The Elbow
Simon Blease
David W. Stoller
Marc R. Safran
Arthur E. Li
Russell C. Fritz
Magnetic resonance (MR) imaging is now well established as a key imaging tool in the assessment of the elbow. Superior depiction of muscles, ligaments, and tendons and the ability to directly visualize nerves, bone marrow, and hyaline cartilage are advantages of MR imaging relative to conventional imaging techniques. The origin of elbow pain may be complex, and the ability of MR to accurately depict soft-tissue pathology as well as osseous damage is critical to its usefulness. Ongoing improvements in magnet technology and surface coil design and newer pulse sequences have resulted in faster acquisition and higher-resolution images. Clinical experience has confirmed the utility of MR imaging in detecting and characterizing disorders of the elbow in a noninvasive fashion.1,2,3,4,5,6,7,8
The elbow is a complex joint, but unlike the shoulder or knee the complexity arises from highly adapted bony architecture rather than sophisticated soft-tissue support. Although arthroscopy has a well-defined role, arthroscopic studies have not added significantly to the understanding of the anatomy and pathology of the elbow.
As in the knee, many problems in the elbow arise from increased varus and valgus stress delivered in acute or, more commonly, chronic situations. Conditions amenable to characterization on MR imaging include:
The sequelae of medial traction and lateral compression from valgus stress, including:
Medial collateral ligament (MCL) injury
Common flexor tendon pathology
Medial traction spurs
Ulnar neuropathy
Osteochondritis dissecans
Lateral collateral ligament injury
Lateral epicondylitis
Posttraumatic osseous abnormalities such as:
Radiographically occult fractures
Stress fractures
Bone contusions
Apophyseal avulsions
Cartilaginous extension of fractures in children (a condition difficult to evaluate with computed tomography [CT])
Intra-articular loose bodies and capsular pathology, especially if fluid or contrast material is present within the elbow joint
Biceps and triceps tendon injuries
Entrapment neuropathies
Bursitis
Arthropathies
Soft-tissue masses about the elbow
Imaging Techniques and Protocols for the Elbow
Pearls and Pitfalls
Techniques and Protocols
It is important to locate the joint as close to isocenter as possible either using a prone position or an open magnet.
Axial images must include the radial tuberosity.
Sagittal images should have a larger field of view to evaluate a possibly retracted biceps tendon.
Extremity Coils and Patient Positioning
There are a variety of technical challenges to obtaining optimal images of the elbow. It is an off-center structure, which makes it difficult to perform isocenter imaging. In a conventional magnet, the elbow is scanned with the patient in a supine position with the arm at the side. Injuries commonly produce a fixed flexion deformity (due to painful limitation of motion or structural block), however, making positioning the patient in a conventional supine posture difficult. A surface coil is essential for obtaining high-resolution images. Depending on the size of the patient and the size of the surface coil relative to bore of the magnet, it may be necessary to scan the patient in a prone position with the arm extended overhead (the so-called Superman position, also used sometimes in wrist imaging). In general, the prone position is less well tolerated and results in a greater number of motion-degraded studies. The elbow should be scanned in as comfortable position as possible to avoid such motion artifact. The elbow is typically extended and the wrist is placed in a neutral position. Patients who cannot extend the elbow are more difficult to position, and obtaining optimal imaging results requires more time and skill. Taping a vitamin E capsule or other marker to the skin at the site of tenderness or at the site of a palpable mass is useful to ensure that the area of interest has been included in the study, especially when there is no pathology identified on the images. Structures that curve in the extended position, especially the biceps tendon, may be better imaged by adopting the “Superman” position but with the elbow in 90° of flexion.9
With open MR scanners, the joint can generally be placed at the isocenter regardless of patient size or joint deformity. The patient can be positioned for optimal comfort rather than having to adopt a potentially painful posture. Increasing field strength (to 3 Tesla [T] or above) and the use of microscopy surface coils10 promise a much more detailed view of the joint.
Pulse Parameters
Excellent images may be obtained with both low- and high-field strength MR systems. With high-field systems (Fig. 9.1), proton density (PD) and fat-suppressed fast spin-echo (FS PD FSE) images are typically obtained in the axial and sagittal planes. T1- and PD-weighted and FS PD FSE or STIR sequences usually are obtained in the coronal plane. Although the STIR sequence has a relatively poor signal-to-noise ratio because of the suppression of signal from fat, pathologic changes are often more conspicuous due to the effects of additive T1 and T2 contrast. FS PD FSE sequences must be added to the FS T1-weighted sequence if arthrographic gadolinium contrast has been used. FS T1-weighted images alone are not sensitive to marrow or muscle edema, tendinosis, or cysts. FS PD FSE sequences may also be helpful in visualizing hyaline cartilage defects, especially in the coronal plane.
If a low-field open scanner is used, specific pulse sequences must be adopted to achieve optimal images. The reduced cross-talk between slices and better contrast resolution allow the successful use of gradient echo (GRE) T1-weighted 3D acquisitions to provide excellent hyaline cartilage depiction. In general, FSE T1 and fast-STIR sequences are the mainstay of elbow imaging. FSE T2 sequences suffer from reduced soft-tissue discrimination at field strengths of less than 0.5 T. Higher-field-strength open systems (0.7 T and above) are better able to generate acceptable images with sequences derived from conventional higher-field-strength scanners.
In general, axial images should extend from the distal humeral metaphysis to the radial tuberosity. The common flexor and extensor origins from the medial and lateral humeral epicondyles and the biceps insertion on the radial tuberosity are routinely imaged with this coverage. Images are usually obtained with 3- or 4-mm thick slices using a sequence with a long repetition time (TR). Coronal images are angled parallel to a line through the humeral epicondyles on the axial images, and sagittal images are angled perpendicular to a line through the humeral epicondyles on the axial images.
The field of view on axial images should be as small as the signal of the surface coil and the size of the patient’s elbow allow. The field of view for coronal and sagittal sequences is usually larger, to include more of the anatomy about the elbow. This is especially important when imaging a ruptured biceps tendon, which may retract 10 cm or more from the joint line. Slice thickness, interslice gap, and TR may be increased on axial sequences, just as the field of view is increased on coronal and sagittal sequences, as long as the surface coil provides adequate signal to image the entire length of the area of interest.
Additional sequences may be added or substituted, depending on the clinical problem being investigated. T2*-weighted
GRE sequences provide useful supplemental information for identifying loose bodies within the elbow. GRE volume sequences allow acquisition of multiple very thin axial images, which may subsequently be reformatted in any plane. In general, GRE sequences should be avoided after elbow surgery because magnetic susceptibility artifacts associated with micrometallic debris may obscure important findings and may also be mistaken for loose bodies. Artifact surrounding orthopaedic hardware is most prominent on GRE sequences because of the lack of a 180° refocusing pulse. It is least prominent on FSE sequences due to the presence of multiple 180° pulses. FSE T2-weighted sequences may be used to obtain higher-resolution images in the same amount of time as conventional spin-echo sequences or to reduce the overall time of the examination. The ability to shorten the examination with FSE is useful when scanning claustrophobic patients or patients who become uncomfortable in the prone position with the arm overhead.
GRE sequences provide useful supplemental information for identifying loose bodies within the elbow. GRE volume sequences allow acquisition of multiple very thin axial images, which may subsequently be reformatted in any plane. In general, GRE sequences should be avoided after elbow surgery because magnetic susceptibility artifacts associated with micrometallic debris may obscure important findings and may also be mistaken for loose bodies. Artifact surrounding orthopaedic hardware is most prominent on GRE sequences because of the lack of a 180° refocusing pulse. It is least prominent on FSE sequences due to the presence of multiple 180° pulses. FSE T2-weighted sequences may be used to obtain higher-resolution images in the same amount of time as conventional spin-echo sequences or to reduce the overall time of the examination. The ability to shorten the examination with FSE is useful when scanning claustrophobic patients or patients who become uncomfortable in the prone position with the arm overhead.
Fat suppression may be added to various pulse sequences to improve visualization of the hyaline articular cartilage. Avoidance of chemical shift artifact at the interface of cortical bone and fat-containing marrow permits a more accurate depiction of the overlying hyaline cartilage. FS T1-weighted images are useful whenever gadolinium is administered, either
intravenously or directly into the elbow joint as a dilute solution. Intravenous gadolinium may provide additional information in the assessment of neoplastic or inflammatory processes about the elbow. Articular injection of saline or dilute gadolinium may be useful in patients without a joint effusion to detect loose bodies, to determine if the capsule is disrupted, or to determine if an osteochondral fracture fragment is stable.
intravenously or directly into the elbow joint as a dilute solution. Intravenous gadolinium may provide additional information in the assessment of neoplastic or inflammatory processes about the elbow. Articular injection of saline or dilute gadolinium may be useful in patients without a joint effusion to detect loose bodies, to determine if the capsule is disrupted, or to determine if an osteochondral fracture fragment is stable.
Related Muscles
Relevant muscles about the elbow include related muscles of the arm and the volar and dorsal muscles of the forearm. The muscles of the arm include the biceps brachii (Fig. 9.2), the brachialis (Fig. 9.3), and the triceps brachii (Fig. 9.4). The superficial volar muscles of the forearm are the pronator teres (Fig. 9.5), the flexor carpi radialis (Fig. 9.6), the palmaris longus (Fig. 9.7), the flexor carpi ulnaris (Fig. 9.8), and the flexor digitorum superficialis (Fig. 9.9). The deep group of the volar muscles of the forearm are the flexor digitorum profundus (Fig. 9.10) and the flexor pollicis longus (Fig. 9.11). The pronator quadratus is covered in Chapter 10 on the wrist and hand. The superficial group of the dorsal muscles of the forearm includes the brachioradialis (Fig. 9.12), the extensor carpi radialis longus (Fig. 9.13), the extensor carpi radialis brevis (Fig. 9.14), the extensor digitorum (Fig. 9.15), the extensor digiti minimi (Fig. 9.16), the extensor carpi ulnaris (Fig. 9.17), and the anconeus (Fig. 9.18). The supinator (Fig. 9.19) is one of the deep dorsal muscles of the forearm. The others, include the abductor pollicis longus, the extensor pollicis brevis, the extensor pollicis longus, and the extensor indicis, are illustrated and discussed in Chapter 10 on the wrist and hand.
MR Anatomic Atlas of the Elbow
Pearls and Pitfalls
MR Anatomy
Axial scans are best for evaluating the neurovascular structures.
Sagittal scans show the bony articular relationship to best advantage.
There are normal “bare areas” at the ulnar trochlear notch and the capitellum; these should not be mistaken for pathology.
Coronal scans are best for evaluating common tendon origins and collateral ligaments.
The anatomic features of the elbow are best appreciated on conventional orthogonal triplane images obtained with the elbow in the fully extended position. Some structures, such as the biceps tendon, however, are better displayed if the elbow is imaged in 90° of flexion.14
Coronal Images
Coronal plane anatomy (Fig. 9.20) is key in demonstrating the collateral ligament complexes and common flexor and extensor tendon attachments:
The anterior bundle of the MCL, extending from the inferior margin of the medial epicondyle to the medial anterior margin of the coronoid process, is especially well demarcated, as is the lateral ulnar collateral ligament (LUCL), seen extending along the posterolateral aspect of the proximal radius to insert laterally on the tubercle of the supinator crest of the ulna.
The anterior articulation between the trochlea and the coronoid, the proximal radioulnar joint, and the articulation between the radius and the capitellum are all well demonstrated.
The rough nonarticular area at the posterior margin of the capitellum should not be mistaken for an osteochondral defect on coronal images through the posterior aspect of the radial head.
Axial Images
The axial plane (Fig. 9.21) demonstrates the distal course of the biceps and brachialis. The common flexor and extensor tendons and collateral ligaments as well as the ulnar nerve are well displayed:
The biceps and brachialis muscles are clearly depicted anteriorly, and the biceps tendon can be followed to the radial tuberosity and the brachialis tendon to the ulnar tuberosity.
The bicipital aponeurosis, also known as the lacertus fibrosus, appears as a thin black line that extends from the myotendinous junction of the biceps to the fascia overlying the flexor-pronator muscle group medially.
The median nerve and the brachial artery and veins lie just deep to the lacertus fibrosus, at the level of the medial epicondyle.
The hypointense common flexor and common extensor tendons can be seen arising from the medial and lateral epicondyles, respectively.
The radial nerve is located between the brachialis and brachioradialis muscles, and the deep branch can be followed distally as it passes between the deep and the superficial heads of the supinator muscle to form the posterior interosseus nerve (an important site of potential impingement).
The annular ligament is a thin hypointense structure that lies just superficial to the articular cartilage of the radial head, which demonstrates intermediate signal intensity.
The insertion of the LUCL can be seen at the lateral margin of the ulna at the level of the radial neck.
The insertion of the anterior bundle of the MCL can be seen at the medial margin of the coronoid process, just anterior and lateral to the ulnar nerve.
Posteriorly, the triceps tendon can be followed to the olecranon.
The anconeus muscle is well seen posterolaterally.
The ulnar nerve and accompanying posterior ulnar recurrent artery and veins are seen posteromedially, deep to the cubital tunnel retinaculum at the level of the medial epicondyle. The ulnar nerve can be followed distally as it passes deep to the humeral and ulnar heads of the flexor carpi ulnaris muscle.
The proximal radioulnar joint and the posterior compartment articulation between the olecranon and the olecranon fossa are also well seen.
The usual site of osteochondritis dissecans—along the anterior aspect of the capitellum—is also well seen on axial images.
Sagittal Images
The sagittal plane (Fig. 9.22) demonstrates the radiocapitellar and humerotrochlear articulations. The triceps tendon is visualized in the longitudinal axis. The common flexor and extension tendons and deeper layer of collateral ligaments are also depicted:
Laterally, the components of the common extensor tendon can be followed to the lateral epicondyle.
Medially, the components of the common flexor tendon can be seen extending proximally to the medial epicondyle.
The intermediate-signal-intensity ulnar nerve is seen along the posterior margin of the medial epicondyle.
Near the midline, the attachment of the triceps muscle and tendon to the olecranon can be seen.
Normal obliteration of the subcutaneous fat is seen posterior to the olecranon at the site of the superficial olecranon bursa.
The posterior and anterior fat pads can be seen along the margins of the distal humerus.
The joint capsule appears as a thin, hypointense structure just superficial to the fat pads.
The brachialis muscle lies just superficial to the anterior joint capsule and can be followed distally to its insertion on the ulnar tuberosity.
The biceps can be followed distally to its insertion on the radial tuberosity. The adjacent low-signal-intensity brachial and ulnar arteries should not be mistaken for the biceps tendon on sagittal images.
The articulation of the radius and the capitellum and the articulation between the trochlea and the trochlear notch are clearly demonstrated.
The normal bare area of the ulna in the midportion of the trochlear notch should not be confused with an osteochondral defect. Similarly, the rough nonarticular area at the posterior margin of the capitellum should not be confused with an impaction fracture or osteochondral defect.
Imaging Checklist for the Elbow
The elbow joint can be divided into medial, lateral, anterior, and posterior compartments. In the medial compartment, structures on the MR checklist include:
MCL
Common flexor tendon
Proximal flexor muscles
Ulnar nerve
Trochlea and coronoid (and overlying cartilage)
Medial epicondyle
In the lateral compartment, checklist structures include:
Radial collateral ligament (RCL)
LUCL
Common extensor tendon
Proximal extensor muscles
Radius and capitellum (and overlying cartilage)
The anterior compartment structures are:
Biceps tendon
Brachialis tendon and muscle
Radial and median nerves
The important posterior compartment structures are the triceps tendon and muscle.
The primary stabilizers of the elbow are:
Ulnohumeral joint (with the coronoid process as the key stabilizer)
MCL (primarily the anterior bundle)
LUCL
Secondary stabilizers include:
Radial head
Common flexor and extensor tendons
The severity of injuries progresses from ligament involvement alone, to involvement of the radial head, coronoid, and olecranon, to radial-ulnar dissociation (a tear of the interosseous ligament with fractures distal to the elbow).
Chronic repetitive injuries include tendinosis and chronic tearing of the common extensor tendon, which is seven times more common than chronic abnormalities of the common flexor tendon (medial epicondylitis). Although the following checklists are presented by plane, use of a multiscreen workstation with an image navigator bar is optimal for triangulating on anatomy and pathology simultaneously in multiple planes.
Coronal Plane Checklist
The examination of the elbow in the coronal plane begins in the medial aspect of the elbow with examination of the anterior bundle of the MCL and the common flexor tendon just superficial to the MCL. Subsequently, the lateral aspect of the joint is examined and the LUCL, the RCL, and the common extensor tendon just superficial to the lateral ligaments are viewed. The radiohumeral and ulnohumeral articulations are then inspected, including examination of the cartilage surfaces and assessment for fractures as well as loose bodies in the joint space. The anterior aspect of the elbow is then examined and the insertions of the biceps tendon into the radial tuberosity and the brachialis tendon into the ulnar tuberosity are evaluated. Finally, the posterior elbow and the triceps insertion are examined, and the full length of the ulnar nerve posterior to the medial epicondyle is visualized.
Medial Compartment
(1) Medial Collateral Ligament (Fig. 9.23)
The anterior bundle of the MCL is optimally visualized on coronal images. The anterior bundle originates at the anteroinferior surface of the medial epicondyle and courses in an oblique posterior fashion to attach firmly to the medial edge of the coronoid process at the sublime tubercle. The fibers of the anterior bundle are continuous with the sublime tubercle, and any fluid interposed between the distal fibers and the medial aspect of the sublime tubercle is interpreted as a deep articular-sided partial tear with partial stripping of the distal ligament from the sublime tubercle. The fluid signal is perpendicular to fluid within the ulnohumeral joint, resembling the letter “T,” and this appearance has been called the T sign. The anterior bundle is the primary constraint to valgus stress on the elbow
and is one of the main stabilizers of the elbow. The posterior bundle of the MCL is thought to be less functionally important and occasionally is difficult to visualize in the coronal plane.
and is one of the main stabilizers of the elbow. The posterior bundle of the MCL is thought to be less functionally important and occasionally is difficult to visualize in the coronal plane.
(2) Common Flexor Tendon (Fig. 9.24)
The common flexor tendon origin is visualized on coronal images arising medial and proximal to the MCL, coursing superficial to the MCL fibers. Tendinosis of the common flexor tendon is visualized as thickening and vague increased T2 (or fat-saturated PD) signal, whereas partial- or full-thickness tears demonstrate fluid signal disrupting tendon origin fibers. Not uncommonly, acute and chronic injuries of the common flexor tendon, often the result of valgus stress, are accompanied by evidence of ulnar neuritis and MCL injuries.
(3) Ulnohumeral Articulation (Fig. 9.25)
The articular cartilages lining the coronoid and trochlea are optimally visualized in the coronal plane, and chondral degeneration and associated subchondral edema and cystic change are evaluated. The size and location of any loose bodies within the joint secondary to chondral and osteochondral abnormalities are also identified. Fractures of the medial epicondyle, trochlea, and coronoid are also visualized in the coronal plane.
(4) Ulnar Nerve (Fig. 9.26)
The ulnar nerve courses medial to the distal humerus shaft and posterior to the medial epicondyle. Although axial images provide more detailed characterization of ulnar nerve injuries, high signal and other abnormalities of the ulnar nerve are confirmed in the coronal plane. A normal variant, the anconeus epitrochlearis (a small accessory muscle extending from the medial epicondyle to the medial ulna), occasionally causes ulnar nerve impingement by narrowing the cubital tunnel.
Lateral Compartment
(1) Lateral Collateral Ligament Complex (Fig. 9.27)
The lateral collateral ligament complex is composed of the RCL, the LUCL, and annular ligament. The RCL and LUCL are optimally visualized in the coronal plane. The RCL originates at the anterior distal aspect of the lateral epicondyle, deep to the common extensor tendon. The RCL fibers insert onto the annular ligament, which circumferentially surrounds the radial head. The origin of the LUCL is also deep to the common flexor tendon and somewhat posterior to the RCL origin along the lateral epicondyle. The LUCL swings posteromedially around the radial head, forming a posteromedial sling for the radius. The LUCL then inserts on the lateral aspect of the proximal ulna at the supinator crest. The most anterior image through the mid-radiocapitellar joint demonstrates the RCL coursing from the lateral epicondyle to the annular ligament surrounding the radial head. The common extensor tendon is superficial to the RCL and originates proximal to the RCL at this image location. On successive coronal images from anterior to posterior, the origin of the LUCL from the lateral epicondyle is visualized, the LUCL is seen swinging around the lateral aspect of the radial head, and the oblique course of the
LUCL posterior to the proximal radius is seen as it courses toward and attaches to the supinator crest on the ulna. Suspected tears and sprains of the RCL and LUCL are confirmed and further characterized in the axial and sagittal planes. Tears of the LUCL predispose to recurrent radial head dislocations. In cases of significant elbow trauma leading to dislocation, it is not uncommon for the RCL, LUCL, and common extensor tendon origin to completely tear off of the lateral epicondyle together as a single ligamentous/tendinous unit.
LUCL posterior to the proximal radius is seen as it courses toward and attaches to the supinator crest on the ulna. Suspected tears and sprains of the RCL and LUCL are confirmed and further characterized in the axial and sagittal planes. Tears of the LUCL predispose to recurrent radial head dislocations. In cases of significant elbow trauma leading to dislocation, it is not uncommon for the RCL, LUCL, and common extensor tendon origin to completely tear off of the lateral epicondyle together as a single ligamentous/tendinous unit.
(2) Common Extensor Tendon (Fig. 9.28)
The common extensor tendon originates from the anterior aspect of the lateral epicondyle and is visualized superficial to and somewhat parallel to the RCL. Common extensor tendinosis and tears are commonly referred to as lateral epicondylitis, or “tennis elbow.” The extensor carpi radialis brevis is the most commonly involved of the extensor tendons. The common extensor tendon is usually visualized on at least three consecutive coronal images from anterior to posterior.
(3) Radiohumeral Articulation (Fig. 9.29)
Cartilage covers the articular surface of the radial head and the opposing capitellum in a nearly 180° arc from anterior to posterior. Articular cartilage also extends over the medial aspect of the radial head and the lateral aspect of the coronoid, forming the third articulation of the elbow at the radioulnar joint. The cartilage covering the lateral aspect of the radial head is normally much thinner, and the absence of cartilage in this location
on coronal images should not be misinterpreted as a cartilage defect. Another common cartilage pseudolesion occurs at the lateral aspect of the capitellum, where a normal groove devoid of cartilage between the capitellum and lateral epicondyle may mimic an osteochondral defect. These cartilage surfaces must be examined carefully for the presence of chondral degeneration and associated stress-related subchondral edema and cystic change. Chondral degeneration is most commonly visualized on the opposing surfaces of the medial radial head and adjacent crest at the lateral margin of the trochlea. Osteochondral lesions of the capitellum commonly occur in teenage and young adult throwing athletes, whereas Panner’s disease (capitellar osteochondrosis) occurs in younger children. Fractures of the radial head and neck, not uncommonly occult on plain films, are easily visualized on MR images.
on coronal images should not be misinterpreted as a cartilage defect. Another common cartilage pseudolesion occurs at the lateral aspect of the capitellum, where a normal groove devoid of cartilage between the capitellum and lateral epicondyle may mimic an osteochondral defect. These cartilage surfaces must be examined carefully for the presence of chondral degeneration and associated stress-related subchondral edema and cystic change. Chondral degeneration is most commonly visualized on the opposing surfaces of the medial radial head and adjacent crest at the lateral margin of the trochlea. Osteochondral lesions of the capitellum commonly occur in teenage and young adult throwing athletes, whereas Panner’s disease (capitellar osteochondrosis) occurs in younger children. Fractures of the radial head and neck, not uncommonly occult on plain films, are easily visualized on MR images.
Anterior Compartment
(1) Biceps and Brachialis Tendons (Fig. 9.30)
Successive anterior-to-posterior images in the coronal plane display the biceps tendon coursing distally to attach at the radial tuberosity along the medial inferior aspect of the proximal radial shaft. The distal tendon is examined for sprain, tendinosis, or tear. If completely torn, the length of retraction is determined. Hypertrophic bony changes at the radial tuberosity insertion site are seen as indirect evidence of chronic distal biceps tendinosis or tearing. Also, fluid around the distal biceps tendon is compatible with bicipitoradial bursitis rather than tenosynovitis, since the distal biceps tendon has no tendon sheath. The brachialis tendon runs parallel and posterior to the biceps tendon and inserts along the anterior proximal ulna along the ulnar tuberosity. The brachialis is also evaluated for signs of tears and tendinosis.
Posterior Compartment
(1) Triceps Tendon (Fig. 9.31)
The triceps tendon is visible from its origin to its insertion on the olecranon. There is normal interposition of fat between the fascicles of the distal triceps tendon, which may produce a heterogeneous appearance to the distal fibers. This should not be misinterpreted as tendinosis as long as individual fibers are still well defined. Tendinosis and strain tend to obscure distinction of individual fibers. Coronal images are particularly useful in determining whether a tear of the distal triceps tendon is complete or partial, and, if partial, the extent and medial-to-lateral location of the tear. The degree of torn tendon retraction can be determined on coronal or sagittal images.
Axial Plane Checklist
Nearly all of the ligamentous and tendinous structures seen in the coronal plane are also visualized in the axial plane. By viewing successive proximal-to-distal axial images in the medial compartment, the origins of the MCL and common flexor tendon just superficial to the MCL are identified on the medial epicondyle, the ulnar nerve is visualized within the cubital tunnel posterior to the medial epicondyle, and a normal variant anconeus epitrochlearis muscle is occasionally seen overlying the ulnar nerve and cubital tunnel. Examination of the lateral aspect
of the elbow reveals the origins of the RCL and LUCL anteriorly along the lateral epicondyle and the origin of the common extensor tendon just posterior to the RCL and LUCL. The flexor and extensor muscles are inspected for strains, tears, or denervation injury. Proximal-to-distal images in the anterior compartment allow the biceps and brachialis tendons to be followed to their insertions. The course of the median and radial nerves is also examined anteriorly. In the posterior aspect of the joint the triceps tendon is evaluated for tears, strain, or tendinosis.
of the elbow reveals the origins of the RCL and LUCL anteriorly along the lateral epicondyle and the origin of the common extensor tendon just posterior to the RCL and LUCL. The flexor and extensor muscles are inspected for strains, tears, or denervation injury. Proximal-to-distal images in the anterior compartment allow the biceps and brachialis tendons to be followed to their insertions. The course of the median and radial nerves is also examined anteriorly. In the posterior aspect of the joint the triceps tendon is evaluated for tears, strain, or tendinosis.
Medial Compartment
(1) Medial Collateral Ligament (Fig. 9.32)
The anterior bundle of the MCLI is most easily found on axial images by first finding the axial image through a small ridge at the most proximal and medial portion of the medial ulna, known as the sublime tubercle. The anterior bundle is seen as a vertically oriented black band of signal lining up along the medial ulna. The anterior bundle can be followed proximally on successive images to where it fans out over its origin on the medial epicondyle. Distal MCL tears are commonly associated with bone marrow edema within the sublime tubercle. Although the coronal plane is probably most useful for evaluating the MCL, the axial and sagittal planes can confirm and further characterize MCL pathology.
(2) Common Flexor Tendon (Fig. 9.33)
Common flexor tendon pathology is visualized in cross-section on axial images. The common flexor tendon origin can be
identified along the anteromedial-most aspect of the medial epicondyle and its course can be followed distally to where it fans out into its various components making up the proximal flexor muscle group. The individual flexor muscles are also delineated and strains, tears, or denervation injuries are identified and localized.
identified along the anteromedial-most aspect of the medial epicondyle and its course can be followed distally to where it fans out into its various components making up the proximal flexor muscle group. The individual flexor muscles are also delineated and strains, tears, or denervation injuries are identified and localized.
(3) Ulnar Nerve (Fig. 9.34)
On axial images the ulnar nerve proximal to the elbow courses medially along the medial border of the triceps. It then descends posteriorly at the level of the elbow to run posterior to the medial epicondyle in the cubital tunnel. Distal to the elbow the ulnar nerve ascends between the two heads of the flexor carpi ulnaris muscle. The entire course of the ulnar nerve through the elbow is visualized in cross-section. T2 hyperintensity within the nerve is suggestive of, but not entirely specific for, ulnar neuritis. Swelling and enlargement of the nerve, and inflammatory infiltration of the perineural fat, increase the specificity of the diagnosis. An underlying structural cause for ulnar neuritis is only occasionally found but may include thickening of the cubital retinaculum overlying the nerve, a normal variant anconeus epitrochlearis muscle, neoplasms, bony spurs, posttraumatic deformities, and ganglion cysts. Ulnar nerve compression is sometimes caused by the anconeus epitrochlearis muscle (seen in 11% of the population), which is an anomalous muscle replacing the cubital retinaculum and extending posterolaterally from the posterior medial epicondyle to the medial ulna.
(4) Ulnohumeral Joint (Fig. 9.35)
Although usually identified in the coronal or sagittal plane, fractures of the coronoid, trochlea, olecranon, and medial epicondyle are further characterized in the axial plane. Loose bodies within the joint are also confirmed axially. Salter I physeal injuries of the medial epicondyle occur in teenage throwing athletes and are known as “Little Leaguer’s elbow.”
Lateral Compartment
(1) Lateral Collateral Ligament Complex (Fig. 9.36)
The origins of the RCL and LUCL are visualized in the axial plane along the anterior aspect of the lateral epicondyle. The RCL originates just anterior to the LUCL. From its origin, the short course of the RCL can be followed in cross-section to its insertion on the annular ligament. The longer, oblique course of the LUCL is more difficult to appreciate on axial images but can be identified on successive proximal-to-distal images as the LUCL turns 90° around the posterolateral aspect of the radial head to insert on the supinator crest of the ulna. Tears most commonly occur at the lateral epicondyle origin and are seen as fluid signal disrupting the normal location of origin fibers along the anterior lateral epicondyle. Axial plane images are used to confirm tears suspected on coronal or sagittal images and are sometimes helpful in localizing which components of the lateral stabilizers are involved.
(2) Common Extensor Tendon (Fig. 9.37)
Of the three lateral ligament/tendon structures arising from the lateral epicondyle, the origin of the common extensor tendon is the most posterior and proximal. Tears and tendinosis involving the common extensor tendon are evaluated in cross-section in the axial plane, as are the extensor muscles, which contribute to the common extensor tendon. Strain, tears, or denervation injuries of the extensor muscles, as well as the supinator and brachioradialis muscles, are localized on axial images.
(3) Radial-Humeral Articulation (Fig. 9.38)
Fractures or osteochondral defects of the radius and capitellum identified in other planes are further characterized in the axial plane. Loose bodies are also identified.
Anterior Compartment
(1) Biceps and Brachialis Tendons (Fig. 9.39)
The biceps and brachialis tendons are seen in cross-section, and the biceps tendon should be followed to its distal insertion on the medial inferior aspect of the proximal radius, at the radial tuberosity. In addition, radial tuberosity hypertrophy from chronic tendinosis and distal bicipitoradial bursitis are also evaluated in the axial plane. On images proximal to the elbow, the brachialis tendon begins anterolaterally, whereas the biceps is anteromedial. The brachialis runs posterior to the biceps. As the tendons are followed distally, the biceps courses posterolaterally, whereas the brachialis runs posteromedially. The two tendons cross mid-elbow, with the
brachialis eventually inserting medially on the proximal ulna and the biceps inserting on the proximal radius.
brachialis eventually inserting medially on the proximal ulna and the biceps inserting on the proximal radius.
(2) Median and Radial Nerves (Fig. 9.40)
The radial nerve is seen coursing between the brachialis and brachioradialis anterolaterally, splitting into superficial and deep branches near the level of the biceps myotendinous junction. The median nerve runs just medial to the brachial artery, anterior to the brachialis muscle. Evidence of median and radial neuritis is usually seen on axial MR images as denervation (hyperintensity on FS PD FSE images) and/or fatty atrophy of the muscles innervated by the affected nerve. Occasionally, an underlying cause for the neuritis is identified, such as a mass lesion or an anatomic anomaly (e.g., severe bicipitoradial bursitis compressing the median nerve or a ganglion cyst compressing the radial nerve).
Posterior Compartment
(1) Triceps Tendon (Fig. 9.41)
The triceps tendon is also imaged in cross-section in the axial plane. It inserts in a U shape along the olecranon. Triceps tendon tears, tendinosis, or strain is evaluated in the axial plane.
Sagittal Plane Checklist
On medial-to-lateral sagittal images, the full length of the common flexor tendon is seen first on the medial-most images. The MCL is depicted next, although it is occasionally difficult to see in the sagittal plane. The ulnar nerve is visualized coursing posterior to the medial epicondyle. The ulnohumeral and radiohumeral articulations are then examined, inspecting the cartilage surfaces and looking for loose bodies. On the central images, the biceps and brachialis tendons are visualized anteriorly and the triceps tendon posteriorly. At the lateral elbow, the origins of the RCL and LUCL are evaluated. The common extensor tendon is visualized on one image lateral to the RCL and LUCL origin.
Medial Compartment
(1) Common Flexor Tendon and Ulnar Nerve (Fig. 9.42)
On medial-to-lateral sagittal images through the elbow, the common flexor tendon is seen on the most medial images as a hypointense band of signal parallel to the long axis of the ulna, coursing to insert on the medial epicondyle. Tears, strain, or tendinosis of the common flexor tendon can be characterized
on sagittal images, while triangulating on the tendon simultaneously in the coronal and axial planes. The anterior bundle of the MCL is not as well seen on sagittal images but can occasionally be visualized on images just deep to the common flexor tendon. Strain or tears of the flexor muscles distal to the common flexor tendon insertion are also evaluated on sagittal images.
on sagittal images, while triangulating on the tendon simultaneously in the coronal and axial planes. The anterior bundle of the MCL is not as well seen on sagittal images but can occasionally be visualized on images just deep to the common flexor tendon. Strain or tears of the flexor muscles distal to the common flexor tendon insertion are also evaluated on sagittal images.
The ulnar nerve can be seen coursing just posterior to the medial epicondyle, making sagittal plane images useful for confirming abnormalities suspected on axial plane images. The ulnar nerve is often visualized on the same sagittal image as the common flexor tendon.
(2) Ulnohumeral Articulation (Fig. 9.43)
The cartilages lining the coronoid, olecranon, and trochlea are particularly well visualized and characterized on sagittal images. One common pitfall is a normal pseudodefect in the middle of the trochlear notch between the olecranon and coronoid articular surfaces, formed by a normally cartilage-free groove. Fractures of the coronoid process, olecranon, and trochlea are characterized with regard to size and displacement. The size of the coronoid process fracture fragment is important in determining potential elbow instability, and the fracture fragment is measured in the sagittal plane. On successive medial-to-lateral sagittal images near the midportion of the joint, the bone between the trochlea and the distal humeral shaft becomes thin, forming anterior and posterior fossae known as the coronoid fossa anteriorly (which receives the coronoid on flexion) and the olecranon fossa posteriorly (which receives the posterior olecranon on extension). These two fossae are common sites for loose bodies, anterior more often than posterior. In addition, in cases of degenerative arthritis, these two fossae are examined for the presence of hypertrophic bone, which may limit flexion and extension.
Lateral Compartment
(1) Common Extensor Tendon and Lateral Collateral Ligament Complex (Fig. 9.44)
On lateral-to-medial sagittal images through the lateral compartment, the common extensor tendon is seen first on the most peripheral lateral slice. It appears as a hypointense band of signal parallel to the radius and can often be visualized along its entire course on one or two images before inserting on the lateral epicondyle. The myotendinous junction of the proximal extensor muscles is also visualized on sagittal images. Strains or tears of the proximal extensor muscles and tendon are well characterized on these images.
The lateral collateral ligament complex is encountered medial to the common extensor tendon. Both the RCL and LUCL are often identified on the same image. The RCL is located just anterior to the LUCL, and the RCL courses distally from the lateral epicondyle, parallel to the long axis of the radius, to insert on the annular ligament fibers surrounding the radial head. The LUCL is located just posterior to the RCL, and the fibers diverge to run posteriorly and distally. The origins
of the LUCL and RCL overlap somewhat on MR images, and occasionally it is difficult to completely distinguish them. The course of the LUCL, as it runs behind the radial head to insert on the supinator crest of the ulna, can be followed on the next three or four successive medial images. The sagittal plane is helpful to confirm or further characterize tears of the lateral ligaments and tendons that are suspected on images obtained in other planes.
of the LUCL and RCL overlap somewhat on MR images, and occasionally it is difficult to completely distinguish them. The course of the LUCL, as it runs behind the radial head to insert on the supinator crest of the ulna, can be followed on the next three or four successive medial images. The sagittal plane is helpful to confirm or further characterize tears of the lateral ligaments and tendons that are suspected on images obtained in other planes.
(3) Radiohumeral Articulation (Fig. 9.45)
The cartilage surfaces covering the radial head and the nearly 180° arc of the capitellum are visualized on sagittal images, as are loose bodies displaced anteriorly or posteriorly in the joint. Fractures of the radial head and capitellum are characterized on these images as well. Similar to the coronoid fossa on the ulnohumeral side, there is a radial fossa forming a small concavity on the anterior surface of the distal humerus, which receives the radial head in flexion. The radial fossa is examined for loose bodies and osteophytes.
Anterior Compartment
Biceps and Brachialis Tendon (Fig. 9.46)
On sagittal images through the lateral aspect of the joint, the entire course of the distal biceps tendon can be visualized on one or two images coursing parallel to the distal humeral shaft before curving 90° to insert on the radial tuberosity. Complete tears, partial tears, and tendinosis are characterized with regard to the extent and the location of the involved tendon. In cases of complete tendon rupture, the amount of proximal retraction is measured.
Analogous to the biceps tendon in the lateral aspect of the joint, the full course of the distal brachialis muscle and tendon can be visualized on sagittal images through the medial joint. The brachialis courses parallel and posterior to the biceps tendon, and its distal course and insertion on the anterior proximal ulna are often visualized on the same image as the biceps tendon, or on one image medial to the biceps insertion.
Posterior Compartment
(1) Triceps Tendon (Fig. 9.47)
Sagittal plane images through the midline demonstrate the insertion of the distal triceps muscle and tendon on the olecranon. Tendinosis, strain, and tears (including the degree of retraction) can be characterized on these images.
Sample MRI Report, Elbow Injury
Clinical Information: Partial dislocation, hyperextension mechanism. Evaluate for medial ligament tear.
Technique: Axial, coronal, and sagittal T1-weighted images without fat suppression and proton density images with fat suppression.
Findings: There is a partial undersurface tear of the distal aspect of the anterior band of the medial collateral ligament (Fig. 9.48A). There is a sprain of the proximal fibers of the anterior
band of the medial collateral ligament (Fig. 9.48B). There is mild tendinosis of the common flexor tendon (Fig. 9.48C). There is mild tendinosis of the common extensor tendon. There is a sprain of the proximal origin of the lateral ulnar collateral ligament with bone marrow edema in the lateral aspect of the capitellum (Fig. 9.48D). There is muscle edema corresponding to the flexor digitorum superficialis muscle, indicating a muscle strain (Fig. 9.48E). There is also mild edema demonstrated in the extensor digitorum muscle (Fig. 9.48F).
band of the medial collateral ligament (Fig. 9.48B). There is mild tendinosis of the common flexor tendon (Fig. 9.48C). There is mild tendinosis of the common extensor tendon. There is a sprain of the proximal origin of the lateral ulnar collateral ligament with bone marrow edema in the lateral aspect of the capitellum (Fig. 9.48D). There is muscle edema corresponding to the flexor digitorum superficialis muscle, indicating a muscle strain (Fig. 9.48E). There is also mild edema demonstrated in the extensor digitorum muscle (Fig. 9.48F).
There is moderate edema within the brachialis muscle consistent with a grade 1 to 2 muscle strain of the brachialis muscle with hemorrhage (Fig. 9.48G). The biceps tendon and brachialis tendon are seen in continuity. Anterior soft-tissue hemorrhage is apparent and capsular distention is appreciated on sagittal images. There is elevation of the anterior and posterior fat pads secondary to a large hemorrhagic joint effusion (Fig. 9.48H).
Axial images confirm extensive edema and hemorrhage within the brachialis muscle group (Fig. 9.48I). The ulnar nerve is seen without hyperintensity.
Impression:
Partial undersurface tear of the distal aspect of the anterior band medial collateral ligament. There is a small focus of decreased signal intensity associated with this, which may represent a small osseous avulsion. There is a sprain of the proximal fibers of the anterior band of the medial collateral ligament.
There is a sprain of the origin of the lateral ulnar collateral ligament associated with subchondral marrow edema of the lateral aspect of the capitellum.
Grade 1 to 2 muscle strain of the brachialis. Mild grade 1 strain of the extensor digitorum muscle and also grade 1 strain of the flexor digitorum superficialis muscle medially.
Large hemorrhagic joint effusion with elevation of anterior and posterior fat pads
Intact brachialis and biceps tendon distally
Normal Anatomy of the Elbow
Pearls and Pitfalls
Normal Anatomy of the Elbow
The elbow is a tri-arthrodial ginglymus joint.
The medial and lateral humeral condyles are the origin of the common flexor and extensor groups of the forearm.
The coronoid process is the key to the bony stability of the joint.
Posterolateral stability depends on the LUCL.
The normal but complex maturation of the elbow ossification centers can be mistaken for pathology.
A thorough understanding of the anatomy and function of the elbow is essential for accurate interpretation of MR images. The anatomic structures of the elbow are depicted reliably on MR images, and knowledge of the relative functional significance of these structures allows assessment of clinically important anatomy. Focusing on the relevant anatomic structures leads to more meaningful interpretation of the images and facilitates clinical problem solving. As with other joints, new knowledge gained from arthroscopic examination usefully modifies and refines this process.14
Osseous and Articular Anatomy
The elbow is a tri-arthrodial ginglymus joint; in other words, it is composed of three articulations (the radioulnar, radiohumeral, and ulnohumeral), which are contained within a common joint cavity and together work like a hinge. These three articulations arise from three embryonic mesenchymal cavities, which in normal development merge to form one cavity. Remnants of the dividing membranes occasionally remain as plicae in the adult.15 The articulation of the radius and ulna makes up the proximal radioulnar joint. The radial head rotates within the radial notch of the ulna, allowing supination and pronation distally. The capitellum also articulates with the radius and the trochlea articulates
with the trochlear notch of the ulna in a hinge fashion, allowing flexion and extension of the elbow joint (Fig. 9.49).
with the trochlear notch of the ulna in a hinge fashion, allowing flexion and extension of the elbow joint (Fig. 9.49).
Humerus
The distal humerus consists of medial and lateral condyles as well as the articular surfaces of the trochlea and the capitellum. The trochlea is a pulley-like surface that articulates with the trochlear notch of the ulna. A continuous surface of articular cartilage covers the trochlea and forms an arc of about 300° to 330°. The trochlear groove courses from anterolateral to posteromedial, defining medial and lateral lips of the trochlea. The capitellum, also known as the capitulum, is an anteriorly directed sphere that articulates with a depression in the radial head. The trochleocapitellar groove articulates with the medial rim of the radial head throughout the arc of flexion and extension (Fig. 9.50). The crest at the trochlear margin of the trochleocapitellar groove and the adjacent medial rim of the radial head are common sites of early articular cartilage loss.
The prominent medial epicondyle arises proximal to the trochlea and serves as the site of origin for the flexor-pronator muscle group via the common flexor tendon. The MCL also originates from the medial epicondyle, and there is a groove or sulcus for the ulnar nerve at its posterior margin. The lateral epicondyle is much less prominent than the medial epicondyle. It arises just proximal to the capitellum and serves as the site of origin for the extensor-supinator muscle group and the lateral collateral ligament. The radial fossa of the humerus lies just
proximal to the capitellum anteriorly and receives the radial head during flexion (see Fig. 9.50). The coronoid fossa lies just proximal to the trochlea and receives the coronoid process during flexion. Posteriorly, the olecranon fossa receives the tip of the olecranon during extension (Fig. 9.51). The olecranon and coronoid fossae are separated by a thin membrane of bone in about 90% of individuals. A supracondylar process of the humerus is found about 6 cm proximal to the medial epicondyle in 1% to 3% of individuals.16 The supracondylar process may fracture and contribute to median and ulnar nerve entrapment.
proximal to the capitellum anteriorly and receives the radial head during flexion (see Fig. 9.50). The coronoid fossa lies just proximal to the trochlea and receives the coronoid process during flexion. Posteriorly, the olecranon fossa receives the tip of the olecranon during extension (Fig. 9.51). The olecranon and coronoid fossae are separated by a thin membrane of bone in about 90% of individuals. A supracondylar process of the humerus is found about 6 cm proximal to the medial epicondyle in 1% to 3% of individuals.16 The supracondylar process may fracture and contribute to median and ulnar nerve entrapment.
Ulna
The proximal ulna comprises the olecranon and coronoid processes, which together form the articular surface of the trochlear notch. The trochlear notch is also known as the greater sigmoid notch or semilunar notch. The congruent articulation of the humeral trochlea and the trochlear notch of the ulna are largely responsible for the inherent bony stability of
the elbow joint. It is for this reason that any fracture of the coronoid process (which forms the anterior lip of the notch) has significant implications for the future stability and motion of the joint. The ulnohumeral articulation allows the hinge-like flexion and extension of the elbow. The trochlear notch is divided longitudinally into medial and lateral facets by a guiding ridge. In most individuals, the trochlear notch is also divided transversely by a bare area that is not covered by a continuous surface of articular cartilage (Fig. 9.52). Therefore, in most individuals there are four articular surfaces of the trochlear notch that articulate with the trochlea of the humerus. A fifth articular surface, known as the radial notch (Fig. 9.53), arises just distal to the coronoid laterally and articulates with the radial head. This radial notch, also known as the lesser sigmoid notch, consists of an arc of about 70° and is oriented perpendicular to the greater sigmoid notch.
the elbow joint. It is for this reason that any fracture of the coronoid process (which forms the anterior lip of the notch) has significant implications for the future stability and motion of the joint. The ulnohumeral articulation allows the hinge-like flexion and extension of the elbow. The trochlear notch is divided longitudinally into medial and lateral facets by a guiding ridge. In most individuals, the trochlear notch is also divided transversely by a bare area that is not covered by a continuous surface of articular cartilage (Fig. 9.52). Therefore, in most individuals there are four articular surfaces of the trochlear notch that articulate with the trochlea of the humerus. A fifth articular surface, known as the radial notch (Fig. 9.53), arises just distal to the coronoid laterally and articulates with the radial head. This radial notch, also known as the lesser sigmoid notch, consists of an arc of about 70° and is oriented perpendicular to the greater sigmoid notch.
The triceps muscle and tendon attach to the posterosuperior nonarticular aspect of the olecranon (Fig. 9.54). The proximal tip of the olecranon is separated from the tendon by a subtendinous olecranon bursa. The brachialis muscle and tendon insert on the anterior nonarticular aspect of the coronoid and the ulnar tuberosity distally, at the level of the radial tuberosity. The anterior bundle of the MCL inserts at the medial margin of the coronoid process. A crest along the lateral aspect of the ulna is the site of the ulnar origin of the supinator muscle. A tubercle on the supinator crest serves as the insertion site for the ulnar part of the lateral collateral ligament, also known as the LUCL. The annular ligament arises from the posterior and anterior margins of the radial notch of the ulna (see Fig. 9.52, Fig. 9.55).
Radius
The proximal radius comprises the radial head and neck as well as the radial tuberosity (Fig. 9.56). The radial head has a central depression that articulates with the capitellum. Two thirds of the outer rim of the radial head is covered with hyaline cartilage and articulates with the radial notch of the ulna
at the proximal radioulnar joint. The anterolateral third of the radial circumference is normally devoid of articular cartilage and lacks strong subchondral bone. It is this portion of the radial head that is most commonly fractured. The radial tuberosity (see Fig. 9.56), at the distal margin of the radial neck, consists of an anterior surface for the bicipitoradial bursa and a posterior aspect where the biceps tendon attaches. The bicipitoradial bursa separates the biceps tendon from the radial tuberosity during full pronation. The pseudodefect of the capitellum (Fig. 9.57) is seen at the radial head-capitellar articulation on posterior coronal and on sagittal images. This is the result of the normal interruption of the posterior capitellar articular surface at the junction with the lateral epicondyle.
at the proximal radioulnar joint. The anterolateral third of the radial circumference is normally devoid of articular cartilage and lacks strong subchondral bone. It is this portion of the radial head that is most commonly fractured. The radial tuberosity (see Fig. 9.56), at the distal margin of the radial neck, consists of an anterior surface for the bicipitoradial bursa and a posterior aspect where the biceps tendon attaches. The bicipitoradial bursa separates the biceps tendon from the radial tuberosity during full pronation. The pseudodefect of the capitellum (Fig. 9.57) is seen at the radial head-capitellar articulation on posterior coronal and on sagittal images. This is the result of the normal interruption of the posterior capitellar articular surface at the junction with the lateral epicondyle.
Epiphyseal Maturation
The capitellum is the first of the six ossification centers about the elbow to appear radiographically. It generally becomes visible at 1 to 2 years of age (Fig. 9.58). The medial epicondyle appears next, at about 4 years of age. It is the last ossification center to fuse with the humerus. The medial epicondyle usually does not fuse with the humerus until age 15 or 16. The radial head ossifies shortly after the medial epicondyle, usually around 5 years of age. It often matures as one or more flat sclerotic centers that may, on radiographs, be mistaken for a fracture or avascular necrosis. At about 8 years of age the trochlea ossifies, sometimes in a multicentric fashion that on radiographs may be confused with fractures or osteochondrosis. MR imaging confirms the normal appearance of multiple trochlear ossification centers that contain high-signal-intensity yellow marrow on T1-weighted images. The olecranon ossifies at about 9 years of age, shortly after the trochlea and just before
the lateral epicondyle. The lateral epicondyle appears at about 10 or 11 years of age and fuses at approximately age 14. It initially appears as a thin, vertically oriented sliver that may be mistaken for an avulsion fracture.
the lateral epicondyle. The lateral epicondyle appears at about 10 or 11 years of age and fuses at approximately age 14. It initially appears as a thin, vertically oriented sliver that may be mistaken for an avulsion fracture.
Awareness of the normal orderly sequence of epiphyseal maturation about the elbow is important in recognizing a medial epicondyle that has been avulsed and trapped in the medial aspect of the joint. The trochlear ossification center is normally not seen radiographically before the appearance of the medial epicondyle. Therefore, the finding of an apparent trochlear ossification center in a child 4 to 8 years of age without visualization of the medial epicondyle suggests avulsion and displacement of the medial epicondyle.
Joint Capsule and Collateral Ligaments
The anterior and posterior portions of the joint capsule are relatively thin, whereas the medial and lateral portions are thickened to form the collateral ligaments. The fibers of the anterior capsule have a cruciate orientation, which results in significant strength. The anterior capsule is normally lax in flexion and taut in extension. The synovial membrane lines the joint capsule. The normal capacity of the fully distended joint is 25 to 30 mL. Intracapsular, but extrasynovial, fat pads normally occupy the coronoid fossa anteriorly and the olecranon fossa posteriorly (see Fig. 9.54). Displacement of these fat pads is a well-known radiographic sign of an elbow effusion or hemarthrosis. A synovial fold (Fig. 9.59) extending from the posterior fat pad is a frequent finding. As in the knee, these folds occasionally cause symptoms requiring arthroscopic treatment.
Medial Collateral Ligament Complex
The MCL complex consists of anterior and posterior bundles as well as an oblique band, which is also known as the transverse ligament (Fig. 9.60). The posterior bundle and the transverse ligament lie at the deep margin of the ulnar nerve and make up the floor of the cubital tunnel. The functionally important anterior bundle of the MCL extends from the inferior aspect of the medial epicondyle to the medial aspect (sublime tubercle)
of the coronoid process (see Fig. 9.50B). The anterior bundle, which is clearly displayed on coronal images (Fig. 9.61), provides the primary constraint to valgus stress and commonly is damaged in throwing athletes (Fig. 9.62).17,18,19 However, the anterior band has a variable insertion that should not be mistaken for pathology.20
of the coronoid process (see Fig. 9.50B). The anterior bundle, which is clearly displayed on coronal images (Fig. 9.61), provides the primary constraint to valgus stress and commonly is damaged in throwing athletes (Fig. 9.62).17,18,19 However, the anterior band has a variable insertion that should not be mistaken for pathology.20
Lateral Ligament Complex
The lateral ligament complex (Fig. 9.63) consists of:
RCL (Fig. 9.64)
Annular ligament (see Fig. 9.64)
Variably present accessory lateral collateral ligament
The RCL proper arises from the lateral epicondyle anteriorly and blends with the fibers of the annular ligament, which surrounds the radial head. The annular ligament is the primary stabilizer of the proximal radioulnar joint and is evaluated best on axial images. The annular ligament is tapered distally to form a funnel about the radial head. The anterior fibers of the annular ligament become taut in supination, whereas the posterior fibers become taut at the extreme of pronation. Disruption of the annular ligament results in proximal radioulnar joint instability. The accessory lateral collateral ligament is an inconstant structure that extends from the annular ligament to the supinator crest along the lateral aspect of the ulna. When present, it acts to further stabilize the annular ligament during varus stress. A more posterior bundle, known as the ulnar part of the lateral collateral ligament or the LUCL, arises from the lateral epicondyle and extends along the posterior aspect of the radius to insert on the tubercle of the supinator crest of the ulna. Anatomic dissection has shown that the LUCL is invariably present.13,16 The LUCL acts as a sling or guy-wire that provides the primary ligamentous constraint to varus stress.19,20,21,22 Disruption (or division) of the LUCL results in a pivot shift phenomenon and posterolateral rotatory instability of the elbow.23 Both the RCL proper and the LUCL are clearly displayed on coronal images progressing from anterior to posterior. They should be considered separately because of the difference in functional significance of these structures. Anatomic dissection has shown that appearances may be variable and arthrography may be needed to aid visualization.22,23
Muscles and Tendons
The muscles of the elbow are divided into anterior, posterior, medial, and lateral compartments:
The anterior compartment contains the biceps and brachialis muscles (Fig. 9.66), which are evaluated best on sagittal and axial images. The brachialis extends along the anterior joint capsule and inserts on the ulnar tuberosity. The biceps lies superficial to the brachialis and inserts on the radial tuberosity. At the level of the joint line, the biceps is visualized only as a small anteriorly placed tendon.
The posterior compartment contains the triceps (see Fig. 9.66) and anconeus (Fig. 9.67) muscles, and they are evaluated best on sagittal and axial images. The triceps inserts on the proximal aspect of the olecranon. The anconeus arises from the posterior aspect of the lateral epicondyle and inserts more distally on the olecranon. The anconeus provides dynamic support to the lateral collateral ligament in resisting varus stress.
The medial and lateral compartment muscles are seen best on coronal and axial images:
The medial compartment structures (Fig. 9.68) include the pronator teres and the flexors of the hand and wrist that arise from the medial epicondyle as the common flexor tendon. The common flexor tendon provides dynamic support to the MCL in resisting valgus stress.
The lateral compartment structures (Figs. 9.69 and 9.70) include the supinator, the brachioradialis, the extensor carpi radialis longus, as well as the extensors of the hand and wrist that arise from the lateral epicondyle as the common extensor tendon.
As in other joints there is variant muscular anatomy, most notably the anconeus epitrochlearis, which lies posterior to the common flexor origin and may be a potential cause of ulnar nerve compression.
Neurovascular Structures
The brachial artery and veins descend along the anteromedial aspect of the brachialis muscle in the arm. The brachial artery (Fig. 9.71) branches into the radial and ulnar arteries (see Fig. 9.71) along the anteromedial aspect of the biceps tendon at the level of the radial head. The radial artery (see Fig. 9.71) has a more proximal origin in up to 15% of individuals. The ulnar, median, musculocutaneous, and radial nerves are subject to entrapment in the elbow region. These nerves normally are surrounded by fat and are seen best on axial images. Prominent veins may accompany the median or ulnar nerves and should not be mistaken for swollen, edematous nerves on T2-weighted or STIR sequences.
Pathology of the Elbow
Medial Collateral Ligament Injury
Pearls and Pitfalls
Medial Collateral Ligament Injury
The anterior band of the MCL is the primary restraint to valgus stress.
MCL rupture frequently occurs with posterior dislocation.
The MR “T sign” is indicative of a partial tear at the sublime tubercle, which may be occult to surgical inspection.
Strain of the flexor digitorum superficialis frequently accompanies an MCL injury.
Lateral compartment bone bruises strongly suggest MCL disruption.
Degeneration and tearing of the MCL, with or without concomitant injury of the common flexor tendon, is a common injury in throwing athletes.
Diagnosis, Etiology, and Clinical Features
Damage to the medial stabilizing structures is usually caused by chronic microtrauma from repetitive valgus stress. The anterior band of the MCL (Fig. 9.72) is the primary soft-tissue restraint to valgus stress, and it is most at risk during the acceleration phase of throwing (Fig. 9.73).22,23,24 The MCL may also be injured in posterior dislocation of the elbow. Complete rupture of the anterior bundle of the MCL usually occurs as a sudden event. There may be an acute popping sensation followed by pain and limitation of extension. Clinically, there may be crepitus on palpation of the ligament, and Tinel’s sign may be positive.
Midsubstance MCL ruptures (Fig. 9.74) can be differentiated from proximal avulsions (Fig. 9.75) and distal avulsions (Fig. 9.76). In one study of a large series of surgically treated throwing athletes,17 midsubstance ruptures of the MCL were the most common (87%), and distal (10%) and proximal (3%) avulsions were found less frequently. In other reports, midsubstance ruptures were not as commonly found.25,26 Since the fibers of the flexor digitorum superficialis muscle blend with the anterior bundle of the MCL,16 associated strains of the flexor digitorum superficialis muscle commonly occur when the MCL is injured (see Fig. 9.76). In addition, ulnar traction spurs at the insertion of the MCL on the coronoid process (caused by repetitive valgus stress) have been found in 75% of professional baseball pitchers.27 Chronic degeneration of the MCL is characterized by thickening of the ligament secondary to scarring, often accompanied by foci of calcification or heterotopic bone (Fig. 9.77).17 The findings are similar to those seen after healing of MCL sprains in the knee, in which the development of heterotopic ossification has been termed the Pellegrini-Stieda phenomenon.
A number of different conditions may occur secondary to the repeated valgus stress to the elbow that occurs with throwing (Fig. 9.78). Medial tension overload typically produces extra-articular injury such as flexor-pronator strain, MCL sprain, ulnar traction spurring, and ulnar neuropathy. Lateral compression overload typically produces intra-articular injury such as osteochondritis dissecans of the capitellum or radial head, degenerative arthritis, and loose body formation. All of these related pathologic processes associated with repeated valgus stress can be assessed with MR imaging.28 The additional information provided by MR imaging can be helpful in formulating a logical treatment plan, especially when surgery is being considered.
Rupture of the MCL is also commonly encountered as a result of posterior dislocation of the elbow.29,30 After the shoulder, the elbow is the second most common joint to be dislocated.30 The mechanism of posterior elbow dislocation usually involves falling on an outstretched arm. Typically there is rupture of the medial and lateral collateral ligaments as well as the anterior and posterior capsule during posterior elbow dislocation.22 Associated rupture of the common extensor tendon or the common flexor tendon may also occur. The extent of injury secondary to elbow dislocation is well delineated with MR imaging.
MR Appearance
Acute injury of the MCL (Fig. 9.79) can be detected, localized, and graded with MR imaging. The status of the functionally important anterior bundle of the MCL complex is best assessed on axial and coronal images (Fig. 9.80). Partial tears of the MCL, which may also occur in pitchers with medial elbow pain, characteristically spare the superficial fibers of the anterior bundle and are therefore not visible with an open surgical approach unless
the ligament is incised to inspect the torn capsular fibers.31,32 MR imaging, therefore, is particularly important in localizing these partial tears, which are treated with repair or reconstruction. Detection of partial detachment of the deep undersurface fibers of the anterior bundle (Fig. 9.81) may require intra-articular contrast and MR or CT arthrography.33 The capsular fibers of the anterior bundle of the MCL normally insert on the medial margin of the coronoid process at the sublime tubercle. Undersurface partial tears of the anterior bundle are characterized by distal extension of fluid or contrast along the medial margin of the coronoid, producing the so-called T sign.33 FS images may show marrow edema due to a stress response at the humeral origin or the coronoid attachment. It is important to differentiate partial tears from an anatomically variant distal MCL insertion (Fig. 9.82).
the ligament is incised to inspect the torn capsular fibers.31,32 MR imaging, therefore, is particularly important in localizing these partial tears, which are treated with repair or reconstruction. Detection of partial detachment of the deep undersurface fibers of the anterior bundle (Fig. 9.81) may require intra-articular contrast and MR or CT arthrography.33 The capsular fibers of the anterior bundle of the MCL normally insert on the medial margin of the coronoid process at the sublime tubercle. Undersurface partial tears of the anterior bundle are characterized by distal extension of fluid or contrast along the medial margin of the coronoid, producing the so-called T sign.33 FS images may show marrow edema due to a stress response at the humeral origin or the coronoid attachment. It is important to differentiate partial tears from an anatomically variant distal MCL insertion (Fig. 9.82).
![]() FIGURE 9.76 ● Distal avulsion of the anterior bundle of the MCL (A) and disruption of the posterior bundle of the MCL (B) on coronal FS PD FSE images. |
![]() FIGURE 9.77 ● Proximal anterior bundle avulsion and heterotopic ossification on T1-weighted coronal image. |
Typical MR findings include:
Heterotopic ossification indicated by increased fat signal of bone marrow in the ligament (Fig. 9.83) or hypointensity in sclerotic ossification
Increased signal within the ligament, usually in the anterior bundle on FS PD FSE images (Fig. 9.84)
A chronically thickened ligament (anterior bundle), hyperintense on T1- or PD-weighted images and hypointense on FS PD FSE images (Fig. 9.85)
Epicondylar avulsion, including visualization of corticated structures, the donor site on the humerus, surrounding edema and Little Leaguer’s elbow, and a hyperintense sublime tubercle from stress response avulsive stress
Stress response or fracture at the humeral origin site or attachment site on ulnar coronoid
Synovitis, intermediate to hyperintense on FS PD FSE images
A traction spur (hyperintense on T1- and PD-weighted images if it contains marrow and hypointense if it is calcified)
Associated lateral impaction injury, on T1- and PD-weighted images indicated by hypointense (if edematous) capitellum, hypointense subchondral cysts (in chronic injury), and hypointense subchondral sclerosis, hypointense loose bodies (especially in osteoarthritis)
Possible visualization of discontinuity of fibers in a complete tear
Possible hypertrophy of sublime tubercle
Hyperintense associated flexor-pronator (FPG) strain or tear on FS PD FSE images (Figs. 9.86 and 9.87)
![]() FIGURE 9.83 ● Chronic anterior bundle MCL proximal tear with heterotopic ossification within the substance of the ligament. (A) Coronal T1-weighted FSE image. (B) Axial T1-weighted FSE image. |
![]() FIGURE 9.84 ● Hyperintensity in a partial tear of the anterior bundle and anterior fibers of the posterior bundle of the MCL. (A, B) Coronal FSE PD FSE images. (C) Sagittal FS PD FSE image. |
![]() FIGURE 9.86 ● Flexor digitorum superficialis (A) and pronator teres muscle (B) strain in association with acute proximal tear of the anterior bundle of the MCL. Coronal FS PD FSE images. |
![]() FIGURE 9.87 ● Flexor digitorum superficialis strain in association with an anterior bundle tear of the MCL. Coronal FS PD FSE image. |
After contrast administration, T1-weighted images demonstrate enhancement of the inflamed or injured MCL, and MR arthrograms display the following:
Extravasation of contrast in complete ruptures
Extension of contrast around the sublime tubercle in distal deep partial tears
“T sign” on coronal images in distal partial tears that spare the superficial fibers (caused by fluid tracking between the torn fibers and the medial aspect of the coronoid process)
Treatment
Patients with symptomatic MCL insufficiency usually are treated with reconstruction using a palmaris tendon graft (Fig. 9.88). Graft failure, although unusual, can be evaluated with MR imaging. Other complications include damage to the ulnar nerve and medial antebrachial cutaneous nerve. Lateral compartment bone contusions often are seen in association with acute MCL tears and may provide useful confirmation of recent lateral compartment impaction secondary to valgus instability.
Medial Epicondylitis
Pearls and Pitfalls
Medial Epicondylitis
This is a midlife overuse injury.
There is a frequent association with ulnar neuritis.
Medial epicondylitis is a degenerative disorder, similar to supraspinatus and patellar tendinosis, and there is no inflammatory response.
Medial epicondylitis, also known as golfer’s elbow, pitcher’s elbow, or medial tennis elbow, is less common than lateral epicondylitis.34,35 It is caused by degeneration of the common flexor tendon secondary to overload of the flexor-pronator muscle group that arises from the medial epicondyle (Fig. 9.89).36,37,38 The most common location is the interval between the pronator teres (Fig. 9.90) and flexor carpi radialis. The spectrum of damage to the muscle-tendon unit includes muscle strain injury, tendon degeneration (tendinosis), and macroscopic tendon disruption (Fig. 9.91). Caused by chronic valgus stress, it occurs mainly in individuals 30 to 50 years of age, with an equal sex distribution. In golfer’s elbow it is seen most commonly in the dominant hand, and it is also associated with throwing sports such as baseball.
Although tears of a normal muscle-tendon unit may occur at the myotendinous junction, failure of a muscle-tendon unit through an area of tendinosis is a much more common clinical entity.39 Tendon degeneration, or tendinosis, is common about the elbow,34,38 and concurrent medial and lateral epicondylitis secondary to flexor and extensor tendinosis is not unusual.
MR Appearance
MR imaging is useful for detecting and characterizing acute muscle injury as well as for following its resolution.40 Coronal FS sequences are the most sensitive for detecting muscle pathology. The common flexor tendon and MCL should be evaluated carefully for associated tearing when there is evidence of medial muscle strain injury on MR images (see Figs. 9.89 and 9.91). However, abnormal signal intensity within a muscle may simply be due to the effect of a therapeutic injection for epicondylitis, rather than an indication of muscle strain. Increased signal intensity on FS PD FSE sequences may be seen after an intramuscular injection and may persist for as long as a month.41 Ideally, therefore, steroid injections should be administered after MR imaging to avoid the confounding appearance of the injection on the structures about the elbow.
With MR imaging it is possible to differentiate tendinosis (Fig. 9.92) (secondary to degeneration, microscopic partial tearing, and repair) from macroscopic partial tearing or complete rupture (Fig. 9.93). This distinction is made by identifying fluid signal intensity delineating the presence or absence of tendon fibers on FS PD FSE images. The appearance of medial and lateral epicondylitis about the elbow is similar to the appearance of other common degenerative tendinopathies that involve the attachment of tendons to bone. Similar MR criteria can be used to evaluate the common flexor and common extensor tendons in the elbow, the supraspinatus tendon in the shoulder, the patellar tendon in the knee, and the plantar fascia in the foot. In each of these conditions, there is degenerative tendinosis and a failed healing response that precedes rupture.39,42,43
The best MR diagnostic clue is thickening and increased signal intensity within the flexor-pronator group (FPG) at the level of the medial epicondyle. In medial tension overload there is often increased signal intensity within the common flexor tendon origin at the medial epicondyle, and the tendon is often thickened as well. Discrete to large irregular tears may also be seen, in which case there is fluid signal intensity within the tendon. Associated common flexor muscle belly strains are also characterized by increased signal intensity. Lateral compression may cause osteochondral injuries of the humeral capitellum with chondromalacia and underlying bone marrow edema or cysts. Loose bodies may also be seen. In skeletally immature individuals there may be avulsion of the medial epicondyle (see discussion of Little Leaguer’s elbow below).
Characteristic MR findings include:
Intermediate-signal-intensity tendon with or without reactive epicondylar edema (hypointense on T1- or PD-weighted images and hyperintense on FS PD FSE images)
Partial MCL tear
Synovitis, intermediate to hyperintense on FS PD FSE images
Thickened tendon (see Fig. 9.92)
Discontinuous fibers possibly seen in tendon tear, hyperintense MCL on FS PD FSE images if the tear is acute (see Fig. 9.93)
Flexor-pronator group (FPG) swelling and edema
Associated lateral impaction injury (see Fig. 9.93B) with subchondral cysts (in chronic injury), subchondral sclerosis, and loose bodies (especially in osteoarthritis)
Associated ulnar neuritis, indicated by hyperintensity on FS PD FSE images and thickening of the nerve, usually within the cubital tunnel
![]() FIGURE 9.92 ● Common flexor tendinosis characterized by tendon degeneration. Coronal FS PD FSE image. |
Treatment
Treatment for medial epicondylitis may be conservative, including limitation of activity, physical therapy, and steroid injections, or surgical. Surgical options include release of the common flexor origin and extirpation of affected tissue; tendon repair; and, in the presence of ulnar neuritis and common flexor tendinosis, transposition or decompression of the ulnar nerve. MR imaging facilitates surgical planning by delineating and grading tears of the common flexor tendon as well as evaluating the underlying MCL and adjacent ulnar nerve. Coronal, sagittal, and axial plane sequences are all useful for assessing the degree of tendon injury. Ulnar neuritis associated with common flexor tendinosis (found in 25% to 50% of patients undergoing surgery for medial epicondylitis) may be difficult to identify clinically. Patients with concomitant ulnar neuropathy have a significantly poorer prognosis after surgery than do patients with isolated medial epicondylitis44,45 and, as mentioned, require transposition or decompression of the ulnar nerve in addition to débridement and repair of the abnormal flexor tendon.37,44,45,46 The availability of improved preoperative information from MR studies may reduce the need for extensive surgical exploration in cases in which the MCL is clearly intact on MR imaging. In addition, MR imaging may be useful for problem solving in patients who develop recurrent symptoms after surgery for medial or lateral epicondylitis.
Little Leaguer’s Elbow
In skeletally immature individuals, valgus stress overload may cause the flexor muscle-tendon unit to fail at the unfused apophysis of the medial epicondyle, causing injury variously called Little Leaguer’s elbow (Fig. 9.94), extension overload injury, medial epicondylar avulsion, medial epicondylitis, medial epicondyle apophysitis, and thrower’s elbow. The most commonly used of these terms, Little Leaguer’s elbow, is derived from the fact that stress fracture, avulsion, or delayed closure of the medial epicondylar apophysis not infrequently occurs in young baseball players secondary to overuse.47 The valgus stress results in tension injuries on the medial aspect of the elbow and compressive injuries on the lateral aspect.
MR Appearance
With MR imaging, particularly FS sequences, it is possible to identify soft-tissue or marrow edema about the medial epicondylar apophysis, useful in detecting these injuries before complete avulsion and displacement occur.6 The characteristic appearance of the medial muscle-tendon unit varies depending on the age of the patient. In a child, the ossification nucleus of the medial epicondyle may be displaced into the joint (Fig. 9.95), whereas in an adolescent there is more likely to be a crescentic flake of bone avulsed from the margin of the epicondyle (Fig. 9.96).
Key MR findings include:
A zone of separation through the epicondylar region with or without epicondylar edema
Presence of a discontinuous MCL, indicating a tear
Variable signal within the fragment, depending on whether there is sclerosis or edema
Marrow edema in the parent bone (e.g., humerus)
Fluid signal in MCL tears (tears may be complete or incomplete)
Although STIR images have a lower spatial resolution, they have a high sensitivity for edema, and characteristic findings include high signal within the fragment and in the parent bone and high-signal edema in the MCL complex, with or without defects.
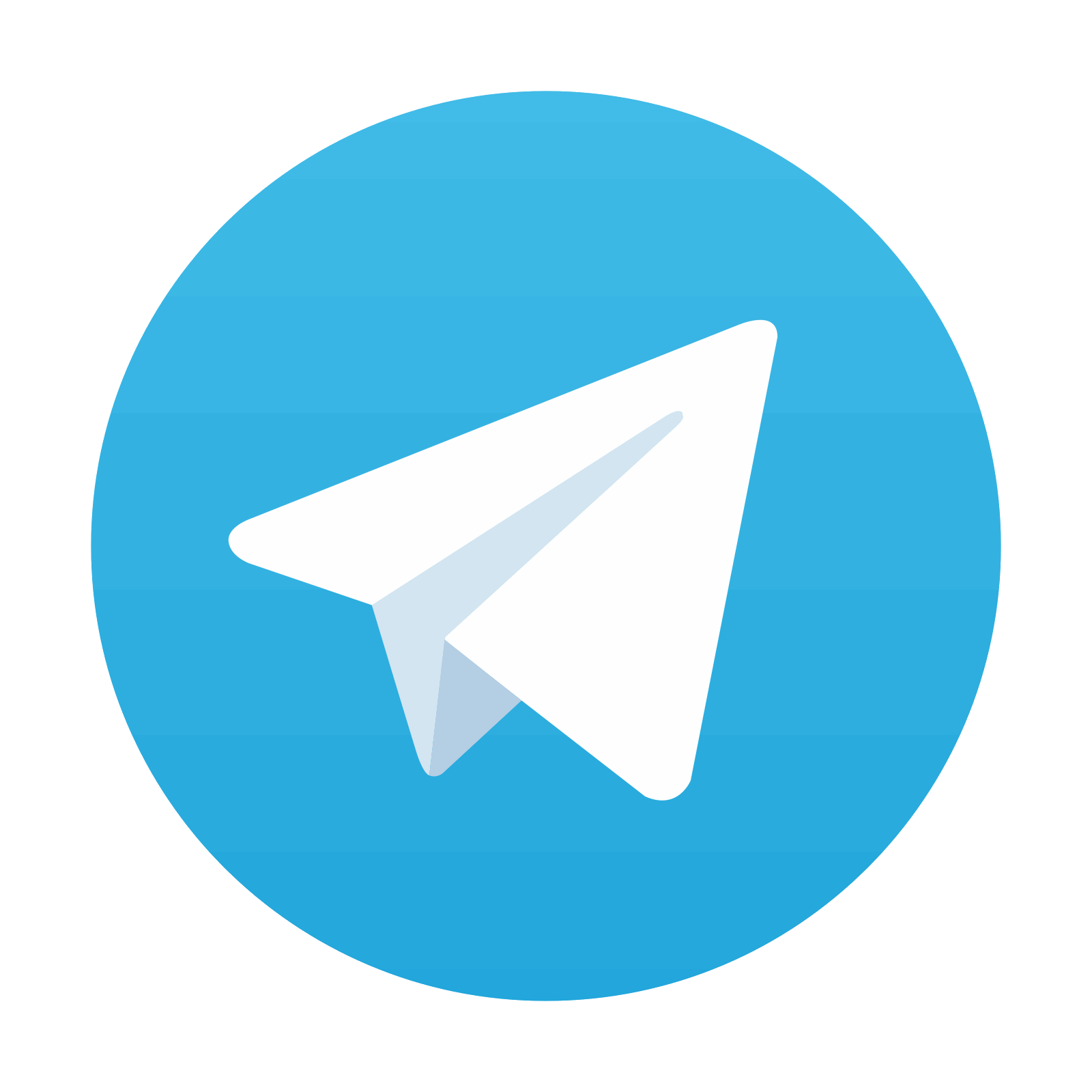
Stay updated, free articles. Join our Telegram channel

Full access? Get Clinical Tree
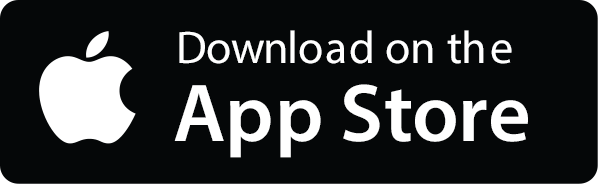
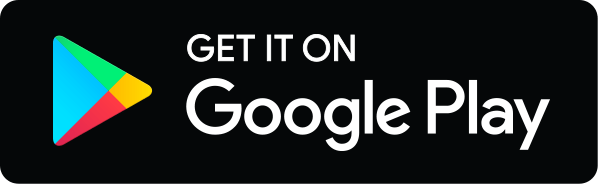
