The Dislocated Knee
Gregory C. Fanelli
John D. Beck
John T. Riehl
Mark E. McKenna
Craig J. Edson
Knee dislocation occurs when there is complete disruption of the femoral-tibial articulation. These injuries are true orthopedic emergencies. Prompt recognition is essential to avoid catastrophic complications. The incidence of knee dislocation is thought to be around 1 in 100,000 of all hospital admissions (1). Specific studies report varied incidence from low (2, 3) to considerably higher (4). Previous studies on incidence that have been largely based on radiographic or clinical diagnosis in a hospital setting, however, may be under representative of the true incidence as many knee injuries spontaneously reduce in the field. Therefore, any multiligament-injured knee should be treated as a dislocation in the acute setting because the knee may have spontaneously reduced prior to radiographic examination.
Most knee dislocations involve tears of the central pivot, including both the anterior cruciate ligament (ACL) and the posterior cruciate ligament (PCL) along with one or both of the collateral ligaments. Significant capsular injury can further add to instability. In addition to ligamentous and capsular injury, meniscal and cartilaginous lesions may be present as well. Associated fractures, compartment syndrome, and trauma to other parts of the body are not uncommon with these often high-energy mechanisms (2, 5, 6, 7, 8 and 9).
Neurovascular injury occurs relatively frequently in the multiple ligament-injured knee. Vascular injuries are found in approximately 16% to 64% of these injuries (3, 6, 7, 10, 11). A detailed neurovascular examination is essential, both pre- and postreduction. Any suspected or confirmed arterial injuries warrant prompt attention, as these injuries carry a significant risk of amputation (10, 11, 12, 13, 14 and 15).
Historically, nonoperative treatment consisting of immobilization was the treatment of choice for multiligament injuries to the knee (16, 17). With advances in arthroscopic techniques of ligament reconstruction, nonoperative treatment is frequently limited to medically unstable patients or those with low functional demands.
ANATOMY AND BIOMECHANICS OF THE KNEE
The primary plane of motion in the knee is in the sagittal plane. Some rotation of the tibia on the femur occurs normally as well. Normal range of motion (ROM) is from 0° of extension (or a few degrees of hyperextension) to approximately 140° of flexion. Internal and external rotations are typically 10° in either direction, and external rotation in full extension leads to the “screw home” mechanism. This allows the knee to “lock” in full extension and reduces the work of the quadriceps during standing. Stability of the knee is maintained in part by the bony articulation between the femoral condyles and the tibial plateau. Medial and lateral menisci increase the contact surfaces and thus increase static stability to the joint.
The osseous anatomy of the knee consists of the articulations of the proximal tibia, the distal femur, and the patella. The tibial plateau has an approximate 10° posterior slope. The medial plateau is slightly concave, whereas the lateral plateau has a more rounded appearance. Although, the tibial plateau is somewhat flattened relative to the curved distal femur, congruency is maintained within the knee as the menisci help to increase conformity within the tibiofemoral articulation. The tibial spines separate the medial and lateral plateaus and serve as attachments for the menisci and cruciate ligaments.
The distal femur is divided into medial and lateral condyles. The size of the condyles are asymmetric with the distal femur, forming a trapezoidal shape (18). The medial condyle projects more distally, whereas the lateral condyle projects more anteriorly. The condyles are separated by the trochlear groove, which contributes to the patellofemoral articulation.
The patella is the largest sesamoid bone in the body and serves as a fulcrum for the extensor mechanism as well as providing a protective surface for the anterior aspect of the knee.
Ligamentous Anatomy
The four major ligamentous stabilizers of the knee include the ACL, the PCL, the medial collateral ligament (MCL), and the lateral collateral ligament (LCL). In addition, the posteromedial corner (PMC) and posterolateral corner (PLC) of the knee are important structures that also contribute to knee stability. If injuries to these two structures are not recognized or addressed at the time of definitive treatment of the four main stabilizers, undue stresses may be placed on repairs/reconstructions and lead to adverse outcomes.
The primary function of the ACL is to resist anterior translation of the tibia relative to the distal femur. In addition, it serves as a secondary adjunct to varus and valgus stability in full extension. The ACL originates on the posteromedial lateral femoral condyle and courses anteriorly and distally to insert in the depression in front of and lateral to the anterior tibial spine (19). Two anatomic bundles make up the ACL. The posterolateral bundle is tight in extension, whereas the anteromedial bundle is tight in flexion (20). The ACL is typically 35 to 40 mm in length and 10 to 12 mm in width (21). It is an intra-articular structure, yet it has its own synovial membrane. It receives its blood supply from the middle geniculate artery and is innervated by the posterior articular nerve, a branch of the tibial nerve (22).
The PCL resists posterior translation of the tibia and is a secondary restraint to tibial external rotation. The PCL has a broad femoral origin on the posterolateral aspect of the medial femoral condyle and it inserts centrally on the posterior tibial plateau. It is an intra-articular structure, but is also encompassed by its own synovial sheath. The posteromedial bundle of the PCL is tight in extension, whereas the anterolateral bundle is under more tension in flexion (23). These bundles are supplemented by the posterior meniscofemoral ligaments. The average length of the PCL is 38 mm and its width is 13 mm (23, 24 and 25). The vascularity of the PCL is supplied by the middle geniculate artery, and it is innervated by nerve fibers from the popliteal plexus from the tibial and obturator nerves (26).
The MCL and the PMC are the primary restraints to valgus stress in the knee. The medial side of the knee can be divided into thirds from anterior to posterior. The anterior third consists of capsular ligaments covered by the extensor retinaculum. The middle third contains the superficial and deep MCL. The PMC occupies the posterior third and includes the posterior oblique ligament, the posterior horn of the medial meniscus, and the termination of the semimembranosus (27). Alternatively, the anatomy of the medial side of the knee has been described by Warren and Marshall in terms of layers. The most superficial layer is the sartorial fascia. The second layer consists of the superficial MCL. The deep MCL and the medial joint capsule are found in layer three (28).
The superficial MCL is the primary restraint to valgus stress of the knee at 30° of knee flexion. Its origin is on the medial epicondyle of the distal femur, and it inserts just posterior to the insertion of the pes anserinus and just anterior to the adductor tubercle. It inserts on the anteromedial tibia approximately 5 cm below the joint line (29). The posterior oblique ligament, semimembranosus, and oblique popliteal ligament resist valgus stress in full extension as well as providing contribution to anteromedial rotatory stability (30).
The lateral side of the knee has also been divided into layers (31). The biceps femoris and the iliotibial band make up the most superficial layer. The peroneal nerve lies deep to the biceps at the level of the distal femoral condyle. The middle layer consists of the patellar retinaculum anteriorly and the patellofemoral ligaments posteriorly. The deep layer, layer III, consists of the LCL, popliteal tendon, popliteofibular ligament, fabellofibular ligament, arcuate ligament, and lateral joint capsule.
The LCL is the primary restraint to varus stress with the knee in 30° of flexion. In an anatomic study performed by LaPrade et al., the LCL was found to originate on average 1.4 mm proximal and 3.1 mm posterior to the lateral epicondyle of the femur in a bony depression. It attaches on the fibular head, 28.4 mm distal and 8.2 mm posterior to the anterior superior margin of the fibula, again in a bony depression (32). The remaining structures in layer III make up the PLC. The PLC provides static support to resist posterior translation of the tibia as well as external rotation and varus angulation. The popliteus tendon attaches to the femur anterior to the LCL, with an attachment on the posteromedial proximal tibia.
Neurovascular Anatomy
The neurovascular bundle within the popliteal fossa is at significant risk both during and in the period immediately following knee dislocations. The risk of injury to the neurovascular structures in this area can be explained by some of their anatomic features.
The popliteal fossa is bordered by the semimembranosus muscle superomedially, the biceps femoris tendon at its superior lateral border, and the two heads of the gastrocnemius muscle inferiorly. Within the popliteal fossa, the popliteal artery and vein are separated by a thin layer of fat from the underlying posterior joint capsule. Traversing through the popliteal fossa, from deep to superficial, are the popliteal artery, popliteal vein, and posterior tibial nerve. With the knee in full extension, the popliteal fascia is tensioned, making palpation of the popliteal artery difficult. Palpation of the pulse in this region is therefore best performed with the knee in slight flexion. Proximally, the popliteal artery emerges from the adductor hiatus and is tethered to this fibrous tunnel. Distally, the popliteal artery is also relatively immobile as it enters another fibrous canal deep to the soleus. These two somewhat immobile points leave the popliteal artery vulnerable to injury when the knee is dislocated. Superior, inferior, and middle geniculate branches stem from the popliteal artery, but are unable to maintain adequate collateral circulation in the event of a vascular injury.
The sciatic nerve enters the popliteal space from between and deep to the semitendinosus and the long head of the biceps, at a variable level it divides into the tibial and common peroneal nerves. The common peroneal nerve follows the lower edge of the biceps toward the fibula crossing superficially to the lateral head of the gastrocnemius. The tibial nerve continues down the middle of the popliteal fossa and gives off muscular branches to the plantaris and gastrocnemius muscles. The common
peroneal nerve then courses distally around the fibular head to innervate the anterior and lateral compartments of the lower leg. The course of the peroneal nerve makes it more susceptible to injury during knee dislocation than the tibial nerve, explaining the significant increase in its observed incidence (14, 17, 33).
peroneal nerve then courses distally around the fibular head to innervate the anterior and lateral compartments of the lower leg. The course of the peroneal nerve makes it more susceptible to injury during knee dislocation than the tibial nerve, explaining the significant increase in its observed incidence (14, 17, 33).
CLASSIFICATION OF KNEE DISLOCATIONS
Numerous classification systems exist to describe knee dislocations. The most commonly, the direction of displacement of the proximal tibia relative to the distal femur is used to describe the injury. However, this system does not account for spontaneously reduced dislocations and may fail to recognize other important considerations in the multiple ligament-injured knee. Mechanism of injury, the presence or absence of open wounds, the degree of displacement, and the status of the neurovascular structures are additional aspects of the injury that may be used to help describe knee dislocations. In practice, all of the above characteristics are helpful in the classification of the multiple ligament-injured knee and can assist in determining optimum treatment.
The directional classification of knee dislocations is based on the position of the tibia relative to the distal femur. Anterior dislocations occur after a hyperextension injury greater than 30° (2) and are the most common directional dislocation. Posterior knee dislocations occur in 25% of all knee dislocations and typically result from a posteriorly directed force applied to the proximal tibia. Lateral, medial, and rotatory dislocations have also been described (10). The direction of dislocation may raise the suspicion of the treating physician to certain associated injuries. For example, intimal tears of the popliteal artery are more common with anterior dislocation, whereas popliteal artery transaction is more likely to occur with posterior dislocation.
High-energy dislocations typically occur following motor vehicle collisions and falls from height. Low-energy injuries typically refer to those that occur during athletic activities (34). An ultra-low-energy dislocation has been described in morbidly obese patients that sustain severe ligamentous injury following seemingly trivial trauma (6, 35).
Schenck developed an anatomic classification system that classifies knee dislocations on the basis of the specific structures about the knee that are compromised (Table 78.1) (36). The dislocation is classified in terms of the ligamentous injury pattern, and the letter C designates a circulatory injury, whereas the letter N indicates neurologic injury. It has been used by some authors to direct treatment and predict outcome.
INJURY MECHANISMS
Knee dislocations can occur as the result of high-energy, low-energy, or even “ultra-low-energy” injuries. The direction of the dislocation as well as the injury pattern is dependent upon both the position of the knee at the time of injury and the direction of the force applied. Motor vehicle collisions, falls from height, farm injuries, and industrial accidents make up most of the high-energy knee dislocations. Low-energy knee dislocations typically occur from sports-related injuries and appear to have a lower incidence of associated neurovascular compromise. Ultra-low-energy dislocations have been described as well where seemingly trivial trauma has caused knee dislocations with associated neurovascular injury in morbidly obese patients (35).
Table 78.1 Modified Schenck classification of knee dislocation | ||||||||||||||||||
---|---|---|---|---|---|---|---|---|---|---|---|---|---|---|---|---|---|---|
|
Anterior dislocations typically result from a hyperextension mechanism. As Kennedy (2) applied a hyperextension force to the knee in 12 cadaveric specimen, he noted tearing of the posterior capsule of the knee followed by cruciate rupture. This occurred on average at 30° of hyperextension and produced anterior dislocation of the tibia. As hyperextension continued to an average angle of 50°, the popliteal artery was noted to be placed on considerable stretch.
Posterior dislocations are the result of a significant posteriorly directed force applied to the proximal tibia and can be associated with substantial damage to the extensor mechanism (2). This is one possible mechanism in highenergy motor vehicle collisions and may be the result of a dashboard injury. In a low-energy injury, a fall landing on the tibial tubercle with a plantar-flexed ankle may produce a posterior knee dislocation as well.
Lateral and medial dislocations typically result from severe varus and valgus stresses, respectively. Rotational forces produce a rotational type of dislocation. Quinlan and Sharrard (9) described the mechanism for
posterolateral dislocation to be a flexed knee with “severe abduction-medial rotation violence to the knee while the limb was not bearing weight.”
posterolateral dislocation to be a flexed knee with “severe abduction-medial rotation violence to the knee while the limb was not bearing weight.”
ASSOCIATED INJURIES
As stated previously, most knee dislocations involve tears of the central pivot, along with one or both of the collateral ligaments. Additional intra-articular pathology such as chondral or meniscal injury is not uncommon. Capsular injuries prevent arthroscopic reconstruction of cruciate ligaments within the first several days postinjury and may also cause swelling to become severe within the first 48 hours.
Swelling and/or vascular injury may lead to compartment syndrome, especially when fractures are present. Injuries to other body regions also are not uncommon. Neurologic and vascular injuries will be covered later in detail.
INITIAL EVALUATION OF THE MULTIPLE LIGAMENT-INJURED KNEE
General Considerations
Initial management of knee dislocations demands a comprehensive and systematic approach to facilitate accurate and efficient diagnosis and treatment. Individuals sustaining high-energy trauma should be treated following standard advanced trauma life support (ATLS) protocols with assessment to screen for orthopedic emergencies including: vascular disruption, open wound, compartment syndrome, or an irreducible joint (37). A secondary survey should include a comprehensive physical examination supplemented by appropriate ancillary studies to formulate a treatment plan.
Patients with obvious deformity or varus/valgus malalignment can be rapidly diagnosed with a knee dislocation. Uncontained heamarthrosis, abrasions or contusions of the knee, gross crepitus, or laxity should alert the examiner to the possibility of a potential knee dislocation (38). These findings are important to recognize as 34% to 50% of knee dislocations present to the emergency department in a reduced position (13, 17). In addition, any patient with two or more ligamentous injuries of the knee should be treated as a potential knee dislocation (2, 6, 39, 40). The importance of accurate and timely diagnosis of knee dislocation lies in the recognition of potential vascular injury as the morbidity associated with delayed revascularization is extremely high (10, 41).
Physical Examination
Following the initiation of ATLS protocols when appropriate, emergency department evaluation should include a thorough, but efficient, history composed of: mechanism of injury, position of limb at time of injury, and manipulation of the limb prior to arrival. This history can provide clues to the direction of dislocation and energy of injury. Physical examination should start with inspection of the extremity to evaluate resting position and gross alignment. Determination of distal vascularity is of foremost concern and should be assessed with palpation/Doppler assessment of dorsalis pedis and posterior tibial pulses, capillary refill, skin color, and skin temperature. Hard signs of vascular injury including active bleeding, distal ischemia, expanding heamatoma, and popliteal bruit warrant emergent vascular surgery consultation. In the setting of knee dislocation with asymmetric or absent pedal pulses, immediate reduction may be required to restore distal blood flow (13). If symmetric pulses are present, orthogonal X-rays of the knee should be obtained emergently prior to manipulation and reduction should be attempted unless a dimple sign is present. Dimpling of the skin on the anterior-medial knee is pathoneumonic for posterolateral dislocation with the medial femoral condyle buttonholing through the anteriomedial joint capsule. Attempted closed reduction of these dislocations is associated with a high rate of skin necrosis and therefore closed reduction should not be attempted if a “dimple sign” is present (42).
Reduction is attempted with longitudinal traction applied to the tibia with manipulation of the proximal tibia according to the direction of the dislocation. Following reduction, a repeat neurovascular examination should be performed and documented. Postreduction examination should include inspection of the skin for abrasions, ecchymosis, swelling, open wounds, and skin dimpling, all of which provide information about underlying pathology. Diffuse swelling with uncontained heamarthrosis on the medial and lateral knee suggest major disruption of the joint capsule and should raise suspension of spontaneously reduced knee dislocation, even in the setting of normal X-rays (38).
A complete neurologic examination is an essential aspect of the initial evaluation and should be performed both prior to and after a closed reduction. Motor and sensory examination of the superficial peroneal, deep peroneal, and tibial nerve distributions should each be evaluated and documented. Sequential neurologic examinations should be performed over the first 48 hours, and repeated at 1 and 2 weeks as motor grades are often acutely reduced after knee dislocation secondary to pain alone (33). On serial examination, the presence of progressive deterioration of neurologic function raises the suspicion of an impending compartment syndrome (43).
In the acute setting, pain and swelling can limit the ability to accurately assess the extent of ligamentous injury in a conscious patient. Examination should be performed in a careful and controlled manner to avoid iatrogenic injury. A stabilized Lachman test in which the examiner’s thigh is placed under the injured knee allows for relatively pain-free evaluation of anterior endpoint. The most sensitive test for determining ACL and PCL deficiency are
Lachman test at 20° of flexion and posterior drawer at 90° of flexion, respectively. The collateral ligaments are evaluated at 30° and at full extension by applying varus or valgus stress. Gross laxity at full extension implies disruption of the collateral ligament, one or more of the cruciate ligaments, and associated capsule. A more detailed ligamentous examination typically requires conscious sedation or general anesthesia.
Lachman test at 20° of flexion and posterior drawer at 90° of flexion, respectively. The collateral ligaments are evaluated at 30° and at full extension by applying varus or valgus stress. Gross laxity at full extension implies disruption of the collateral ligament, one or more of the cruciate ligaments, and associated capsule. A more detailed ligamentous examination typically requires conscious sedation or general anesthesia.
Under anesthesia, a complete examination can be preformed that eliminates confounding variables such as muscular guarding. Evaluation of the PCL includes measurement of tibial step-off compared with contralateral extremity, posterior drawer, and reverse pivot-shift. Lachman, pivot-shift, and anterior drawer are preformed for ACL competence. The posterior lateral and posterior medial corners can be examined with posteriolateal and posteriomedial drawers. The dial test can be used to differentiate PCL and PLC injuries. In addition, the supine heel lift test can evaluate for recurvatum secondary to PLC, PMC, PCL, and posterior capsule deficiency. Finally, varus and valgus stress can be applied to the knee in hyperextension, neutral, and at 30° of flexion.
Imaging Studies
Plain anteroposterior (AP) and lateral radiographs should be obtained in all cases of suspected knee dislocations. Initial X-rays will confirm the direction of dislocation to aid in planning reduction as well as identify associated osseous injuries. Occasionally, tibiofemoral widening on AP X-ray in a swollen knee is the only radiographic sign of a spontaneously reduced knee dislocation. Fracture patterns that suggest ligamentous injury include fibular head avulsion, tibial tubercle avulsion, and Segond fractures. A fibular head fracture can represent disruption of the LCL, popliteofibular ligament, or biceps femoris insertion. A Segond fracture is an avulsion fracture of the lateral tibial plateau associated with a torn ACL (37). Following manipulation, repeat X-rays are mandatory to confirm reduction and evaluate for residual subluxation.
Arteriography remains the gold standard for assessment of vascular injury, following knee dislocation. However, the use of routine angiography for all knee dislocations has come into question. An angiogram and vascular consult is indicated in any patient with signs of vascular compromise including diminished pulses, absent pulses, color or temperature changes of the involved limb or ankle-brachial index (ABI) less than 0.90. Patients with hard signs of vascular injury (active bleeding, distal ischemia following reduction, expanding hematoma, and popliteal bruit) require an emergent vascular surgery consult. In this situation, an angiography should be performed in the OR as angiography in an angiography suite delays the time to repair by an average of 3 hours (44). Recently, the use of MR angiography has been suggested as an alternative to arteriography to evaluate for vascular injury in the acute setting. MRA is less invasive than standard arteriography and avoids potential complications associated with contrast and arterial punctures including renal failure, allergic reaction, and iatrogenic vascular injury. The potential benefits of MRA have been well established in other settings (45, 46). Early data on MRA following knee dislocation have been promising (47), but are still limited in comparison with standard angiography.
Following the acute management of the dislocated knee MRI should be obtained to guide operative management. MRI in the acute setting can determine the presence and extent of ligamentous pathology, avulsion versus intrasubstance tear, as well as concomitant meniscal and articular cartilage injuries. MRI is the gold standard for evaluation of soft tissue injuries about the knee. Numerous studies have evaluated the sensitivity and specificity of MRI in this setting. In 2008, Bui et al. retrospectively reviewed MRI findings of 20 patients with knee dislocations to compare them with operative findings. They had two false negative interpretations, both involving meniscal tears. In their 20 patients, they had four false positive interpretations in which the ligament was reported as a complete tear and was found to be partial or healed at time of injury. This data was confounded by the time of surgery ranging from 26 to 223 days (48). Twaddle et al. (49) found MRI to be 85% to 100% accurate in predicting the extent of soft tissue injury following knee dislocation (49). In addition, abnormalities of the peroneal nerve can also be identified on MRI. In 2002, Potter et al. (47) retrospectively reviewed 21 knee dislocations and correlated MRI findings with operative examination. In their series, all 10 nerve injuries noted on MRI were confirmed at surgery. As MRI and MRA technology continue to improve, the detail and accuracy provided may allow combined MRI/MRA to supplant arteriography as the gold standard.
Vascular Injuries
The popliteal artery is at risk for injury during knee dislocation because of its anatomic location. Proximally, the popliteal artery is tethered at Hunter’s canal, and distally it is constrained at the soleal arch (50). This anatomic tethering, combined with the limited collateral circulation of the knee, leads to the high incidence of vascular injury following knee dislocation, ranging from 16% to 64% in the literature (3, 7). The incidence of arterial injury is greatest with anterior and posterior dislocations, 39% and 44%, respectively (10). A full spectrum of vascular injuries can be encountered depending on the mechanism of injury, including transection, contusion, injury to the intimal and medial layers without loss of continuity, and thrombus formation (51). Posterior dislocations typically result in complete transection of the popliteal artery, whereas anterior dislocations produce a traction injury to the artery resulting in extensive intimal injury/tear (10). Initially, it was thought arterial injury was less common with low-velocity knee dislocations (LVKD). Recent studies reported a 58% incidence of arterial injury in obese
patients with LVKD. This patient population has a 17% risk of amputation even after repair (35, 52, 53 and 54). With the epidemic of obesity in America and the difficulty of examination, the evaluation of this patient population must be done carefully to ensure a knee dislocation with a potential arterial injury are not missed.
patients with LVKD. This patient population has a 17% risk of amputation even after repair (35, 52, 53 and 54). With the epidemic of obesity in America and the difficulty of examination, the evaluation of this patient population must be done carefully to ensure a knee dislocation with a potential arterial injury are not missed.
Regardless of the direction of the dislocation, mechanism of energy, or velocity of injury, vascular injury should always be suspected and evaluated. Delay in diagnosis of vascular injury requiring repair is associated with an extremely high morbidity. Green and Allen (10) reported an 11% amputation rate if vascular repair was undertaken within 8 hours and an 86% amputation rate if delayed for greater than 8 hours. This data was confirmed by the results of the lower extremity assessment project (LEAP) study. They found an average warm ischemia time for patients with amputation following knee dislocation was 7.25 hours; whereas those not requiring amputation averaged 4.7 hours. They concluded that prolonged warm ischemic time was the major factor in determining amputation (41). This reinforces that prompt and accurate diagnosis of vascular trauma is essential in successful management of knee dislocations.
The diagnosis of vascular injury should be made clinically by a detailed history, physical examination, and appropriate ancillary studies. A pointed history should include any manipulation performed prior to arrival and past or present signs of ischemia (pain, paresthesias, paralysis, pallor, and diminished limb temperature). Physical examination should include assessment of pulses by palpation or Doppler compared with the contralateral extremity, signs of active bleeding, distal ischemia, expanding hematoma, and popliteal bruit or thrill. In any patient without obvious vascular injury, ABI should be preformed. Distal perfusion as well as motor and sensory function should be evaluated and documented. Any abnormalities or asymmetry are concerning for vascular injury and should be further investigated. If obvious vascular injury and limbthreatening ischemia are present immediate vascular consultation and repair is necessary.
It is well known that normal pulses, a warm foot, and brisk capillary refill can be present with arterial injury (43). Consequently, vascular injury can be overlooked at time of initial consultation. Collateral circulation through the superior knee arteries, from the profundus femoral and articular branches off the popliteal artery can preserve the vascular supply to the lower extremity in the initial stage of the injury and distal pulses may still be palpable (55). In addition, intimal flap tears initially are undetectable on physical examination because pulses are normal, but may progress to complete arterial occlusion with thrombus. A missed diagnosis of vascular injury and subsequent delay of arterial repair or bypass leads to a significant increase in morbidity with a high rate of amputation. As a result, many authors initially recommended liberal or mandatory angiographic studies in cases of knee dislocations (56, 57, 58 and 59). The use of routine angiography has been debated in favor of selective angiograms on the basis of clinical examination and ABI. Proponents of routine angiogram emphasize the high morbidity associated with missed vascular injury (Fig. 78.1). They also note that normal pulses, Doppler signals, and capillary refill after initial closed reduction do not rule out a vascular injury that progresses over time, causing late vascular compromise (2).
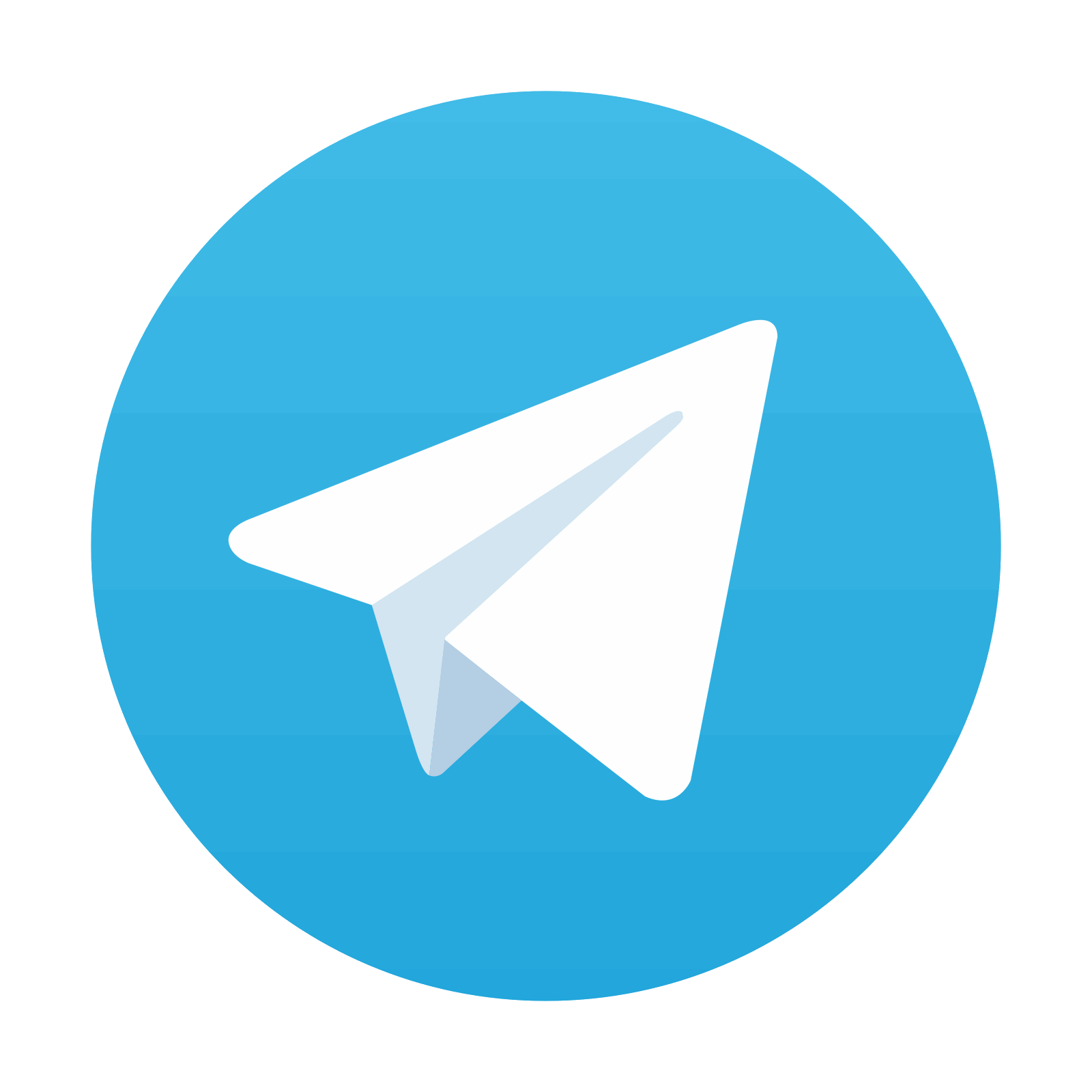
Stay updated, free articles. Join our Telegram channel

Full access? Get Clinical Tree
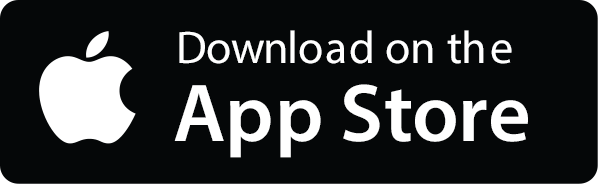
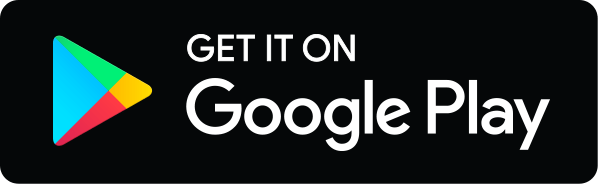