1.2 The Nervous System and Motor Learning
Introduction
Therapists throughout the world are constantly asked to examine, evaluate, and intervene with individuals who present large variations in movement problems that affect their everyday activities and quality of life. These functional movement limitations generally arise from musculoskeletal, neuromuscular, cardiopulmonary, and integumentary problems, although organ, digestive, endocrine, and a variety of other systems may also interact and cause motor problems. Each system presents the therapist with different challenges, and many problems within each system can lead to pelvic floor dysfunction. No matter the disease or pathology that has led to the dysfunction, a large component of the movement itself is controlled and modified by the central nervous system (CNS) and its interaction with the way in which the muscles function within the pelvic floor. Conceptual models of the regulation and control of movement by the CNS have changed dramatically over the last few decades. In the 1960s, therapists were taught that movement control within the nervous system was developmental and hierarchically based, hard-wired, and functioned by feedback. Today, students learn that the motor output from the nervous system is based on a dynamic systems model in which there is distributed function, consensus, and a feedforward program that is soft-wired and constantly changing depending on practice, environmental demands, and internal restraints within the individual. Concepts and current research relevant to the regulation of movement by the central nervous system affect the way therapists analyze clinical problems, establishing the scientific rationale for selecting appropriate interventions; each physician therefore needs to ensure continuing education. Discussion of the CNS principles of motor learning, motor control, neuroplasticity, and the neural substrates underlying the way in which these concepts and principles apply to pelvic floor dysfunction is just one piece of a much larger puzzle relating to human performance and movement. The goal of this section is to provide an opportunity to identify these CNS variables and analyze how and why they may affect the outcome of an intervention.
Motor Control and Motor Learning
Before discussing the specific concepts behind motor control and motor learning, the basis for the general concepts used needs to be clearly identified. Motor control is the way in which the CNS controls motor performance, and the study of it developed from the sciences of neurophysiology and neuropsychology. Motor control measurements are made in units of milliseconds, and these continue to provide basic research data leading to a better understanding of the way in which the CNS controls behavior [Kandel et al. 2000, Shumway-Cook and Woollacott 2001]. Motor learning is not defined in millisecond responses, but rather as the ability of the CNS to retain movement programs and then transfer those programs into new environments.
Motor Control Theories
Early therapists developed models for motor control based on observation and palpation, and then went to the literature to develop theoretical frameworks [Rood 1962, Knott and Voss 1968, Ayers 1974, Bobath 1985, Brunnstrom 1992, Johnstone 1995]. Other therapists [Carr and Shepherd 1987] trained functional movement, because they believed it had greater carry-over into life activities. Although Bernstein [1967] had hypothesized a systems model for motor control decades earlier [Tuller et al. 1982], it was not until the 1980s that his theories became popular. Researchers began to develop and test new theoretical constructs that might explain variance in motor control as it relates to movement and posture. Although this and other models are widely accepted and used to explain motor control today, researchers are constantly hypothesizing and testing new explanations for the way in which people control motor output. Therapists concerned with the way in which movement and posture are controlled should be aware that research will continue to widen our understanding and change our perspectives on intervention. Today, researchers use a variety of tools and techniques to test hypotheses. Some researchers may direct their models to specific concepts of spinal motor generators and their effect on muscle performance, while others may select a more holistic model, studying the interactions between various areas of the brain and the spinal generators. Other research may focus on spinal lesions and functional activities, or hand function following hemiplegia, or the rate and trajectory of interlimb control in cerebellar dysfunction; however, there appears to be no research available that examines pelvic floor dysfunction in relation to specific theories of motor control. For this reason, this section will present current theories of motor control and extrapolate from them to the way in which motor control may influence both the striated and smooth muscles within the pelvic floor—whether functioning as a postural stabilizer or as an active mover during interaction with other muscles.
Contemporary Motor Control Theories
The central pattern generator (CPG) has become the basic spinal level unit when considered from the viewpoint of a systems model. In the past, this unit was regarded as a reflex and a stereotypical motor response. Today, CPGs are thought of as oscillators, because of their rhythmical activity [Kandel et al. 2000]. General CPGs control both flexor and extensor synergies and thereby control the entire limb. Other, more discrete CPGs appear to regulate pairs of antagonistic muscles. Once these CPGs or oscillators are combined or coupled within a limb, multijoint intralimb patterns develop. When the CPGs are coupled between limbs, interlimb coordination is produced, which allows for great variation in movement patterns. These combinations can lead to reciprocal interlimb patterns, such as gait, or cross-diagonal patterns, such as bilateral reaching. The way in which these CPGs couple with the pelvic floor muscles can only be speculated on, but the assumption that coupling occurs during all interlimb/trunk patterns is commonly accepted [Kandel et al. 2000].
In the past, therapists were taught that a single descending tract from a higher center within the brain controlled the voluntary response during movement. Similarly, postural control was considered as co-contraction of agonist and antagonist synergies. Today, it is known that there are multiple descending pathways that regulate posture and movement. There are three large descending systems: the ventral–medial, the lateral, and the corticospinal systems (Fig. 1.20) [Leonard 1998, Bear et al. 2001]. Each system is made up of a variety of descending tracts which serve different functions when modulating the CPGs. The ventral–medial system is primarily responsible for axial trunk activity and plays a key role in regulating antigravity postural activities, which require coactivating both agonist and antagonist simultaneously. It would appear that similar tracts during postural activities, or automatic responses such as a sneeze, would also regulate the pelvic floor as a support system in an antigravity environment. The lateral system is responsible for regulating axial/limb musculature. These activities often have a movement component. Again, the pelvic floor would seem to work in synergy with many movement activities during open-chain and closed-chain patterns such as walking, running, or jumping. The corticospinal tract is primarily responsible for fine motor coordination, especially in the distal muscles of the upper extremity. This last system was once thought to control volitional movement, but today it is known that volitional control is regulated through all three systems. The control may originate in the cerebral cortex, but depending on the movement or postural pattern to be regulated, different tracts will descend into the brainstem and then others onto the CPGs within the spinal system, in order to execute the desired response volitionally. Today’s theory and research would not support the concept that asking an individual to control a movement such as squeezing of a pelvic floor muscle volitionally means that the individual would transfer control of the synergistic responses of the CPGs. For this reason, the individual would be able to contract pelvic floor muscles actively, but not automatically respond as part of a synergic pattern, such as during a cough, or an intentional activity, such as running or jumping.
Another aspect involved in the regulation of motor control is information processing. Input to the central nervous system is sent to many areas simultaneously. In this way, the brain can optimize the information and distribute it to multiple areas. With multiple inputs from both internal and external sources going to multiple areas simultaneously, the brain can respond appropriately within the constraints of the situation (Fig. 1.21). For example, when an individual is anticipating a weight shift during a reaching pattern, such as picking up a shoe off the floor, specific synergistic patterns are required. By contrast, if the same individual is quickly perturbed when bumped, then a different response is needed. The way in which the nervous system distributes the information and determines the best motor response depends on the ability to process information. This process allows the individual to refine movement or postural patterns, or initiate compensatory patterns when the initial response is inaccurate [Pribram 1988].
Bernstein’s theory of motor control [Bernstein 1967, Tuller et al. 1982] identified that the brain needs to have a mechanism for reducing the degree of freedom that would exist if it triedto control each muscle and joint action separately. Bernstein considered that the numbers of degrees of freedom could be dramatically reduced if the muscles worked in synergy and if the syneries coupled the muscles and joints of a limb or inter-limb activity to produce functional patterns based on the context within which the pattern is needed. Functional synergies suggest that the muscles are activated in an appropriate sequence, force, timing, and direction, and that these variables are organized as a ratio. This relationship creates a scaling mechanism within which the nervous system can change a motor behavior depending on the specificity of the task and the environment within which the task is performed [Shumway-Cook and Woollacott 1995]. These functional synergies are flexible and soft-wired. Thus, they represent emergent properties that allow for learning and changing of existing programs. It would seem logical that the pelvic floor muscles would work best when controlled and modified as part of a synergy pattern that emerges within specific parameters. These properties would allow for control of the pelvic muscles in conjunction with other synergistic patterns during functional activities.
As was stated previously, information is processed and sent to multiple sites simultaneously. Similarly, within a single center such as the basal ganglia or cerebellum, information can be processed in multiple subsystems that may lead to different responses. This concept is referred to as reciprocity, and is defined as information flowing between two or more systems or subsystems simultaneously. Distributed function, on the other hand, suggests that one center can do more than one thing and will share that responsibility with additional areas. This redundancy may be a key to neuroplasticity and recovery after brain injury. In patients with disorders such as a stroke, Parkinson’s disease, etc., this type of redundancy may therefore also help improve the pelvic floor dysfunction if various systems and subsystems are challenged during the retraining process. A third concept, consensus, suggests that motor behavior will occur when a majority of brain centers have reached a set threshold to produce the desired response. It also provides the possibility that together the brain centers act as filter mechanisms for extraneous information and will dampen irrelevant data [Kandel et al. 2000, Newton 2001, Shumway-Cook and Woollacott 2001]. If an individual does not regain normal pelvic floor function after trauma, such as childbirth in women, the brain may adapt to the elongated position and not tighten the muscles into the shortened range during normal or stressful activities. This would lead to incontinence during such activities, but not necessarily to problems when the pelvic floor is not under stress.
Motor control is not a singular concept. Instead, it represents a variety of neural mechanisms. This control is a result of emergent properties. These properties arise from neural centers that work in cooperation, reach consensus, compare prior memories with current information, and select appropriate movement strategies that match with the appropriate force, direction, duration, and sequence of agonist and antagonist synergies in order to accomplish the desired task [Newton 2001]. To become part of these goal-specific activities, the pelvic floor muscles have to be incorporated into these emergent properties as part of the total synergistic activity needed in order to achieve control over the muscles during all functional activities. In summary, motor control theory as accepted today envisages a combination of synergic patterns, muscle linkage, and coordinated neurological structures.
Although the limbic emotional system is not considered to be part of the motor control system, therapists should know that it has a direct influence on CPGs within both the brainstem and the spinal cord. Patients with pelvic floor dysfunction often have strong emotional reactions. During the evaluation phase, therapists have to distinguish between patterns and tonal characteristics created by motor control deficits and by limbic emotional deficits, in order to design appropriate interventions. For example, a person who has been sexually abused may suffer from hypertonia out of fear and anxiety. It is also possible that such a person could suffer from hypotonia due to a lack of self-esteem, a lack of physical activity, and poor posture. On the other hand, a competitive runner may suffer from hypertonia in the pelvic floor due to intense training requirements and an inability to go to the bathroom at regular intervals. Thirdly, a person with stroke may have hypertonic pelvic floor muscles due to the neurological injury.
Although the limbic system is often associated with emotions and declarative memory [Holstege 1991, Holstege et al. 1996], it can also be regarded as a second motor system, due to its direct and indirect effect on motor performance. The limbic system is the emotional driving component of all intentional movement. An analogy for the brain’s three subsystems (cognition, emotion, and motor) might be the way in which a car runs. When an individual recognizes the key and where it fits into the ignition, it is synonymous with the use of cognitive and perceptual subsystems. The engine functions as the cerebellum, while the starter motor would be analogous to the basal ganglia. The limbic system links cognition with the motor by driving the intention of the action. It is the limbic system that decides whether or not to turn the key on. No matter how perceptually or cognitively intact an individual may be or how functional the motor system is, neither can express itself in an integrated fashion in the absence of a drive to link the two. In other words, without the drive to turn on the ignition switch, the car will never run and the engine will remain dysfunctional. Thus, the limbic system has the potential to modulate motor system responses depending on the emotional context of the environment—whether cognitively driven, as in intentional motor control, or hypothalamically driven, as in automatic smooth-muscle function. The stronger the emotional link, the harder the individual will find it to control motor responses and reach consensus among all of the systems when integrating a function into complex synergic motor programming. Patients often have strong emotional reactions to a lack of bowel or bladder control, whether it is due to hypotonicity or hypertonicity. These emotional reactions make it difficult to modulate the CPGs and to control motor function. Thus, a potential key to regaining motor function would be to help the individual to relax by taking deep breaths, disengage the functional activity from a learned emotional response, and run motor programs that include the pelvic floor within the context. Once the programs are running as a synergic pattern, the patient’s attention needs to be focused specifically on the activity or task and not on the pelvic floor muscles themselves. Similarly, the patient needs to bring in emotional memories and feelings while the motor system is running the activity. In this way, the program can become integrated as a procedural program, and the CNS will develop an ability to refine the program no matter what the emotional context is [Umphred 2001] (see section 1.1 for additional information on the limbic system’s influence on somatic and autonomic motor systems.) Cognitive, limbic, and motor functions are conceptually separate and are located in different structures in the brain. However, in the context of motor responses, it is a consensus between all of these centers that ultimately drives motor output.
Motor Learning
Motor learning is defined as the ability of the human nervous system to learn and modify motor programs [Shumway-Cook and Woollacott 1995, 2001].
The difference between motor control and motor learning has to be understood in order to distinguish between these two conceptual areas in therapy. Motor control is the study of the way in which humans control movement that has already been acquired. Motor learning is the study of how humans acquire, modify, and retain these memory patterns so that they can be reused. The acquisition or modification of a movement, or both, can be in relation to initial learning (e. g., toilet training a child) or in relation to repeated acquisition following an injury (e. g., re-learning bowel and bladder control following a stroke or head injury). Four principles of motor learning can be identified that play a crucial role in the therapeutic decision-making process [Shumway-Cook and Woollacott 1995]:
- Learning is a process of acquiring the capability for skilled action
- Learning results from experience or practice
- Learning cannot be measured directly—instead, it is inferred on the basis of behavior
- Learning produces relatively permanent changes in behavior, so that short-term alterations should not be thought of as motor learning.
Motor learning is a complex process reflecting the response of the nervous system to a task-specific activity that emerges from an interaction between the need to perform the task and the environment within which the task is being performed [Candia et al. 2003, Nudo 2003, Ward et al. 2003].
Various factors that affect motor learning need to be considered. Some factors are within the cognitive and affective areas [Das et al. 2001, Jackson et al. 2001, Sapolsky 2003, Hashino 2004], while others fall specifically within the motor system. Factors that arise outside the motor system are cognitively and emotionally based, but still express themselves through motor behavior. Central nervous system behaviors such as arousal, attention, and motivation are controlled by areas not considered to be part of the motor system [Kandel et al. 2000], but ultimately affect an individual’s long-term motor performance. The long-term memory used by the cognitive and emotional systems is referred to as declarative memory. Declarative memory takes cognitive attention and requires intact limbic/emotional centers to store in long-term memory. If a patient’s problems have a highly emotional component [Sapolsky 2003], then using cognition to create motor control may not be effective in achieving storage in long-term memory. This certainly needs to be considered when teaching a patient how to regain pelvic floor control following disease or trauma.
Memories that are developed and driven by the motor system itself are called procedural memories. These memories become motor programs that can run automatically in a feedforward process as part of the nervous system’s response to environmentally specific tasks. This feedforward mechanism is similar to turning on a videotape and running a movie. The motor system will adjust the fine turning of the program via feedback [Kandel et al. 2000, Kuo 2002], but will not change the feedforward mechanism unless the program is no longer appropriate to meet the environmental task-specific demands. When pelvic floor movement activities are taught and practiced using total cortical attention, a therapist should not expect that the movements will automatically become part of a total body pattern or a feedforward procedural motor program. To help the pelvic floor muscles become part of a procedural motor program or part of a central pattern generator’s response to a specific environmental demand, the muscles need to be incorporated into total body movement patterns that relate to a task-specific activity [Yahagi et al. 2003]. In this way, the pelvic floor musculature becomes part of a synergic program.
Example
A person who suffers from leakage of urine during coughing and sneezing can learn to tighten the pelvic floor muscles when taking the deep breath preceding the cough or sneeze, and to extend rather than flex the spine during the sneeze or cough.
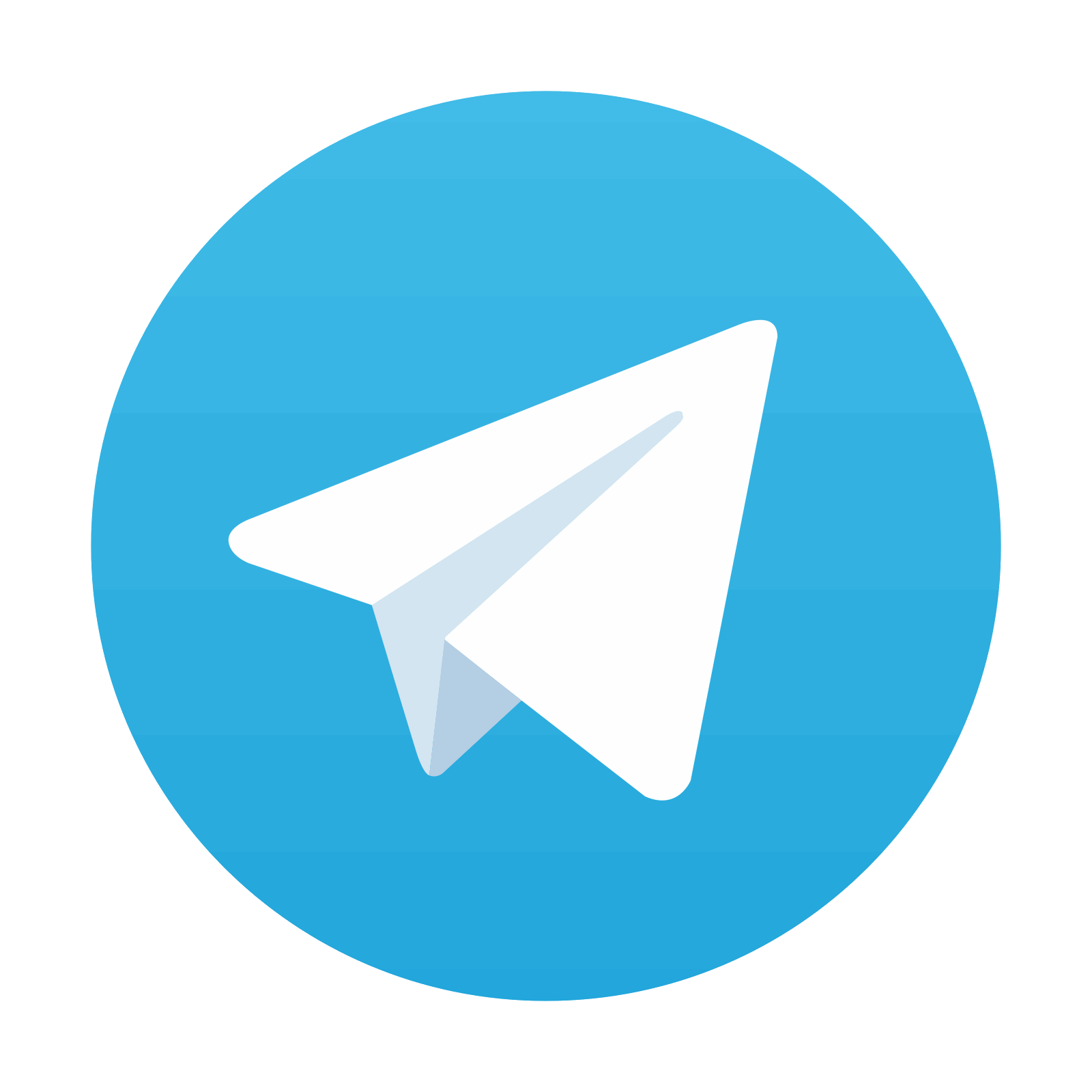
Stay updated, free articles. Join our Telegram channel

Full access? Get Clinical Tree
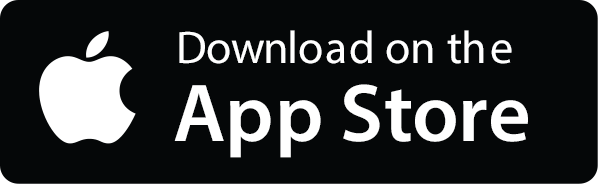
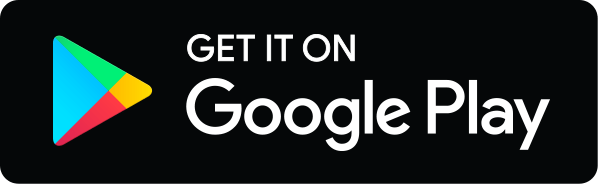