Chapter 8 The Genetics of Lupus
GENETIC EPIDEMIOLOGY
Familial aggregation of SLE has been consistently observed in many studies, with a sibling recurrence risk ratio (λs) of 20 to 40 (depending on the population studied).1,2 The λs is a statistic that measures the ratio of the phenotype frequency in siblings of affected probands compared to the phenotype frequency in the general population and is a crude measure of the potential a phenotype has for a genetic explanation.3 As a comparison, the λs for a monogenic trait such as cystic fibrosis is about 10-fold higher (λs=500). The higher the λs the easier it is to identify the gene(s) responsible for disease.
Among twin studies, an increased concordance rate among monozygotic twins (24 to 69%) is almost 10 times higher than that seen in dizygotic twins (2 to 9%), providing further support for a genetic contribution to SLE.4,5 The lack of a 100% penetrance among monozygotic twins, however, demonstrates that environmental factors, genetic imprinting, X-chromosome inactivation, or random processes may also play a role in the disease.6 Grennan et al. found that 25% of monozygotic twins were concordant for SLE. However, none of 18 HLA identical same-sex siblings of SLE probands had definite SLE—suggesting that most of the genetic predisposition to SLE is attributable to genes outside the HLA region.7
Except for isolated SLE cases with complement deficiency, the pattern of inheritance in SLE does not seem to follow a simple Mendelian trait such as autosomal dominant, autosomal recessive, or sex-linked recessive. However, dominant models have been shown to fit well among multi-case SLE families.8,9 In one study, a segregation analysis applied to 19 multi-case families for SLE demonstrated a Mendelian dominant inheritance pattern (with penetrance of 92% in females and 49% in males) and a gene frequency of 0.10.8 In a separate study, Winchester and Nunez-Roldan calculated that a polygenic model with at least three or four dominant alleles fit best among their SLE families.9
GENOMIC METHODS
Association and linkage studies are the two major approaches to genetic dissection of complex traits.10 Association studies are performed on both single cases (in case-control studies) and family-based studies. In the population-based case-control association design, differences in relative allele or genotype frequencies at a single marker locus are evaluated between unrelated affecteds and healthy controls. To avoid population stratification, which may cause spurious associations in such studies, family-based association tests have been developed in which the transmission of marker alleles from heterozygous parents to affected and unaffected offspring are compared in small nuclear families.11
Transmission from generation to generation occurs in sets of linked loci called haplotypes. The measure of this linkage effect in the population (linkage disequilibrium, LD) differs substantially from population to population and decays gradually due to recombination and mutation. Genome-wide linkage disequilibrium mapping has been recently applied to detect disease genes.12,13 However, the extent of LD in the human genome is not completely characterized. Estimates of up to 500,000 single-nucleotide polymorphism (SNP) markers may be needed to capture most of the information.12 With rapid advancements in genotyping technology, genome-wide association scans using hundreds of markers are underway and are expected to become standard in the near future. In such studies, however, large numbers of association tests are computed requiring correction of statistical significance for multiple testing.
SLE has substantial intrafamilial subphenotype variability among siblings. The classification for study typically requires the patient expressing 4 of 11 American College of Rheumatology (ACR) criteria.14,15 This, in turn, further increases genetic heterogeneity. Many epidemiologic and environmental elements such as ethnicity, gender, hormonal exposure, UV radiation, pregnancy, smoking habits, and viral exposures are thought to influence these phenotypic variations. The interpretation of these statistical analyses is especially complicated. Overall, association studies seem to be more powerful than linkage for complex diseases.13 Association studies of the entire genome depend on a dense map of markers. They are unlikely to detect extremely rare variants. Currently, most of the published association lupus results are based on case-control association studies, which are subject to artifacts derived from phenotypic variation and population stratifications. Of the many purported associations published in lupus, studies confirming the initial results have been performed in only a minority of cases. As has proven typical for virtually all human phenotypes, many purported genetic associations in SLE have not been confirmed. Consequently, a complex literature has been emerging.
THE HLA REGION
In 1999, the human MHC Sequencing Consortium annotated 224 genes in this 3.6-Mb genomic segment.16 Today, there are 239 genes in the region.17 The presentation of antigens to T-cells is the important known function of the products of MHC genes, but the functions of many other genes in this region have yet to be characterized. There are three class I alpha-chain genes (HLA -A, -B, and -C) and three pairs of highly polymorphic MHC class II alpha and beta-chain genes (HLA-DR, DP, and DQ). HLA-DR, however, may have an extra beta-chain gene whose product could pair with the DR alpha chain. Hence, the three sets of genes could give rise to four types of MHC class II molecules. The genes encoding the alpha chains of MHC class I and the alpha and beta chains of MHC class II molecules are linked within the complex. There are more than 200 alleles of human MHC class I and II, and therefore most individuals are likely to be heterozygous at MHC loci with co-dominant expression of both alleles. The MHC class III region contains genes that encode complement component C4 (C4A and C4B), C2, and factor B (Bf gene); some that encode cytokines such as tumor necrosis factor-alpha (TNF-alpha, TNF gene); and some that encode lymphotoxins (LTA and LTB).
The development of many autoimmune diseases has been etiologically linked to exposure to infectious agents. Several studies support molecular mimicry as a mechanism for the involvement of class II epitopes in infectious disease-induced self-reactivity. Many suggest that selective evolutionary pressure from infectious agents maintains a wide variety of MHC molecules in the population.18,19 For example, the HLA-B53 allele has strong association with recovery from a lethal form of malaria. This allele is very common in West Africa, where malaria is endemic.
The DR-B1 alleles DR2 and DR3 have shown consistent associations with SLE in Caucasian populations, with a two- to threefold increase in frequency.20–22 However, HLA associations in many non-Caucasian populations have not been very convincing. This could be due to different levels of admixture in African-American populations, and therefore matching the ethnic ancestry of the cases and controls is important and challenging. Despite many case-control studies, few studies have applied family-based association in the HLA region,23,24 and in European-Americans only DRB1*1501 (DR2) seems to be associated in two different family-based populations (p=0.0007, p<0.05).23,24 This is an example of association studies showing different results with HLA-DR3 and DR2. The association with HLA-DR2 can be localized to this allele, whereas the association with DR3 cannot be reduced to less than an extended haplotype containing HLA-DR3 and many other genes.23
Because antigen recognition by T-cells is dependent on the presence of specific MHC molecules, HLA class II alleles may show stronger association with the autoantibody profiles observed in SLE than with disease expression or particular clinical manifestations. In fact, several studies suggest that the contribution of HLA class II genes in SLE is predominantly at the level of production of specific autoantibodies rather than with SLE itself.25–27 There are a number of studies that have found association of HLA alleles with various autoantibodies. These include a study of HLA DR3/DR2 with anti-Ro,26 which identifies haplotypes at HLA-DQ (which also appears to be associated with anti-Ro through gene complementation and perhaps with polymorphism of the T-cell receptors).28,29
COMPLEMENT COMPONENTS
Complement cascade plasma proteins, key components of the innate immune system, can be activated by three distinct pathways: classical, alternative, and lectin. Complements have important roles in host resistance to bacterial infection and in the clearance of immune complexes. Therefore, they prevent autoimmunity. In addition, complements have an important role in lymph node organization, B-cell maturation, differentiation and tolerance, and IgG isotype switching.30,31
C2, C4A, C4B, and factor B are complement components located in the MHC class III region. Different alleles of these three components are linked to particular HLA haplotypes and are inherited as extended MHC haplotypes or complotypes. Considerable differences in complotype frequencies have been observed among various SLE ethnic groups. Complement deficiency is usually inheritied as a recessive trait except for C1 inhibitor, CR1, and properdin deficiency (which are autosomal dominant, codominant, and X linked, respectively). In Europeans, homozygous C4 or C2 deficiency is found in up to 1 in 10,000.32,33 However, C2 deficiency has not been identified in the African-American population. More than 35 variants have been observed in the human C4A and C4B loci, including null C4 variants (C4AQ0, C4BQ0) that have arisen due to allelic deletion. Heterozygous deficiency of C4 probably is the most common form of complement deficiency among lupus families.34 However, there is no correlation between the number of C4 genes expressed and C4 concentration. Indeed, the increase in C4AQ0 in SLE patients could be due to other genes on the extended haplotype of HLA-B8;SC01;DR3.35
C2 deficiency in Caucasians is linked to another HLA region extended haplotype: HLA-A25,B18,DR2,DQB1.36 Associations of anticardiolipin antibodies with C4A or C4B null allotypes in the U.S. black population,37 or anti-Ro with homozygous C2 deficiency in Caucasians,38 could be due to these MHC extended haplotypes. In fact, mild alterations of serum complement levels in heterozygous C2 and C4 deficiency patients suggest that the primary factors for inducing lupus in these patients may be beyond serum complement activity. Homozygous C2 and C4 deficient patients usually have a mild disease with a low titer of anti-nuclear antibodies. In contrast, C1q deficiency (although much less frequent) has a higher predictive risk for lupus (>90%) and is associated with more severe disease and glomerulonephritis. About 20 families with C1 (C1q, C1r, C1s) deficiencies have been described in the literature, and heterozygous deficiencies are difficult to identify. Complement components C3,C5 through C9 deficiencies (which are usually associated with infection) have also been reported with SLE.
The CR1 complement receptor (C3b/C4b receptor) is expressed on erythrocytes and is the structural basis of the Knops blood group. Its genetic control is under the influence of two alleles with codominant expression, which is based on the varying number of long homologous repeats (LHR).39 Erythrocyte CR1 is crucial for buffering immune complexes in circulation and transporting them to phagocyte Fc gamma and complement receptors located in the liver and spleen. Therefore, the number of functional erythrocytes expressing CR1 is important in this process. The severe anemia of malaria and hemolytic anemia influences CR1 expression, as does the serum erythropoietin level. In fact, this is reason to use recombinant erythropoietin in SLE patients.40 Although low levels of CR1 expression in SLE have been shown in different studies, the deficiency is at least in part reversible (and inherited CR1 deficiency does not seem to clearly predispose to SLE).41 Both functional and structural polymorphisms of CR1 have been reported with different results among lupus patients. A recent meta-analysis of these polymorphisms among 18 studies suggests an association of CR1-B (also called S allele) and SLE in European-Americans (OR=1.66).42 However, the CR1-C allele (which in theory may better explain the lack of clearance of immune complexes in SLE) was not associated.42 In fact, only one study suggests an association of the CR1-C allele with lupus.43
Another structurally related complement receptor, CR2 (CD21), is expressed only on lymphocytes and dendritic cells and has been implicated in lupus susceptibility in both human and animal models. Moreover, it serves as the receptor for Epstein-Barr virus. Although some variations in the CR2 gene due to alternative splicing have been detected, in contrast to CR1, a specific LHR variation in human CR2 has not been identified44 and may be the reason for a lack of association with this gene (which is suspected to be important in SLE).
FCγ RECEPTORS
The low-affinity Fcγ receptor (FcγR) genes are attractive candidates for SLE disease susceptibility. Numerous studies have demonstrated association with one or more FcγR alleles and SLE. Three distinct but closely related classes of FcγR have been identified in humans: FcγRI (CD64), FcγRII (CD32), and FcγRIII (CD16). FcγRs vary in their binding capacity for IgG, their preference for IgG subclasses, the cell types in which they are expressed, and the intracellular signals they elicit. They may be stimulatory (FcγRIIA, FcγRIIIA, FcγRIIIB, FcγRIIC) or inhibitory (FcγRIIB) to immune responses.45
Normal individuals with an HLA haplotype containing DR2 or DR3, both of which are found with increased frequency in SLE patients, are more likely to have prolonged Fc receptor-mediated clearance of IgG than normal control groups without these haplotypes.46 There are also allelic variations of FcγRIIA and FcγRIIIA that influence the ability to bind certain IgG subclasses and alter the responses of phagocytes to IgG-opsonized antigens.47,48
A meta-analysis comprising more than 1000 patients established an association with FcγRIIA-R131 and SLE, especially in African-American populations, and with FcγRIIIA-F176 and SLE in European-Americans and other ethnic groups.49 In addition, a potential dose-response relationship between the FcγRIIA-R131 allele and the risk of SLE was also identified, demonstrating a greater odds of having SLE if a patient had the 131R/R genotype compared to the 131R/H genotype (OR=1.23, 95% CI 1.03-1.46) or 131H/H genotype (OR=1.55, 95% CI 1.21-1.98).50
The FcγRIIIA polymorphism has an impact on the development of lupus nephritis. A comparison of 1154 lupus nephritis patients with 1261 non-nephritis SLE subjects revealed a significant overrepresentation of the low-binding F158 allele among patients who developed renal disease (OR=1.20, 95% CI=1.06 to 1.36, p=0.003).51 The 176F/F genotype had the highest risk of renal disease when compared to the 176V/V genotype (OR 1.47, 95% CI=1.11 to 1.93, p=0.006).51
FcγR genes are in a cluster of ∼300 kb on chromosome 1q23. However, the potential role of linkage disequilibrium between the FcγR genes is not yet established. In a cohort of 46 Hispanic SLE patients with a high prevalence of lupus nephritis in which there was a selection for haplotypes containing FcγRIIA-R131 and FcγRIIIA-F176, no LD was detected between FcγRIIA and FcγRIIIA (35 Kb separation).52 In a study of Japanese SLE patients, significant LD was detected, however, between FcγRIIIA and FcγRIIIB but not between FcγRIIA and FcγRIIIA (nor between FcγRIIA and FcγRIIIB).53
Associations of SLE with other Fcγ receptors have also been reported. At least two studies have shown an association between FcγIIB-232T and SLE in Asians.54,55 FcγIIB is the only gene among the FcγR family that contains an immunoreceptor tyrosine-based inhibitory motif (ITIM) and has the ability to transmit inhibitory signals in B-cells and myelomonocytic cells. In fact, in one study of SLE in a Thai population an association of FcγRIIIA-176F was identified, but this may have been attributable to its strong LD with FcγRIIB-232T and/or FcγRIIIB-NA2.56 Alternatively, in a European-derived sample, association of SLE with FcγRIIB has been identified in a variant of the 2B.4 promoter haplotype.57 This study also demonstrated a lack of LD between the FcγRIIB promoter haplotypes with FcγRIIA and FcγRIIIA polymorphisms in European-Americans. These variable LD and association results obtained in the different studies could be due to random variation, admixture effects, phenotypic variation, lack of appropriate controls and population stratification, or the confounding influence of other susceptibility loci.
TUMOR NECROSIS FACTORS
The increased expression of the tumor necrosis factor (TNF) alpha, a proinflammatory cytokine in MHC class III region, has been correlated with a variety of different autoimmune and infectious disorders. The −308 polymorphism is located in the promoter region. It contains two alleles (TNF1 and TNF2) that regulate low or high expression. TNF2 (-308 A) has been associated with SLE either independently of HLA haplotypes (OR=5 in Caucasians58 and OR=2.72 in African-Americans59) or as a part of an extended MHC haplotype (HLA-A1-B8-DRB1*0301-DQ2).60 In fact, the combined HLA-DR3, TNF-alpha −308A, IL-1alpha −889C/C genotype produced an OR = 8.0 (p < 0.00001) in a Caucasian population.61 The TNF-alpha −308 A polymorphic association is related to autoimmunity in general rather than specific SLE, and there were also some negative results in lupus that could be due to random variation or population heterogeneity.62,63 Interestingly, because TNF2 seems to be a common susceptibility allele for various autoimmune rheumatic diseases and at the same time has a protective role for tuberculosis, it has recently been suggested that autoimmune diseases might be a consequence of natural selection for enhanced TB resistance.64 Polymorphism of other related genes in this family [such as TNF-B or TNF-R2 (p75), FAS, and FAS ligand] have also been considered as risk factors for lupus with various results.
MANNOSE-BINDING LECTIN (MBL)
The lectin pathway is responsible for an antibody-independent pathway of complement activation that is initiated by binding of the mannose-binding lectin (MBL) to carbohydrates on the surface of pathogens. MBL activates complement through associated serine proteases. MBL deficiency is not uncommon in the general population (5 to 10%) and is associated with increased susceptibility to infection as well as enhanced progression to SLE and severe rheumatoid arthritis.65,66 According to a meta-analysis of more than eight published studies and two additional unpublished samples, MBL variant alleles confer a 1.6 times overall increased risk for SLE (67,68). In general, MBL variants have been considered a severity marker rather than a susceptibility marker for SLE.
PDCD1, CTLA-4
The CD28-B7 and immune co-stimulatory molecule ICOS pathways promote T-cell activation, whereas the cytotoxic T lymphocyte antigen-4 (CTLA-4) and PD-1 pathways lead to down-regulation of T-cell activity. PD-1 belongs to the CD28-B7 family that contains an immunoreceptor tyrosin-based inhibitory motif (ITIM) and serves as a negative regulator of immune responses. C57BL/6 knockout mice for PD-1 have been shown to develop lupus-like nephritis and arthritis.69 The PD1.3A intronic SNP has been associated with human SLE in both Europeans and Mexicans.70 It appears that this SNP alters a binding site for the runt-related transcription factor 1 (RUNX-1), which could lead to aberrant regulation of PD-1. Additional reports suggest that SNPs affecting RUNX1 binding site are associated with rheumatoid arthritis as well as psoriasis.71,72 Interestingly, PD-1 originally became the focus of interest after a linkage effect was identified at 2q37 in Northern European lupus families.73 However, it is not completely clear whether this intronic SNP is responsible for the entire linkage observed.
Like the PD-1 pathway, the cytotoxic T lymphocyte antigen-4 (CTLA-4) B7 pathway leads to the down-regulation of T-cell activity. It is possibly the most robust regulatory process controlling autoreactivity, which is illustrated by severe lymphoproliferative disorders with early lethality in CTLA-4-deficient mice.74,75 Many autoimmune disorders including SLE have been associated with variants of this gene, but the results have not been consistent. However, two recent meta-analyses for the exon 1 (A/G) polymorphism suggestively confirm the implication of this gene in SLE with an OR=1.24 for the G allele in an Asian population76 and OR=1.39 for the GG genotype when assuming all studies come from the same population.77
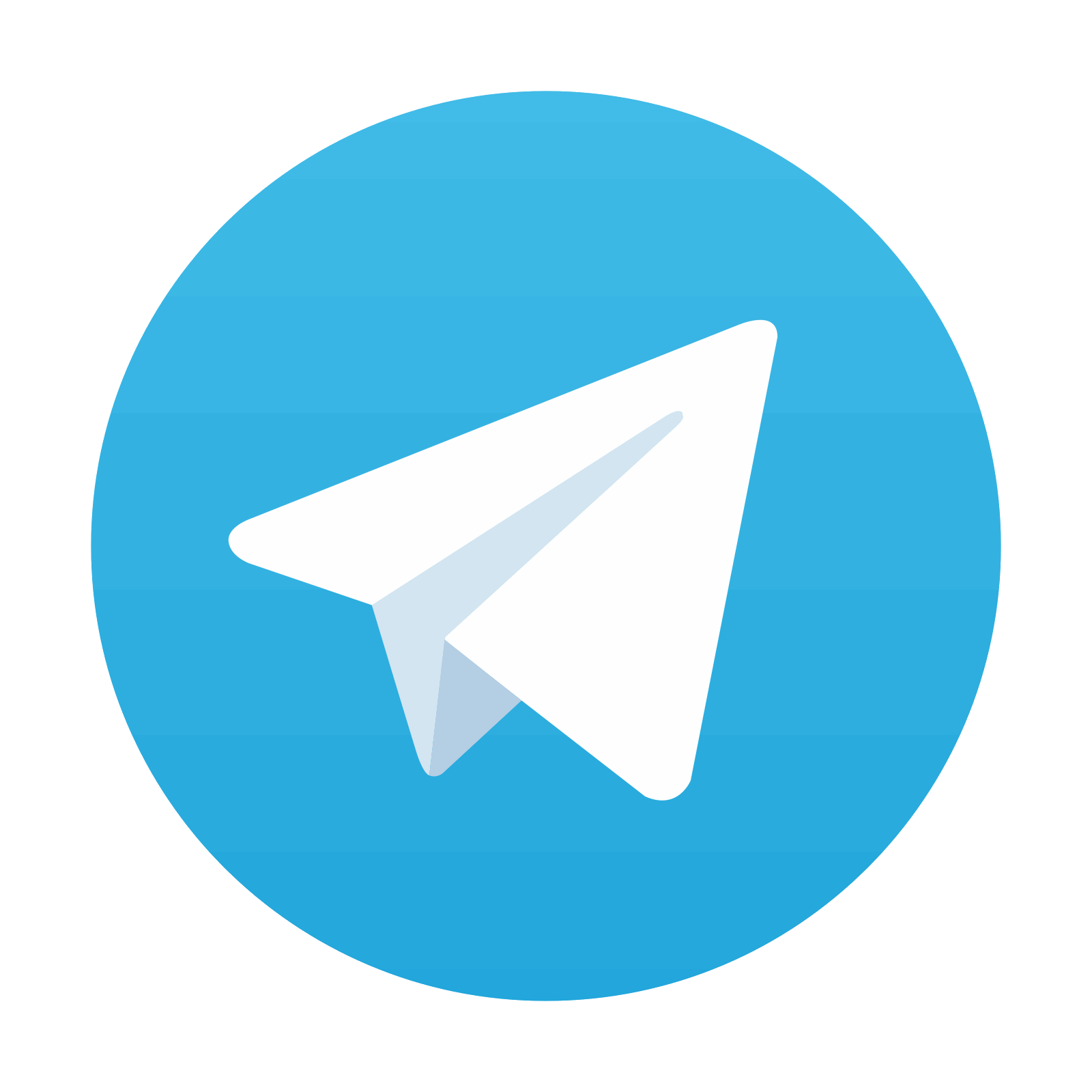