porous, and has more vascular channels compared to adults. Because of its lower density, growing bone has a lower modulus of elasticity, diminishing its strength and response to bending forces but allowing it to absorb greater energy before failure. This mechanical property explains the occurrence of some of the fracture types unique to children. While children sustain complete fractures like adults, incomplete fracture types are more common in children compared to adults. As the child matures, fracture patterns sustained more closely resemble those of an adult.
Incomplete Fractures.
Incomplete fractures include stress fractures and insufficiency fractures in adults. A stress
fracture is an incomplete fracture that is caused by repetitive stress in a person with normal bone quality. Once thought to be uncommon in children, they are becoming more widely recognized, especially in adolescent athletes. Insufficiency fractures are incomplete fractures that occur in weakened bone as a result of minor trauma. In the adult, this is most often seen in the elderly with osteoporotic bone. In children, these fractures are seen in individuals with an underlying bone disorder, such as osteogenesis imperfecta, or in those who have osteoporosis from disuse, such as a nonambulatory child with cerebral palsy. Both stress and insufficiency fractures can progress to complete fractures.
fracture is an incomplete fracture that is caused by repetitive stress in a person with normal bone quality. Once thought to be uncommon in children, they are becoming more widely recognized, especially in adolescent athletes. Insufficiency fractures are incomplete fractures that occur in weakened bone as a result of minor trauma. In the adult, this is most often seen in the elderly with osteoporotic bone. In children, these fractures are seen in individuals with an underlying bone disorder, such as osteogenesis imperfecta, or in those who have osteoporosis from disuse, such as a nonambulatory child with cerebral palsy. Both stress and insufficiency fractures can progress to complete fractures.
![]() FIGURE 32-1. Plastic deformation (A), torus or buckle fractures (B) greenstick fractures (C), and complete fracture (D). |
Children can also have unique types of incomplete fractures, including plastic deformation, greenstick fractures, and torus or so-called buckle fractures (Fig. 32-1).
Plastic Deformation.
When a bending force is applied to a long bone in the immature individual, some of that force is first dissipated in the initial elastic deformity that occurs. More is lost in the plastic deformity that follows. Plastic deformation represents an internal microscopic mechanical failure of bone that results in both an angular and rotational distortion of long bones, seen predominantly in diaphyseal cortical bone. This occurs when the force applied is greater than the elastic limits of the bone but less than that which produces a failure of the internal structure (obvious fracture). Microscopically, there is a disruption of the junctions between osteon units. Thus the internal structure has undergone a permanent disruption that usually needs to be corrected. Clinically, minimal swelling is noted because of a limited fracture hematoma and periosteal stripping (31). Radiographs reveal angulation without an obvious fracture line.
Green Stick Fracture.
If the limit of plastic deformation is exceeded, gross failure of a portion of the osseous structure occurs. If it is a bending force, the failure occurs on the tension side of the diaphysis where the apex of the bending force occurs. Because of a number of factors, including the increased flexibility of the osseous structure and the dissipation of a great deal of the force with the initial elastic and plastic deformation, there may not be enough residual force to complete the failure of the entire osseous structure (30). This results in a typical incomplete greenstick fracture pattern in which there is a failure of the tension side and plastic deformation on the compressive or concave side.
The Buckle or Torus Fracture.
The metaphysis of long bones is composed mostly of cancellous bone surrounded by a thin layer of cortical bone. If a longitudinal force is applied along the axis of the extremity, this thin cortex will fail in compression producing the typical bulging of the “torus” or “buckle.” This compression failure occurs most commonly at the metaphyseal-diaphyseal junction, the site of transition from the dense cortical bone of the diaphysis to the more porous metaphyseal type. Torus fractures are typically not associated with soft-tissue swelling at the fracture site because the incompletely fractured cortex prevents the extravasation of blood into the deep soft tissues. While this pattern is most commonly seen in the distal radius, it can also occur in the metaphyses of the distal femur, proximal tibia, and proximal humerus.
Complete Fractures.
Complete fractures in children may be similar to adult fractures or involve the physis. Diaphyseal fracture patterns are directly related to their mechanisms of injury: torsional forces result in spiral fractures, a perpendicular moment force results in a transverse fracture, and longitudinal compressive forces result in oblique fractures. A combination of compressive and moment forces results in a higher energy “butterfly” fracture pattern. The last two fracture patterns, butterfly and oblique, may be length unstable (Fig. 32-2).
This description of fracture patterns, although basic, can guide treatment. The general principles of fracture reduction in order are: re-creation of the mechanism of injury to displace the interposing soft tissue (usually the infolded periosteum) followed by reversal of the mechanism of injury to realign the fracture, using the intact periosteum hinge to help stabilize the reduction. Spiral fractures, particularly those that are “greenstick” fractures, are reduced by de-rotating the fracture back to the reduced position. These fractures typically have an intact longitudinal band of periosteum that provides enough stability such that cast immobilization is successful in maintaining reduction in most cases (52). Transverse fractures have intact periosteum on the compression side of the fracture, which may interpose into the fracture site and interfere with reduction. These fracture types often can be simply reduced by following the principles of fracture reduction described above. Fracture reduction for transverse fractures is best maintained by three-point molding of the cast
around the fracture site. Butterfly and oblique fractures may be length unstable. Because of this, closed reduction and cast immobilization may not always be adequate. Maintenance of length in a cast is more difficult for these fractures compared to length-stable patterns, such as the transverse fracture. Fixation is sometimes necessary to maintain the length of butterfly and oblique fracture types.
around the fracture site. Butterfly and oblique fractures may be length unstable. Because of this, closed reduction and cast immobilization may not always be adequate. Maintenance of length in a cast is more difficult for these fractures compared to length-stable patterns, such as the transverse fracture. Fixation is sometimes necessary to maintain the length of butterfly and oblique fracture types.
Fracture patterns in children are different when compared to adults because of other anatomical and mechanical differences. Comminuted fractures are less common because pediatric bone more readily dissipates energy before failure and its porosity limits the propagation of fracture lines (30). The smaller diameter of a child’s bone also influences its strength. Because bone resistance to torque is proportional to the fourth power of its radius, even a small increase in cortical diameter with growth results in a large increase in the bone’s ability to resist torsional loading. The epiphysis, which is largely cartilaginous in younger children, transmits the energy of the injury to the metaphysis, explaining the increased frequency of metaphyseal fractures in this age group.
Physeal Fractures.
The immature skeleton is defined by an open physis, and the manipulation and preservation of the physis is the most unique, and challenging, feature of children’s fracture care. Fractures that extend through the physis or traverse the physis and its adjoining metaphysis or epiphysis are best described by the Salter-Harris classification (Fig. 32-3). This radiographic classification system is based on the location of the fracture with respect to the physis. It reliably describes physeal fractures and helps to guide treatment, but is less useful for assessing long-term prognosis.
Ham Peterson carefully reviewed physeal fracture classifications and developed a revised classification which may have better prognostic significance (53).
The physis has unique biomechanical properties that are determined by several factors. In studies of the physes of animals, the zone of provisional calcification is the weakest area when subjected to mechanical stress (33, 34). Physeal fractures in these studies occurred predominantly through this zone. Microfractures, however, were identified in other zones of the physis, including the germinal zone. In a study of human cadavers, forces applied across joints resulted in physeal fractures more frequently than ligament disruptions (35). Physeal fractures in children occur mostly through the zone of hypertrophying cells (36).
The biomechanical stability of the physis is not solely determined by the zone of provisional calcification. Physeal resistance to shear forces is improved by both its nonplanar topography and its reinforcing structures. Many physes are not flat but are instead undulating, as best seen in the distal femoral physis.
Smaller projections within the physis also have been identified and are called mamillary processes. Some physes overlap the edges of their adjacent metaphyses with cup-shaped contours called lappet formations, as in the proximal tibia. There are two major microscopic structures unique to the physis. The Perichondrial Groove of Ranvier is composed of both resting and proliferating cells, and the Perichondrial Ring of Lacroix, comprised of cartilage cells contiguous with the metaphyseal periosteum, surround the physes. In addition to contributing to circumferential bone growth, these structures reinforce the physeal periphery. Additionally, some muscular, capsular, and ligamentous attachments span the physis and contribute to its stability. With progression of skeletal maturity, the size of the epiphysis and its ossification increases while the perichondrial ring weakens, making the physis more vulnerable to failure. These changes with age are one of the explanations for the increasing occurrence of physeal fractures with increasing age in children.
Smaller projections within the physis also have been identified and are called mamillary processes. Some physes overlap the edges of their adjacent metaphyses with cup-shaped contours called lappet formations, as in the proximal tibia. There are two major microscopic structures unique to the physis. The Perichondrial Groove of Ranvier is composed of both resting and proliferating cells, and the Perichondrial Ring of Lacroix, comprised of cartilage cells contiguous with the metaphyseal periosteum, surround the physes. In addition to contributing to circumferential bone growth, these structures reinforce the physeal periphery. Additionally, some muscular, capsular, and ligamentous attachments span the physis and contribute to its stability. With progression of skeletal maturity, the size of the epiphysis and its ossification increases while the perichondrial ring weakens, making the physis more vulnerable to failure. These changes with age are one of the explanations for the increasing occurrence of physeal fractures with increasing age in children.
Salter-Harris Classification of Physeal Fractures
Salter-Harris Type I.
In this fracture pattern, the fracture line transverses the entire growth plate without extension into the metaphysis. Salter-Harris type I fractures commonly occur in infants and young children. The epiphyseal fragment may be nondisplaced or minimally displaced, making diagnosis difficult. The clinical exam is critical and usually consists of localized swelling and point tenderness at the physis.
Salter-Harris Type II.
Type II fractures are the most common fractures that involve the physis and represent nearly 75% of all physeal fractures. The fracture line passes through a portion of the growth plate and exits through a segment of the metaphysis that remains attached to the intact portion of the growth plate. This metaphyseal fragment is referred to as the Thurston-Holland fragment. It is typically on the compression side of the fracture (Fig. 32-4).
In Salter-Harris type I and II fractures, the fracture line usually does not violate the germinal matrix of the physis, which remains with the epiphysis, and therefore growth arrest, although possible, is uncommon. These fractures typically heal quickly with few long-term sequelae. Partial growth arrest may occur with more severe trauma, or when periosteum is entrapped in the physis (54, 55) and varies depending on the location of injury. For example, fractures of the distal radial physis rarely lead to physeal closure (56), but physeal fractures of the distal femur cause growth disturbance in up to 50% of those involved (57).
Salter-Harris Type III.
Type III fractures propagate vertically through a portion of the growth plate, then extend into the epiphysis, and exit across the articular surface.
Salter-Harris Type IV.
Type IV fractures cross all zones of
the physis vertically, extending from the metaphysis, across the physis, and through the epiphysis and its articular surface. Because the fracture line extends across the physis, injury to resting chondrocytes can occur. While only a small portion of the physis is breached in both type III and IV fractures, the risk of growth arrest is higher compared to other fracture types (58).
Salter-Harris type III and IV fractures require more exact reduction compared to type I and II fractures. Malreduction of the physis may lead to localized bone bridging at the fracture site, leading to angular deformities or limb-length discrepancies. Additionally, Salter-Harris type III and IV fractures are intraarticular fractures. Anatomic reduction of type III and IV fractures is indicated to lessen the risk
of growth disturbance caused by physeal bridging and to ensure that the joint surface is restored within 2 mm or less of step-off or gapping, diminishing the chances of premature joint arthritis.
of growth disturbance caused by physeal bridging and to ensure that the joint surface is restored within 2 mm or less of step-off or gapping, diminishing the chances of premature joint arthritis.
Salter-Harris Type V.
This injury is the result of a crushing across the physis by axially loading. This injury may be difficult to assess radiographically and often is not displaced. Growth arrest, typically identified at least 6 months after injury, allows the surgeon to retrospectively classify the original injury as a Salter-Harris type V fracture. This fracture is uncommon, and there is some controversy as to whether it actually occurs.
Salter-Harris Type VI.
This very rare type is a peripheral physeal injury at the level of the perichondrial ring (52). This may result from ligamentous avulsion, direct trauma, burn, or other forces. Localized growth arrest may occur and lead to asymmetric growth with angular deformity.
PHYSIOLOGY OF FRACTURE HEALING IN CHILDREN
Fractures in the skeletally immature have two factors that make their management easier than adults: rapid healing and a capacity to remodel. In addition, there may be both symmetrical and asymmetrical growth stimulation of the physis during the healing process.
Rapid Healing.
Fractures in children heal more rapidly and reliably than in adults. Nonunion is rare, especially after closed fractures. Increased bone vascularity, highly osteogenic periosteum, and limited soft-tissue disruption with most fractures are important factors (29). This improved ability to heal fractures influences fracture management. Cast immobilization is adequate for healing and maintenance of alignment for the vast majority of children’s fractures. Internal fixation, often needed to promote healing of adult fractures, is less frequently indicated for children and is mostly used to maintain adequate alignment for healing, acting as an “internal splint.” When fixation is necessary, less rigid implants that are easily removed and that do not violate growth plates, such as smooth wires and flexible intramedullary nails, are used in children compared to plate-screw constructs and rigid intramedullary devices typically employed for adults.
Remodeling Capacity.
Children have the capacity to remodel many fractures that heal with residual angulation (Fig. 32-5). Remodeling is a by-product of the growing bone’s increased vascularity, cellular periosteum, and cellular factors.
Remodeling likely occurs by two different mechanisms. Some remodeling is seen at the site of malunion by gradual resorption on the convexity of the deformity and increased deposition on its concavity, a phenomenon known as “bone drift” (37). In a rat model, analysis of callus on the concave and convex sides of remodeling deformities shows important immunohistochemical differences between these areas (38). Most remodeling, however, occurs through changes within the physis that cause asymmetric longitudinal growth and reorientation of the physis (37, 39).
Remodeling likely occurs by two different mechanisms. Some remodeling is seen at the site of malunion by gradual resorption on the convexity of the deformity and increased deposition on its concavity, a phenomenon known as “bone drift” (37). In a rat model, analysis of callus on the concave and convex sides of remodeling deformities shows important immunohistochemical differences between these areas (38). Most remodeling, however, occurs through changes within the physis that cause asymmetric longitudinal growth and reorientation of the physis (37, 39).
Three important factors affect the capacity of immature bone to remodel: (a) Remodeling capacity is best for fractures close to active growth plates. (b) Remodeling occurs most reliably after fractures that heal with residual angulation in the plane of motion of the nearest joint. (c) Remodeling does not occur to any significant degree in children with <2 years of skeletal growth remaining.
While restoration of anatomic alignment remains an important goal of children’s fracture management, some degree of nonanatomic reduction is acceptable in many types of children’s fractures because of the capacity for remodeling. Exceptions to this include fractures that cross the physis and fractures that extend into the articular surface, both of which require anatomic realignment. Remodeling may continue for 5 to 6 years after fracture healing (39). Severe residual angular deformities and rotational malunions remodel less predictably (37, 40). Because of the limitations of remodeling, the surgeon must be aware of the degree of residual angulation that is “acceptable” when making management decisions. This varies based on the child’s skeletal maturity, the particular bone that is angulated, the location and direction of angulation, and its degree of deformity. Limitations of space do not permit a complete discussion of the accepted limits of remodeling. A good discussion of this topic has been published by Kaye Wilkins (41).
Symmetrical Growth Stimulation (Overgrowth).
Symmetrical growth stimulation, or overgrowth, is another characteristic of fracture healing that is unique to children. While its etiology is not fully understood, loss of periosteal tension and increased blood flow to the physes of the fractured bone are potential causes. Experimentally, transverse sectioning of the periosteum, especially near a growth plate, reduces the tension within the periosteum and results in limb overgrowth. Incomplete transverse sectioning has been shown to cause asymmetric physeal stimulation, resulting in angular deformity (42). By contrast, longitudinal sectioning of the periosteum does not have the same effect (29, 43). Hyperemia is a plausible explanation based on the observation that limb overgrowth occurs in some childhood conditions associated with inflammation or increased vascularity such as inflammatory arthritides and congenital vascular
malformations (44). Clinically, overgrowth is most common after femoral shaft fractures in children between the ages of 2 and 10 years of age, averaging 0.9 cm with a range of 0.4 to 2.4 cm (45).
malformations (44). Clinically, overgrowth is most common after femoral shaft fractures in children between the ages of 2 and 10 years of age, averaging 0.9 cm with a range of 0.4 to 2.4 cm (45).
Asymmetrical Growth Stimulation.
Asymmetrical overgrowth is less common than symmetrical overgrowth. The case of the proximal tibia fracture may offer important insights into the etiology of remodeling and asymmetric bone stimulation after fracture. Incomplete fractures of the proximal tibial metaphysis in children sometimes result in an asymmetrical growth stimulation, typically valgus overgrowth of the tibia, within 1 to 2 years after the fracture. This deformity is likely the result of both asymmetrical hyperemia leading to overgrowth at the fracture site and asymmetric periosteal tethering of physeal growth on the contralateral side. Fortunately for most children, remodeling or reversal of the overgrowth occurs spontaneously, obviating the need for guided growth techniques or osteotomies to correct the overgrowth.
The etiology of this phenomenon of asymmetric overgrowth leading to proximal tibia valgus after an incomplete proximal tibia fracture is likely multifactorial and is not, however, completely understood (Fig. 32-6). Various theories have been proposed. Ogden (46) has demonstrated in cadaver studies that the blood flow to the medial side of the proximal tibial metaphysis is significantly greater than on the lateral side. Most of the data supporting the theory that intact periosteum inhibits longitudinal growth comes from animal studies. Early studies show that in chickens (47, 48) circumferential release of the periosteum resulted in overgrowth of the long bone affected. Subsequent studies in rabbits (49, 50) have shown that the release of only one side of the metaphyseal periosteum can produce asymmetrical growth stimulation. In none of the above animal studies were structural changes able to be demonstrated in histological examination of the physes.
THE INITIAL ASSESSMENT AND MANAGEMENT OF A CHILD WITH MUSCULOSKELETAL TRAUMA
Emergency Department Evaluation.
Most fractures in children are isolated injuries. Emergency department assessment of the injured child begins with the history. High-energy mechanisms of injury, multiple fractures, or other obvious nonskeletal injuries warrant a complete evaluation from the trauma team. For the isolated injury, the extremity is first inspected for swelling or deformity and the skin for abrasions or lacerations, soft-tissue defects, and exposed bone. The neurovascular status limb is determined by testing motor and
sensory function distal to the fracture site, palpation of distal pulses, and assessment of capillary refill. For grossly displaced or unstable fractures, application of gentle longitudinal traction and gross realignment may be done before further evaluation, especially for the limb with vascular compromise. Splinting of the grossly malaligned limb or unstable fracture immediately after evaluation before taking radiographs may be necessary to enhance the child’s comfort and to prevent further trauma. After obtaining high-quality orthogonal radiographic views of the fracture, including images of the joints proximal and distal to the injury site, preparation is made for fracture reduction.
sensory function distal to the fracture site, palpation of distal pulses, and assessment of capillary refill. For grossly displaced or unstable fractures, application of gentle longitudinal traction and gross realignment may be done before further evaluation, especially for the limb with vascular compromise. Splinting of the grossly malaligned limb or unstable fracture immediately after evaluation before taking radiographs may be necessary to enhance the child’s comfort and to prevent further trauma. After obtaining high-quality orthogonal radiographic views of the fracture, including images of the joints proximal and distal to the injury site, preparation is made for fracture reduction.
Pain Control.
For the majority of children’s fractures, analgesia must be provided prior to closed manipulation and casting or splinting in the emergency department. Numerous techniques may be used to provide pain relief and sedation for fracture reduction in the emergency department. These can be categorized into three broad categories: local and regional anesthesia, intravenous sedation, and dissociative anesthesia (59). For upper extremity fractures, local and regional techniques such as hematoma block, intravenous regional anesthesia (IVRA), and axillary block are effective. Intravenous sedation with a benzodiazepine-narcotic combination is not region specific and may be used for all ages. Ketamine sedation is extremely safe and effective, especially for children younger than 10 years of age. The choice of method is based on the needs of the surgeon and, more importantly, on the resources and experience of the emergency department staff. Currently, most children’s hospitals use ketamine sedation or other types of dissociative anesthesia for emergency department fracture reduction. Analgesia should only be performed by those with the proper training and experience with these techniques.
Local and Regional Anesthesia
Hematoma Block.
Local and regional blocks are useful for many different fracture types and are simplest to apply. In a survey of teaching hospitals in the North America, local anesthetic techniques are used less frequently than sedation to provide pain relief for forearm fractures in children (60). A hematoma block works by infiltration of the fracture hematoma with a local anesthetic agent, most commonly lidocaine, which inhibits the conduction of pain impulses in small unmyelinated nerve fibers in the periosteum and local tissues (61). It is used primarily for reduction of distal radius fractures. Because the technique relies on diffusion within the fracture hematoma, it is not effective for fractures with limited hematoma formation, such as greenstick fractures and often physeal fractures in which the physis serves as a barrier to the movement of the anesthetic into the fracture fragments. Fractures that present several days after injury are also not amenable to hematoma blockade because of hematoma coagulation that prevents agent diffusion.
The use of local anesthesia is the simplest method of achieving analgesia for fracture reduction in children. After sterile skin preparation, an 18- or 20-gauge needle attached to a 10-mL syringe filled with 1% to 2% lidocaine without epinephrine is inserted into the fracture hematoma. The hematoma is aspirated; blush of blood into the syringe confirms that the needle is in the hematoma. A total of 3 to 10 mL of 1% to 2% lidocaine (maximum dose of 3 to 5 mg/kg of body weight) is then injected into the fracture site. The process of barbotage, in which the anesthetic agent is mixed with the hematoma, is a potentially safer alternative (see Fig. 32-7). Injection volume must not exceed 10 mL at the wrist because excessive infiltration may result in increased carpal tunnel pressure and potential median nerve injury (62). After allowing several minutes for the agent to exert its effect, the surgeon may then proceed with fracture manipulation.
Intravenous Regional Anesthesia.
IVRA, also known as a Bier block, is utilized at approximately 20% of pediatric centers for reduction of forearm fractures in the emergency department (60). IVRA is used as an alternative to sedation and has been shown to provide satisfactory analgesia for reduction in 90% of cases (63, 64 and 65). Its advantages include ease of administration, rapid onset of analgesia, and a short duration of action, allowing neurovascular assessment immediately after reduction and potentially shortening the time spent in the emergency department. The Bier block acts by blockade of small sensory nerve branches by diffusion of a large volume of a dilute agent from the venous circulation (66). This method is most effective for treatment of forearm fractures and provides less reliable pain relief for manipulation of elbow fractures and fractures about the hand (67).
IVRA has an excellent safety profile. The most common adverse effect is tourniquet pain seen in fewer than 10% of patients. Use of two tourniquets that are alternately inflated reduces the incidence of arm pain but is not necessary for most routine fracture reductions. Toxicity from systemic release of lidocaine is the most serious complication of the Bier block and may result in cardiovascular complications, such as arrhythmias and hypotension, and seizures. To prevent this, the tourniquet should remain inflated for at least 20 minutes following the completion of the reduction before release of the tourniquet to allow tissue fixation of lidocaine, minimizing systemic exposure of the agent. A modified forearm IVRA that utilizes a forearm tourniquet in conjunction with an upper arm tourniquet, and a reduced lidocaine dose has been utilized successfully for treatment of distal radius and hand injuries in children. This method may reduce the risk of anesthetic toxicity (68).
Axillary Nerve Block.
Axillary (brachial plexus) block regional anesthesia is another technique utilized for the management of upper extremity fractures in children that provides pain relief equal to sedation (69, 70). This technique is commonly used in the operating room but has been infrequently utilized in the emergency department because personnel are less familiar with it and its application requires specialized instruction to learn the technique. Its rapid onset and low complication rate are some advantages compared to sedation. Its longer duration of analgesia (up to 4 hours) compared to IVRA makes it possible for the surgeon to perform repeat manipulations if necessary, without readministration of the block. Possible
complications include systemic lidocaine toxicity from intraarterial injection, local hematoma formation at the site of injection, and transient dysesthesias or brachial neuralgia.
complications include systemic lidocaine toxicity from intraarterial injection, local hematoma formation at the site of injection, and transient dysesthesias or brachial neuralgia.
![]() FIGURE 32-7. Barbotage technique. The local anesthetic is mixed with the hematoma by the process called barbotage. A: Half the anesthetic is injected into the hematoma. B: The blood from the hematoma is then withdrawn to equal the original volume. C: This material is then repeatedly injected and reaspirated until the anesthetic is completely mixed within the hematoma. It is important that the final aspirate equal that of the original volume so that the amount of fluid in the hematoma has not been increased. (Reproduced from Chapter 3, Sedation and analgesia in the child with a fracture In: Vol. III Rockwood CA Jr, Wilkins KE, Beaty JH, eds. Fractures in children. 4th ed. Philadelphia, PA: Lippincott-Raven, 1996:72-73, Fig. 3-8; with permission.) |
Conscious Sedation.
Conscious sedation is defined as a level of consciousness that maintains protective reflexes and retains the patient’s ability to maintain an airway independently. During conscious sedation, the patient can respond appropriately to verbal commands or physical stimulation. Deep sedation is defined as a more profound state of unconsciousness, accompanied by partial or complete loss of protective reflexes and inability to respond purposefully to verbal or physical stimuli. The American Academy of Pediatrics has developed specific guidelines for equipment and monitoring of all levels of pediatric sedation (71) to ensure safe emergence following its administration. While inhalational sedation by nitrous oxide has been shown to be safe (72), intravenous sedation is used more commonly. For either sedation technique, continuous monitoring of pulse rate and oxygen saturation is mandatory. Respiratory rate and blood pressure must be periodically monitored during administration and afterward until emergence to ensure safety. Conscious sedation should only be administered by skilled personnel with the proper emergency equipment to manage its potential complications, such as airway compromise or cardiovascular collapse. Deep sedation is utilized in the operating room only.
Benzodiazepines and narcotics are the most widely used agents for intravenous sedation. Benzodiazepines act primarily as sedatives, while narcotics provide pain relief. These drugs act synergistically to induce controlled sedation and analgesia. Numerous combinations of medications have been used successfully for the reduction of children’s fractures (73, 59). Midazolam and diazepam are the most commonly used benzodiazepines. Morphine, meperidine, and fentanyl are the most commonly used narcotics for intravenous management of pain. Complications of intravenous sedation include hypoxemia, apnea, hallucinations, pruritus, and emesis.
Dissociative Anesthesia.
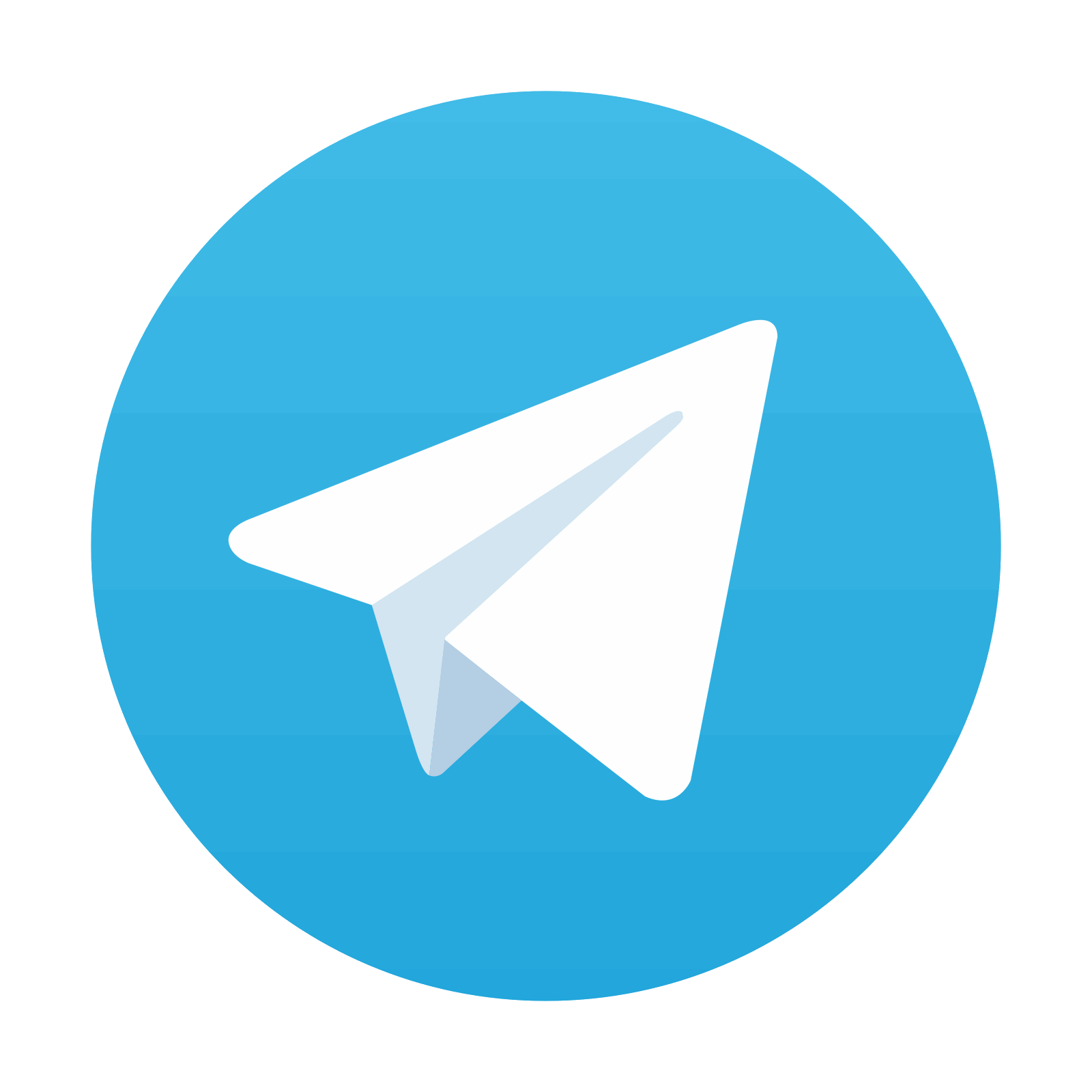
Stay updated, free articles. Join our Telegram channel

Full access? Get Clinical Tree
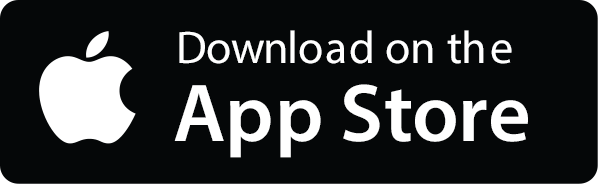
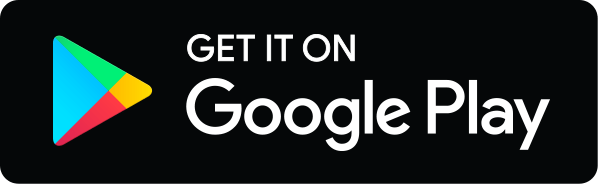
