The Ankle and Foot
David W. Stoller
Richard D. Ferkel
Standard radiographic evaluation of the ankle joint requires anteroposterior, lateral, and mortise radiographs. In patients with foot trauma, an additional oblique view may be obtained. Less frequently, arthrography and tomography may be used, primarily in the evaluation of ligamentous tears and articular cartilage defects. In tarsal coalitions and sustentacular trauma, computed tomography (CT) scans have been used to delineate talocalcaneal, transverse tarsal, and tibiotalar joint anatomy.1 CT is limited, however, to the specific plane of section (i.e., axial or angled coronal) and is dependent on reformatted images for visualization in the other orthogonal planes. Magnetic resonance (MR) imaging of the ankle and foot provides high tissue contrast and excellent spatial resolution, affording superior depiction of complex soft-tissue anatomy (e.g., muscles, ligaments, tendons, and fibrous coalitions).2–11 In addition, marrow and cortical bone definition permit increased sensitivity in the detection of fractures, cysts, inflammatory and infectious conditions, and trauma.12 The unique ability of MR imaging to directly display hyaline articular cartilage has made it valuable in assessing arthritis and osteochondral lesions, and in identifying intra-articular loose bodies.
Imaging Protocols for the Ankle and Foot
Pearls and Pitfalls
Imaging Protocols
FS PD FSE sequences are performed in all three orthogonal or orthogonal-oblique planes.
Decreasing the FOV or increasing the resolution in the coronal plane allows optimal separation of the distal tibial and talar dome chondral surfaces.
High-resolution anatomic images of the ankle and foot are obtained with a dedicated extremity surface coil (quadrature
or phased-array design), using a 12- to 14-cm field of view (FOV) and a 512 × 256 or 256 × 256 acquisition matrix. Routine protocols for evaluation include:
or phased-array design), using a 12- to 14-cm field of view (FOV) and a 512 × 256 or 256 × 256 acquisition matrix. Routine protocols for evaluation include:
T1- or proton density (PD)-weighted axial, sagittal, and coronal images
Fat-suppressed PD-weighted fast spin-echo sequences (FS PD FSE) in all three orthogonal planes (Fig. 5.1)
Thin (i.e., 2 to 3 mm) sections
Effective T2*-weighted contrast can be generated with gradient-echo (GRE) techniques using a partial flip angle of less than 90° (20° to 30°) and is used when FS is suboptimal or in the evaluation of the neuropathic foot.
To image the forefoot, the patient is placed in a prone position to orient the long axis of the foot with the orthogonal axial imaging plane or with the oblique image prescriptions parallel with the long axis of the metatarsals and cuneiform bones. Surface coils, which allow proper placement of the foot with the patient in the supine position, can also be used to evaluate the long axis of the forefoot without drop-off of signal intensity. STIR and fast STIR sequences are useful in identifying forefoot lesions in the coronal plane and stress fractures in the sagittal plane when FS PD FSE images are inhomogeneous with respect to fat suppression.
By placing both legs within the circular extremity or torso coil, comparison with the contralateral ankle and foot can be achieved. Alternatively, when smaller FOVs are needed, the extremities can be imaged one at a time by repositioning the surface coil. The foot is usually placed in a neutral position, although partial plantarflexion may be useful when comparing MR images to a CT ankle examination that was performed in 45° of tibiotalar angulation. A combination foot and ankle/knee coil provides adequate anatomic coverage for imaging the toes and distal metatarsals. Thin (3 mm or less) coronal T1-weighted and STIR images are most useful.
Intravenous contrast, in association with FS sequences, is useful for the evaluation of Morton’s neuroma, inflammatory synovial processes, and certain tendon conditions (partial tears, the healing process, and infiltrative disorders). Intravenous and intra-articular contrast (MR arthrography) has been used on a limited basis in the study of osteochondral lesions and other intra-articular pathology. Articular cartilage is evaluated using a variety of techniques, including FD PD FSE, FSE STIR, and MR arthrography. FS PD FSE and STIR contrast are the more frequently used sequences in characterizing muscle injuries.
Related Muscles
The anterior muscles of the leg are the tibialis anterior (Fig. 5.2), the extensor hallucis longus (Fig. 5.3), the extensor digitorum longus (Fig. 5.4) and the peroneus tertius (Fig. 5.5).
The posterior muscles of the leg include a superficial group and deep group. The superficial group is represented by the gastrocnemius (Fig. 5.6), the soleus (Fig. 5.7), and plantaris (Fig. 5.8).
The deep group of posterior leg muscles comprises the popliteus (see discussion in Chapter 4 on the knee), the flexor hallucis longus (Fig. 5.9), the flexor digitorum longus (Fig. 5.10), and the tibialis posterior (Fig. 5.11).
The lateral muscles of the leg are the peroneus longus (Fig. 5.12) and the peroneus brevis (Fig. 5.13).
The muscles of the foot are the extensor digitorum brevis (Fig. 5.14), the abductor hallucis (Fig. 5.15), the flexor digitorum brevis (Fig. 5.16), the abductor digiti minimi (Fig. 5.17), the quadratus plantae (Fig. 5.18), the lumbricals (Fig. 5.19), the flexor hallucis brevis (Fig. 5.20), the adductor hallucis (Fig. 5.21), the flexor digiti minimi brevis (Fig. 5.22), the dorsal interossei (Fig. 5.23), and the plantar interossei muscles (Fig. 5.24).
![]() FIGURE 5.21 ● ADDUCTOR HALLUCIS The adductor hallucis has two heads and forms a conjoined tendon. The adductor hallucis adducts the great toe and assists in its flexion. |
MR Atlas of the Ankle and Foot
Sagittal Images (Fig. 5.25)
The long axis of the tendons crossing the ankle joint is best seen on sagittal planar images.
Medial Sagittal Images
In the plane of the medial malleolus, the tibialis posterior and flexor digitorum longus tendons are directly posterior to the medial malleolus. The tibialis posterior tendon enters the foot by passing deep to the flexor retinaculum and superior to the sustentaculum tali to its insertion on the tuberosity of the navicular bone. The flexor digitorum longus tendon also enters the foot after passing posterior to the medial malleolus and deep to the flexor retinaculum. This tendon is divided into four segments after crossing the flexor hallucis longus tendon, which contributes slips to the medial two divisions. These segments insert onto the bases of the distal phalanges. The quadratus plantae muscle inserts at the division of the flexor hallucis into four tendons. Distally, each tendon is an origin for the lumbrical muscles.
The deltoid ligament, composed of the tibiocalcaneal, tibionavicular, and anterior and posterior tibiotalar ligaments, appears as a wide band of low signal intensity radiating from the distal tibia (i.e., medial malleolus) to the tuberosity of the navicular bone and the sustentaculum tali. The flexor hallucis longus tendon is located posterior to the tibialis posterior tendon and the flexor digitorum longus. It passes posterior to the medial malleolus, deep to the flexor retinaculum. The low-signal-intensity tendon hugs the posterior talar process and the inferior surface of the sustentaculum tali proximal to its insertion onto the base of the distal phalanx of the great toe.
The plantar flexor digitorum brevis (a first-layer muscle of the sole of the foot) and the quadratus plantae (a second-layer muscle of the sole of the foot) are displayed on medial sagittal images. The adductor hallucis (a first-layer muscle) inserts onto the medial proximal phalanx of the first toe and is seen between the first and second metatarsals on medial sagittal images. The tibialis anterior tendon crosses the dorsal surface of the talus before it inserts on the medial cuneiform bone and the bone of the first metatarsal.
Midplane Sagittal Images
The middle subtalar joint, the tarsal sinus, and the posterior subtalar joint are demonstrated on sagittal images medial to the midsagittal plane. The anterior subtalar joint is shown in the plane of the cuboid and calcaneocuboid joint. The peroneus longus, which extends anteriorly along the lateral inferior surface
of the calcaneus and is inferior to the peroneal tubercle, enters the foot at the lateral inferior margin of the cuboid. The extensor hallucis longus tendon is identified along the dorsum of the foot and inserts onto the distal phalanx of the first toe. The interosseous talocalcaneal ligament, with its associated high-signal-intensity fat, is bordered anteriorly by the anterior process of the calcaneus and posteriorly by the lateral process of the talus. The pre-Achilles fat pad (high signal intensity on T1-weighted sequences) is located directly anterior to the Achilles tendon (low spin density).
of the calcaneus and is inferior to the peroneal tubercle, enters the foot at the lateral inferior margin of the cuboid. The extensor hallucis longus tendon is identified along the dorsum of the foot and inserts onto the distal phalanx of the first toe. The interosseous talocalcaneal ligament, with its associated high-signal-intensity fat, is bordered anteriorly by the anterior process of the calcaneus and posteriorly by the lateral process of the talus. The pre-Achilles fat pad (high signal intensity on T1-weighted sequences) is located directly anterior to the Achilles tendon (low spin density).
Lateral Sagittal Images
In the plane of the fibula, the peroneus brevis and longus tendons pass posterior to the lateral malleolus. The peroneus brevis tendon lies anterior to the peroneus longus and is in direct contact with the lateral malleolus. The peroneus brevis can be followed to its insertion on the base of the fifth metatarsal bone. The peroneus longus tendon disappears inferior and medial to the peroneus brevis tendon and enters the cuboid sulcus. Therefore, it appears shorter than the peroneus brevis tendon on lateral sagittal images.
Coronal Images (Fig. 5.26)
Coronal plane images demonstrate tibiotalar articular surfaces and are also used to assess medial, lateral, and posterior ligaments as well as the tendons.
Posterior Coronal Images
The thick, low-signal-intensity Achilles (calcaneal) tendon is clearly displayed on posterior coronal images. Its attachment to the calcaneal tuberosity can also be observed on these views. The soleus muscle, with its inverted-V-shaped origin from the soleal line of the tibia and posterior fibula, contributes to the calcaneal tendon, along with the gastrocnemius and plantaris. The peroneus brevis and flexor hallucis longus muscles are identified lateral to the soleus muscle, and the peroneal tendons are located inferior to the lateral malleolus. The flexor digitorum longus muscle and tendon cross superficially, in a medial-to-lateral direction, to the tibialis posterior in the distal calf. The tibialis posterior tendon is located medial to the posterior malleolus. The posterior talofibular and inferior tibiofibular ligaments are shown on coronal images at the level of the posterior malleolus and posterior process of the talus. The plantar aponeurosis is superficial to the flexor digitorum brevis muscle, whereas the quadratus plantae muscle lies deep to the flexor digitorum.
Midplane Coronal Images
The calcaneofibular ligament is best visualized at the level of the posterior subtalar joint and lateral malleolus. The lateral process of the talus can be seen in the same sections as the anterior lateral malleolus. The middle subtalar joint is formed by the sustentaculum tali and the inferior medial talar surface. This is the best plane for evaluating talocalcaneal coalitions. The peroneus brevis and longus tendons course laterally, superior and inferior, respectively, to the peroneal groove of the calcaneus. The separate chondral surfaces of the tibial plafond and the talar dome are demonstrated in this plane.
Anterior Coronal Images
The tibiotalar and tibiocalcaneal fibers of the deltoid ligament extend obliquely to the talus and vertically to the sustentaculum tali, respectively. The tibialis posterior tendon is medial to the deltoid ligament and superior to the sustentaculum tali and can be used as a landmark. The flexor digitorum longus tendon enters the foot, having crossed superficially in a medial-to-lateral direction to both the tibialis posterior and the flexor hallucis longus tendons, which are parallel. The flexor digitorum longus tendon is located medial to the sustentaculum tali. The anterior compartment tendons (the tibialis anterior, the extensor hallucis longus, and the extensor digitorum longus) are displayed medially and laterally on the anterior surface of the distal tibia. The anterior tibiotalar fibers of the deltoid ligament are also seen in the plane of the anterior tibia.
Axial Images (Fig. 5.27)
In the axial plane, the low-signal-intensity bands of the anterior and posterior inferior tibiofibular ligaments are demonstrated at the level of the tibial plafond. The inferior extensor retinaculum is identified anterior to and at its attachment to the medial malleolus, and represents the upper limb of this Y-shaped band of deep fascia. At the level of the tibiotalar joint, the tendons of the tibialis anterior, extensor hallucis longus, extensor digitorum longus, and peroneus tertius muscles occupy the anterior compartments in a medial-to-lateral direction. The peroneus brevis muscle and tendon and the more lateral peroneus longus tendon are located posterior to the lateral malleolus. The tendons of the tibialis posterior, flexor digitorum longus, and flexor hallucis longus can be identified posteriorly, running from a medial position posterior to the medial malleolus to a lateral position posterior to the tibial plafond and talar dome. Posterior and medial to the greater saphenous vein, the anterior tibionavicular fibers of the deltoid ligament blend with the low-signal cortex of the anterior surface of the medial malleolus.
The Achilles tendon is identified in cross-section as a thick structure of low signal intensity with a convex posterior sur-face and a flattened anterior surface. The posterior Achilles tendon is formed by the convergence of the gastrocnemius, plantaris, and soleus muscles. The soleus muscle group that is seen at the level of the distal tibia is not visible at the tibiotalar joint level. At the level of the distal lateral malleolus, both the anterior and posterior talofibular ligaments are demonstrated. Medially, the tibionavicular and tibiocalcaneal parts of the deltoid ligament are also shown at this level. The peroneal retinaculum can be seen coursing medial and posterior to the lateral malleolus. The interosseous talocalcaneal ligament is posterolateral
to either the anterior talus or the talar head. The plantar calcaneonavicular ligament, or spring ligament, is located inferior to the lateral malleolus between the lateral talus and tibialis posterior tendon.
to either the anterior talus or the talar head. The plantar calcaneonavicular ligament, or spring ligament, is located inferior to the lateral malleolus between the lateral talus and tibialis posterior tendon.
The calcaneofibular ligament is optimally seen with the foot in 40° of plantarflexion, and on neutral axial images it can be seen lateral to the posterior inferior talus and anterior and medial to the peroneus brevis tendon. The sural nerve, intermediate in signal intensity, is located posteromedial to the peroneus brevis muscle. The tibial nerve is medial to the flexor hallucis longus tendon and continues distally as the medial and lateral plantar nerves. The flexor retinaculum is superficial to the tendons of the deep muscles on the medial side of the ankle. In the foot, the tendons of the flexor hallucis brevis and longus muscles are seen posterior to the first metatarsal and cuneiform. The longitudinally oriented quadratus plantae and abductor hallucis muscles are medial to the calcaneus and cuboid. The peroneus longus tendon, a fourth-layer muscle of the sole of the foot, enters the foot by passing posterior to the lateral malleolus and can be seen obliquely crossing the foot to its insertion onto the base of the first metatarsal and medial cuneiform bone.
The anterior neurovascular bundle, composed of the anterior tibial artery and vein and the deep peroneal nerve, is located posterior to the extensor tendons, whereas the posterior neurovascular bundle, composed of the posterior tibial artery, vein, and tibial nerve, is located posterior to the flexor digitorum and flexor hallucis longus tendons.
Imaging Checklist for the Ankle
A simple way to organize a checklist for the ankle is to analyze the ankle by its constituents: bones and joints, ligaments, and tendons. To confirm and characterize pathology, most of the anatomic structures in the ankle are examined in all three planes.
Key articulations for examination include:
Tibiotalar joint
Posterior subtalar joint
Talocalcaneonavicular joint
All bones are examined for fractures, avascular necrosis (AVN), and edema. Fractures that may be occult on plain films but are well demonstrated on MR examination include:
Fractures occurring at the anterior process of the calcaneus
Fractures of the lateral process of the talus
Navicular fractures
Fractures of the metatarsals
Cuboid fractures (particularly stress fractures)
The ligaments are well displayed and are divided into the:
High tibiofibular ligaments (the anterior and posterior syndesmosis and the interosseous ligament)
Lateral ligaments (the anterior talofibular, calcaneofibular, and posterior talofibular ligaments)
Medial ligaments (the components of the deltoid ligament)
Sinus tarsi ligaments (the extensor roots, interosseous ligament, and cervical ligament)
The tendons are also evaluated. They can be divided into four groups:
The lateral tendons (the peroneus longus and brevis tendons)
The medial tendons (the posterior tibialis, the flexor digitorum longus, and the flexor hallucis longus tendons)
The anterior tendons (the tibialis anterior, the extensor hallucis longus, and the extensor digitorum longus tendons)
The posterior tendons (the Achilles and plantaris tendons)
In conjunction with evaluation of the tendons, the peroneal retinacula covering the peroneal tendons and the flexor retinaculum covering the medial tendons are also examined for tears, commonly diagnosed when the tendons are dislocated. Inferiorly, the plantar fascia should also be inspected.
Sagittal Plane Checklist
In the sagittal plane, all of the major articulations and bones of the ankle are demonstrated. In addition, certain ligamentous structures, including the anterior talofibular (ATFL), posterior talofibular, and transverse tibiofibular ligaments, are examined in the sagittal plane. The long axis of the Achilles tendon is well visualized and the medial, lateral, and anterior tendons are displayed in their long axes, although due to slice gaps, parts of the tendons may not be fully imaged. Triangulating on sagittal images of these tendons is useful for confirmation or further characterization of tendon abnormalities suspected in the axial or coronal plane.
(1) Bones and Articulations
Tibiotalar Joint (Figs. 5.28 and 5.29)
The cartilage surfaces covering the articular surfaces of the talar dome and tibial plafond are visualized on both sagittal and coronal images. Osteochondral lesions, classically described in the talar dome, also affect the tibial plafond. When an osteochondral lesion is identified, the condition of the overlying cartilage (e.g., full-thickness cartilage defect, deep fissuring), the state of the subjacent subchondral bone (including any subchondral cysts or edema), and the presence of any unstable chondral flaps or osteochondral fragments with fluid undermining are key elements in determining the stage and severity of the lesion. The joint is also examined for the presence of effusions, synovitis, and loose bodies.
Impingement of the bony and capsular structures making up the tibiotalar joint can occur anywhere in the joint, and various impingement syndromes have been named according to where in the tibiotalar joint they occur. For example, anterior impingement occurs when large spurs of the anterior tibia and talus are present with anterior synovitis. Anterolateral impingement results from chronic anterior talofibular and calcaneofibular ligament injury (post-inversion injury) and is suggested when there is a synovial mass in the lateral gutter deep to a scarred or chronically torn ATFL and anterolateral capsule. Posterior impingement occurs when the posterior ligaments are impinged between the talus and tibia, and the related os trigonum syndrome occurs when the os trigonum is compressed during plantarflexion, with resulting edema within the os trigonum and across the synchondrosis between the os and the posterior talus.
Subtalar Joints (Fig. 5.30)
The two subtalar joints involve three subtalar facets on the superior surface of the calcaneus, all three of which are optimally visualized on sagittal images. The posterior subtalar facet is located at the posterior subtalar articulation between the talus and calcaneus. The middle and anterior facets make up part of the anterior subtalar joint (or talocalcaneonavicular joint), which is composed of the articulation of three bones: the talus, calcaneus, and navicular. These two joints are susceptible to the usual disorders associated with articular disease, including arthrosis, effusions, ganglion/synovial cysts, and even pigmented villonodular synovitis and other synovial inflammatory processes. The subtalar joints are examined on sagittal images for the presence of subchondral edema on one or both sides of the joint, subcortical cystic change, and cortical irregularity and bony hypertrophic change at the joint. Isolated degenerative joint disease (DJD) is a common underdiagnosed cause of foot pain. Also, subtalar joint DJD is not uncommonly associated with tarsal coalitions as a result of abnormal stresses across the joints. Careful evaluation of the ankle is necessary to identify coalitions in other joints when subtalar DJD is visualized. Coalitions may also involve the subtalar joints themselves.
Other Tarsal Joints (Fig. 5.31)
The calcaneocuboid joint, the talonavicular portion of the talocalcaneonavicular joint, and the cuneonavicular joints are also well visualized on sagittal images and should be examined for pathology. The joints are analyzed for the presence of subchondral edema and cystic change and hypertrophic spurring. The joints should also be inspected for fibrous or osseous coalitions, most commonly calcaneonavicular and talocalcaneal (less commonly talonavicular), and for associated arthrosis.
Hindfoot Bones (Fig. 5.32)
The lateral-most sagittal image displays the lateral malleolus and lateral process of the talus, which should be examined for evidence of fracture or other posttraumatic changes. The talar dome is seen more centrally and should be inspected for evidence of osteonecrosis, osteochondral lesions, or subchondral fractures. The head, neck, and body of the talus are also reviewed for fractures and the tibial plafond for fractures, AVN, or osteochondral lesions. The anterior process of the calcaneus, a site of frequent fractures that are occult on plain films, is assessed for fractures, as is the medial malleolus. The superior and posterior aspects of the calcaneus are reviewed for fractures, AVN, or infection. In children and adolescents, patchy marrow edema is frequently found extending throughout hindfoot and forefoot. There is still debate as to whether this finding reflects a painful syndrome of chronic stress-related edema and bony remodeling or is simply an asymptomatic incidental finding. Less common causes of marrow edema include transient osteoporosis and reflex sympathetic dystrophy (which often is accompanied by soft-tissue edema).
Midfoot/Forefoot Bones (Fig. 5.33)
The navicular, cuneiforms, cuboid, and metatarsal bones are all displayed on sagittal images and should be examined for the presence of stress fractures/acute fractures, AVN, infection, or other bony abnormalities. When significant tarsal-metatarsal DJD is identified, it is imperative to examine Lisfranc’s ligament, extending from the medial cuneiform to the base of the second metatarsal, to ensure the ligament demonstrates normal dark intact fibers. Tears of Lisfranc’s ligament are debilitating injuries that often result in severe midfoot degenerative changes due to the associated tarsal-metatarsal instability caused by such injuries. Lisfranc’s ligament is best evaluated in the axial and coronal planes.
(2) Ligaments
Lateral Ligaments (Fig. 5.34)
The origin and course of the lateral ligaments can be evaluated on sagittal images:
The ATFL is found on sagittal images one slice central to the lateral-most sagittal slice that includes the lateral malleolus. The origin of the ATFL is seen at the anterior inferior tip of the lateral malleolus. The anteromedial course of the ATFL can be followed on the next two images moving centrally, to where it inserts on the talus.
The posterior talofibular ligament (PTFL) is located in a similar fashion. The origin of the PTFL is at the inferior tip of the lateral malleolus, and the tendon can be followed medially to its insertion on the mid-posterior aspect of the talus. The PTFL is seen in cross-section on sagittal images and has a cord-like appearance. Posterior to the talus, this cord-like appearance should not be mistaken for a loose body in the posterior joint.
The calcaneofibular ligament (CFL) also originates from the inferior tip of the lateral malleolus, and courses inferomedially to its attachment on a tubercle on the lateral calcaneus. The course of the CFL is not always well visualized on sagittal images, however, because it may be obscured by overlying peroneal tendons.
The ATFL may be sprained or torn in isolation or together with the CFL, whereas the PTFL is uncommonly acutely injured.
Medial Ligaments (Fig. 5.35)
Although the deltoid ligament components are seen on sagittal images, abnormalities are probably better characterized on coronal and axial images. The deltoid ligament can be found on the medial-most slice of the medial malleolus, in which the medial malleolus appears as two contiguous inferiorly pointing bumps (known as the anterior and posterior colliculi). The origins of the superficial and deep fibers of the deltoid ligament complex are identified at the inferior margin of the medial malleolus, and the fibers can be followed as they course over the next one or two images centrally to their attachment sites.
Sinus Tarsi Ligaments (Fig. 5.36)
The sinus tarsi and canal are fat-filled structures located in the lateral aspect of the ankle between the anterior and posterior subtalar joints. The lateral, intermediate, and medial roots of the inferior extensor retinaculum are the first images seen on sagittal images in the lateral-most aspect of the sinus tarsi. The individual roots may be difficult to distinguish on MR images. On the next image medially, the cervical ligament can be seen originating from the neck of the talus and extending obliquely posteriorly to insert on the calcaneus. Medial to this is the interosseous ligament. Acute strain and high signal associated with these ligaments are seen after injuries. Ganglion cysts arising from these ligaments can also extend into the sinus tarsi fat and occasionally produce symptoms. Joint fluid from within the subtalar joints can also extend into the sinus tarsi, usually without symptoms. As opposed to acute ligamentous injury, the sinus tarsi syndrome represents a more chronic and indolent condition in which there is obliteration of the sinus tarsi fat and chronic scarring (manifested as dark signal on MR images) or synovitis (manifested as intermediate to bright signal on MR images) within the sinus tarsi. Sinus tarsi syndrome often occurs subsequent to ankle sprains or posterior tibialis tendon (PTT) injury. When sinus tarsi syndrome is diagnosed, the lateral ligaments and PTT should be closely examined for associated abnormalities.
(3) Tendons
Achilles Tendon (Fig. 5.37)
The full course of the Achilles tendon is imaged in the sagittal plane. The Achilles tendon should be homogeneously dark in signal and smooth, with a concave or flat anterior border. Midsubstance tendinosis (manifested by fusiform thickening) and insertional tendinitis (seen as distal thickening at the os calcis insertion) can be characterized, as can the location of longitudinal, partial, or complete tears, which are optimally measured in the sagittal plane. The distance from a tear to the os calcis insertion is measured, the location of the tear (at the myotendinous junction, midtendon, or distal tendon insertion) is specified, and the gap between tendon ends is measured. Tear morphology (transverse, oblique, interstitial, involving the medial and/or lateral aspect of the tendon, partial or full thickness) is assessed and specified. In addition, if there is a distal stump of reasonably high-quality tendon left at the distal os calcis insertion, this is measured and noted, as it may be possible to sew the torn tendon directly into an intact distal stump. The presence of associated abnormalities, such as retrocalcaneal bursitis and inflammation of the soft tissues surrounding the tendon (paratendinitis), should also be described.
Plantar Aponeurosis (Fig. 5.38)
The plantar aponeurosis comprises three segments:
A medial segment inferior to the abductor hallucis muscle
A central segment, which originates from the medial process of the calcaneal tuberosity
A lateral segment, which originates along the lateral aspect of the calcaneal tuberosity
Pathology of the true medial segment is rare, whereas pathology of the true central segment is common. Therefore, the central segment is sometimes referred to as the medial segment or medial cord. The proximal portions of the central (or medial) and lateral segments are optimally visualized on sagittal images. When viewing the ankle on sequential sagittal images from medial to lateral, the central (or medial) cord is visualized first, with the lateral cord visualized subsequently, often with no gap seen between the two segments. The normal medial cord is usually somewhat thicker than the lateral cord. Most plantar aponeurosis pathology involves the medial cord first. Any doubt as to which segment is abnormal can be resolved by correlating findings with coronal images. Plantar fasciitis is suggested when there is thickening and increased signal in the proximal plantar aponeurosis. Associated findings such as inferior calcaneal spurs at the plantar aponeurosis origin, calcaneal tuberosity edema, and inflammation in the surrounding fat support the diagnosis. Also, the plantar aponeurosis may be involved by fibromatosis, which typically occurs near the midportion of the aponeurosis, as opposed to plantar fasciitis, which occurs at the calcaneal insertion.
Other Tendons (Fig. 5.39)
The medial, lateral, and extensor tendons are not always optimally viewed in their entirety in the sagittal plane, as sagittal slices can gap over portions of the tendon. However, abnormalities of these tendons seen in the axial and coronal plane are confirmed or further characterized by triangulating on images of the tendon in the long axis on sagittal images. Sagittal images demonstrate the os peroneum, a normal-variant small ossicle within the peroneus longus tendon adjacent to the cuboid. Degeneration and tearing of the peroneus longus tendon around a fractured or otherwise inflamed os peroneum is known as the “painful os peroneum syndrome” and is diagnosed when high signal and tearing of the peroneus longus tendon are visualized about an edematous os peroneum.
Coronal Plane Checklist
The coronal plane is optimal for characterization of osteochondral lesions of the talar dome and tibial plafond. In addition, the deltoid ligament is best viewed in the coronal plane, and the superficial and deep components are readily distinguished. The ATFL, CFL, and PTFL are also visualized. The central and lateral segments of the plantar aponeurosis can be followed back to their individual posterior origins on the inferior calcaneus. The distal courses of the medial and lateral tendons are imaged in cross-section on coronal images. Osseous abnormalities can be further characterized in the coronal plane.
(1) Bones and Articulations
Tibiotalar Joint (Fig. 5.40)
The cartilage surfaces and subchondral regions are displayed on sequential anterior-to-posterior images through the tibiotalar joint and can be examined for abnormalities. Osteochondral lesions of the talar dome and tibial plafond can be localized and staged, and fractures of the medial and lateral malleolus, the tibial plafond, and the talus can be characterized.
Subtalar Joints and Other Articulations and Osseous Structures (Fig. 5.41)
Osseous structures and articulations in the hindfoot and forefoot, including the subtalar joints, the lateral process of the talus, the sustentaculum tali, the navicular, the cuneiforms, and the metatarsals, are also examined in the coronal plane for osseous abnormalities, fractures, arthrosis, or coalitions.
(2) Ligaments
Lateral Ligaments (Fig. 5.42)
In the coronal plane the lateral ligaments are found on the image that cuts through the middle of the lateral malleolus and displays the distal tip most prominently. On this image the PTFL is seen coursing medially from the distal tip of the lateral malleolus to insert on the mid-posterior tibia. The origin of the CFL may also be seen on
this image (or possibly one image posterior). On the next one or two posterior images the course of the CFL can be followed from the distal lateral malleolus posteroinferiorly to its insertion on the lateral calcaneus. On the three or four images anterior to the slice through the middle of the lateral malleolus, the full course of the ATFL is seen as a dark bundle of fibers moving directly anteriorly to insert on the lateral aspect of the talus. The lateral ligaments are examined in the coronal plane, and any findings are correlated with those seen in other planes.
this image (or possibly one image posterior). On the next one or two posterior images the course of the CFL can be followed from the distal lateral malleolus posteroinferiorly to its insertion on the lateral calcaneus. On the three or four images anterior to the slice through the middle of the lateral malleolus, the full course of the ATFL is seen as a dark bundle of fibers moving directly anteriorly to insert on the lateral aspect of the talus. The lateral ligaments are examined in the coronal plane, and any findings are correlated with those seen in other planes.
High Ankle Ligaments (Fig. 5.43)
The anterior syndesmotic ligament (also known as the anterior inferior tibiofibular ligament [AITF]) and the posterior syndesmotic ligament (also known as the posterior inferior tibiofibular ligament [PITF]) are located superior to the ATFL and PTFL on the lateral aspect of the ankle. On coronal images they are visualized coursing obliquely upward from the lateral malleolus to the anterolateral and posterolateral tibia, respectively.
Although the anterior and posterior syndesmotic ligaments are most easily identified on axial images, the coronal and sagittal planes are useful for confirming and further characterizing tears and sprains. These ligaments are important stabilizers of the ankle and are not infrequent causes of persistent pain following inversion injuries. The anterior syndesmotic ligament is more frequently injured than the posterior syndesmotic ligament, and tears are often treated surgically. Undiagnosed tears can lead to significant degenerative arthritis.
Medial Ligaments (Fig. 5.44)
The coronal plane demonstrates both the deep and superficial fibers of the deltoid ligament complex. The deep fibers (posterior tibiotalar and anterior tibiotalar ligaments) form a thick triangular band of fibers that originate from the undersurface of the medial malleolus and attach along the medial aspect of the talus. There is normal high-signal fat interposed between individual fibers of the deep portion of the deltoid ligament, which should not be mistaken for a sprain. In general, the superficial fibers (the superficial posterior tibiotalar, tibiocalcaneal, tibiospring, and tibionavicular ligaments) are thinner than the deep fibers, run parallel with and medial to the deep fibers, and insert more distally (on the navicular, the talus, the spring ligament, and the calcaneus). The deltoid ligament can be acutely sprained or torn or may be scarred. Medial ligament injury commonly occurs in the presence of lateral-sided injury, and in the presence of deltoid ligament injury the lateral ligaments and osseous structures should be examined for concurrent injuries.
(3) Tendons and Muscles
Plantar Aponeurosis (Fig. 5.45)
The central (or medial) and lateral cords of the plantar aponeurosis are easily distinguished in the coronal plane, and abnormalities seen on sagittal plane images are confirmed in the coronal plane.
Inferior Flexor Muscles (Fig. 5.46)
The three most superficial muscles along the plantar aspect of the foot, from medial to lateral, are as follows:
Abductor hallucis
Flexor digitorum brevis
Abductor digiti minimi
These muscles, visualized in cross-section on coronal images, are evaluated for strain or tears and also for the presence of denervation. Chronic denervation is suspected when there is selective fatty atrophy of one or more of the muscles manifested as fatty T1 bright signal interdigitating throughout the muscle. Acute denervation is suggested when there is mild hazy ill-defined high signal on fluid-sensitive sequences throughout the muscle. Denervation suggests injury to one of the branches of the tibial nerve.
Other Tendons (Fig. 5.47)
The lateral, medial, Achilles, and extensor tendons are visualized in the coronal plane, and abnormalities seen on axial plane images are confirmed and further characterized in the coronal plane.
Axial Plane
The tendons and ligaments are visualized in cross-section on axial images, and the axial plane is optimal for evaluating pathology. Bones and articulations are also further analyzed in the axial plane.
(1) Osseous Structures
Hindfoot (Fig. 5.48)
The axial plane affords an additional opportunity to triangulate on and characterize abnormalities of the fibula, tibia, medial and lateral malleolus, talus, and calcaneus.
Midfoot/Forefoot (Fig. 5.49)
The navicular, cuboid, cuneiforms, and metatarsals are also evaluated on axial images. MR of the foot is commonly used to detect stress fractures of the metatarsals. Fractures at the base of the fifth metatarsal, which may be occult on plain films, are readily detected on MR images. Characterization of an accessory navicular as type 1, 2, or 3 is optimally performed on axial images. In addition, stress-related changes across a type 2 navicular synchondrosis, marrow edema in a type 3 cornuate navicular, and tendinosis or tears of the posterior tibialis tendon associated with an accessory navicular are evaluated in the axial plane. Lisfranc’s ligament is often included in the FOV for standard ankle MR examination. Degenerative
arthrosis at the tarsal-metatarsal joints in particular should prompt a careful search for Lisfranc fracture—dislocations in the axial plane.
arthrosis at the tarsal-metatarsal joints in particular should prompt a careful search for Lisfranc fracture—dislocations in the axial plane.
(2) Tendons
Medial Tendons (Fig. 5.50)
Axial images are optimal for evaluating the three medial tendons in cross-section. The medial tendons are:
Posterior tibialis tendon (PTT), the most medial of the three tendons
Flexor digitorum longus (FDL), located just posterolateral to and in close apposition with PTT
Flexor hallucis longus (FHL), the most lateral and posterior of the three tendons
The tibial artery, veins, and nerve run between the FDL and the FHL. The course of the tendons can be followed on sequential images from superior to inferior as they run posteromedial to the tibia and medial malleolus. Inferior to the medial malleolus, the tendons turn and head anteroinferiorly beneath the medial hindfoot to run along the plantar aspect of the foot.
Axial images are particularly helpful in excluding mass lesions (including varices, ganglion cysts, or nerve sheath tumors) within the tarsal tunnel, which may compress the tibial nerve and its branches. Both axial and coronal images are useful for demonstrating denervation of the inferior flexor muscles (supplied by the tibial nerve and its branches). The medial tendons are covered by a flexor retinaculum, which is identified on axial MR images extending posteroinferiorly from the medial malleolus to insert on the calcaneus. The flexor retinaculum may tear from the medial malleolus, allowing subluxation of the medial tendons anterior and medial to the medial malleolus.
Lateral Tendons (Fig. 5.51)
The lateral tendons, the peroneus longus and brevis, are also optimally visualized in the axial plane. When sequential images through the ankle are viewed from superior to inferior, superior images demonstrate the peroneus longus and brevis located posterior to the distal fibula and the peroneus longus located lateral to the peroneus brevis. At this level, the brevis appears flatter and thinner than the longus. At and inferior to the lateral malleolus, the peroneus brevis swings anterior to the longus as the tendons course lateral to the calcaneus. Just inferior to the lateral malleolus, the CFL is visualized coursing anterior and then medial to the peroneus brevis tendon before attaching to the calcaneus. As part of the evaluation of the peroneal tendons, the superior peroneal retinaculum (SPR) at the level of the lateral malleolus is inspected. The SPR originates from the lateral posterior aspect of the lateral malleolus and wraps around the posterolateral aspect of the peroneal tendons before inserting on the calcaneus.
The peroneal retinaculum prevents the peroneal tendons from subluxing laterally over the lateral malleolus. Tears or stripping of the peroneal retinaculum from the lateral malleolus is inferred when subluxation or dislocation of the peroneal tendons is seen lateral to the lateral malleolus.
The peroneal retinaculum prevents the peroneal tendons from subluxing laterally over the lateral malleolus. Tears or stripping of the peroneal retinaculum from the lateral malleolus is inferred when subluxation or dislocation of the peroneal tendons is seen lateral to the lateral malleolus.
As the peroneus brevis degenerates, it becomes C-shaped and wraps around the anterior aspect of the peroneus longus tendon. The peroneus brevis may also develop longitudinal tears and split into two subtendons. The peroneal tendons may be partially torn or completely ruptured. Partial tears can be subclassified as grade I (a thickened tendon) or grade II (a thinned attenuated tendon, representing more severe changes). Complete rupture, classified as grade III, is characterized by absence of the tendon on axial images located at the gap created by the rupture.
Anterior Tendons (Fig. 5.52)
The anterior tendons are also optimally evaluated in the axial plane. The four anterior tendons that run from medial to lateral are:
Tibialis anterior (the most likely of the extensor tendons to tear)
Extensor hallucis longus
Extensor digitorum longus
Peroneus tertius
Posterior Tendons (Fig. 5.53)
The posterior tendons, the Achilles tendon and plantaris tendon, are also evaluated on axial plane images.
The Achilles tendon is seen in cross-section and appears convex posteriorly and concave or flat anteriorly. Tendinosis is
associated with a convex appearance to the tendon anteriorly, as well as tendon thickening and often increased intrasubstance signal. Tears and tendinosis suspected in the sagittal plane are confirmed and further characterized in the axial plane.
associated with a convex appearance to the tendon anteriorly, as well as tendon thickening and often increased intrasubstance signal. Tears and tendinosis suspected in the sagittal plane are confirmed and further characterized in the axial plane.
The plantaris tendon is commonly identified on axial MR images as a small separate tendon inserting medial to the Achilles tendon on the os calcis. Not uncommonly, when the Achilles tendon completely ruptures, the plantaris tendon remains attached to the os calcis and the plantaris may be mistaken for small intact medial fibers of an otherwise torn Achilles tendon.
(3) Ligaments
Lateral Ligaments (Figs. 5.54 and 5.55)
Both the anterior and posterior syndesmotic ligaments follow an oblique upward course from the anterior and posterior lateral malleolus to the tibia and are seen on sequential axial images from superior to inferior through the distal tibia—fibula articulation. The tibial attachments are first seen on superior images and can be followed to their fibular insertions over the next three or four inferior images. Syndesmotic tears (anterior more commonly than posterior), sprains, and chronic thickening and scarring from old injury can be characterized on MR images.
Several images inferior to the syndesmoses, the ATFL is encountered coursing obliquely anteromedially from the anteroinferior lateral malleolus to the anterolateral talus. The ATFL fibers are taut and dark. The distal aspect of the PTFL is seen at the same level, and the course of the ligament to its posterior talar insertion can be followed on the next two or three images superiorly. The origin of the CFL from the lateral malleolus is located at the distal tip of the lateral malleolus and can be followed inferiorly for two or three images as it passes medial to the peroneal tendons and inserts on the calcaneus. A torn ATFL may appear as complete obliteration of fibers or as a fluid-filled discontinuity across the ruptured ligament with retraction of wavy and lax fibers. Subacute tears demonstrate amorphous intermediate-signal scarring, with less surrounding inflammation and fluid. Chronic tears may manifest as severe attenuation of fibers or as chronically thickened and scarred fibers. Also, following a short to moderate interval after ATFL sprain/tearing, lateral impingement of the lateral gutter synovium may occur due to the resulting lateral ankle instability. Lateral impingement is manifested on axial MR images as severe synovitis, often with a synovial impingement mass within the lateral gutter deep to the ATFL and anterolateral capsule. Surgical débridement of the impingement mass and other synovitis and scarring in the lateral gutter may alleviate symptoms associated with lateral gutter syndrome.
Medial Ligaments (Fig. 5.56)
The medial ligaments make up the deltoid ligament complex. On axial images the deltoid ligament is located by a superior-to-inferior review of images starting with the medial malleolus. The first image inferior to the distal tip of the medial malleolus displays the superior aspect of the deltoid ligament. The deltoid is composed of a superficial and a deep component. The deep component sweeps inferolaterally from the tip of the medial malleolus and is visualized on axial images as a striated band of fibers coursing laterally from the medial malleolus to insert onto the talus one or two slices inferior to the medial malleolus. The posterior tibialis tendon is visualized coursing directly posteromedial to the deltoid. The superficial deltoid is broader and is seen as a broad, thin band of fibers just superficial to the deep deltoid component. The superficial fibers fan out anterior and posterior to the deep fibers and extend inferiorly to insert on the calcaneus, the navicular, and the more inferior anterior and posterior talus. The spring ligament is seen more inferiorly extending from the sustentaculum talus to the posteromedial process of the navicular. Similar to other ligaments, the deltoid may be strained or torn, or thickened and scarred.
Sample MRI Report
Clinical Information: Old ankle sprain, inversion injury, continued pain
Technique: Sagittal proton density with fat saturation, sagittal T1, coronal proton density with fat saturation, coronal T1, axial proton density with fat saturation, axial proton density without fat saturation
Findings: There is an osteochondral lesion in the medial aspect of the talus (Fig. 5.57A, B). There is a 3 mm (medial to
lateral) × 8 mm (anterior to posterior) fragment of bone within the osteochondral bed (Fig. 5.57C, D). There is bone marrow edema associated with the osteochondral lesion (Fig. 5.57E). The bone marrow edema is in a 1-cm area. There is interruption of the subchondral plate in the area of the osteochondral lesion (Fig. 5.57F).
lateral) × 8 mm (anterior to posterior) fragment of bone within the osteochondral bed (Fig. 5.57C, D). There is bone marrow edema associated with the osteochondral lesion (Fig. 5.57E). The bone marrow edema is in a 1-cm area. There is interruption of the subchondral plate in the area of the osteochondral lesion (Fig. 5.57F).
Degenerative change is seen in the anterior aspect of the tibiotalar joint with anterior spurring (Fig. 5.57G) and mild bone marrow edema in the anterior distal talus (Fig. 5.57H). This is consistent with anterior osseous impingement of the ankle.
The subtalar joints are intact. The Achilles tendon is intact. The plantar fascia is intact. The anterior talofibular ligament is mildly scarred but intact (Fig. 5.57I). The posterior talofibular ligament, the anterior and posterior syndesmotic ligaments, and the deltoid ligament are intact. The posterior tibialis, flexor digitorum and flexor hallucis longus, the peroneal tendons, and the extensor tendons are intact.
Coronal images confirm the osteochondral lesion in the talar dome. The area of involvement is 5 mm × 12 mm. The actual fragment within the osteochondral bed is appreciated with an anterior-to-posterior dimension of approximately 8 mm.
Impression:
Medial osteochondral talar dome lesion. There is a fragment of osseous tissue within the osteochondral bed that may be attached by synovium. The fragment measures 8 mm anterior to posterior and 3 mm medial to lateral. This correlates with a stage III osteochondral lesion. There is associated bone marrow edema of 10 mm and subchondral sclerosis. There is irregularity of the overlying subchondral plate. There are also mild cystic changes in the adjacent portion of the talus, although no fluid is directly undermining the osteochondral lesion itself.
Anterior osseous impingement of the ankle with spurring of the anterior aspect of the tibiotalar joint and bone marrow edema demonstrated in the anterior distal tibia
Chronic thickening of the anterior talofibular ligament without disruption
Anatomy of the Ankle and Foot
Pearls and Pitfalls
Anatomy of Ankle and Foot
The syndesmotic ligaments consist of the anterior syndesmotic or anterior inferior tibiofibular ligament and the posterior syndesmotic or posterior inferior tibiofibular ligament, the interosseous membrane, and the transverse tibiofibular ligament.
The transverse tibiofibular ligament represents the posterior labrum of the ankle and projects inferior to the posterior tibial margin.
The deltoid ligament consists of superficial and deep layers.
The ATFL is taut in plantarflexion.
The tibial slip is the posterior intermalleolar ligament.
The tendons of the deep calf and the neurovascular structures of the posterior compartment pass deep to the flexor retinaculum.
Compartments of the Leg
Anterior and posterior intermuscular septa and the interosseous membrane define the major compartments of the lower leg (Fig. 5.58):
The anterior compartment of the leg consists of the tibialis anterior, the extensor hallucis longus, the extensor digitorum longus, and the peroneus tertius muscles. The neurovascular bundle contains the deep peroneal nerve and the anterior tibial artery.
The posterior compartment is divided into superficial and deep sections by deep transverse fascia. The superficial posterior compartment consists of the gastrocnemius, the plantaris, and the soleus muscles. The deep posterior compartment contains the popliteus, the FDL, the FHL, and the tibialis posterior muscles. The neurovascular supply is provided by the tibial nerve and posterior tibial artery.
The anterolateral compartment contains the peroneus longus and peroneus brevis muscles. The neurovascular supply is from the superficial peroneal nerve and branches of the peroneal artery.
Distal Tibiofibular Joint
The distal or inferior tibiofibular syndesmosis is a fibrous joint strongly connected by the interosseous ligament, which is continuous with the crural interosseous membrane. The tibiofibular joint is defined by the bony anatomy of the convex medial aspect of the distal fibula and the corresponding concavity on the lateral aspect of the distal tibia (Fig. 5.59).13,14 The strong anterior and posterior inferior tibiofibular ligaments reinforce the joint anterior and posterior to the interosseous ligament. The transverse tibiofibular ligament represents the distal deep fibers of the posterior inferior tibiofibular ligament. Using ankle arthroscopy, we have identified four common variations of the arrangement of the posterior inferior tibiofibular ligament relative to the transverse tibiofibular ligament. The AITF extends obliquely from the anterior border of the lateral malleolus upward and medially to the anterolateral tibial tubercle more superiorly.13,14 The flat band of fibers may be divided into two or three bands, or may present as a multifascicular structure. The PITF is smaller than the AITF and is quadrilateral in shape.13,14 Fibers originate from the posterior border of the lateral malleolus and extend superomedially onto the posterolateral tibial tubercle. The transverse tibiofibular ligament (the deep component of the posterior inferior tibiofibular ligament) is a strong thick band that extends from the posterior fibular tubercle and upper digital fossa and inserts on the posterior aspect of the tibial articular surface, reaching to the medial malleolus. The transverse tibiofibular ligament forms a posterior labrum by projecting inferiorly to the posterior tibial margin, thus deepening the tibial articular surface of the tibiotalar joint. The transverse ligament is in contact with the posterolateral talar articular cartilage surface. Posterior impingement may be caused by hypertrophy of the transverse ligament and its synovial covering.
Ankle Joint
The ankle or tibiotalar joint is a synovial articulation formed by the distal tibia and fibula. Although it is often described as a hinge joint between the talus and the mortise (Fig. 5.60), the apex of rotation of the ankle joint is not fixed, as it would be in a simple hinge joint. In fact, the apex of rotation changes during extremes of plantarflexion and dorsiflexion. The articular surfaces of the tibiotalar joint are covered with hyaline cartilage (Fig. 5.61), and the fibrous capsule attaches to the articular margins of the tibia, fibula, and talus, with an anterior extension onto the talar neck. The capsule, which is thin anteriorly and posteriorly, is reinforced by strong collateral ligaments. The socket, framed by the distal tibia and medial and lateral malleoli, is wider anteriorly than posteriorly and is completed posteriorly by the transverse tibiofibular ligament (Fig. 5.62). The synovial membrane is attached to all articular margins and covers the intracapsular part of the talar neck.
Ankle Joint Ligaments
Deltoid Ligament
The medial or deltoid ligament is a strong band attached by its apex to the border of the medial malleolus and consists of superficial and deep fibers (Fig. 5.63). The triangular, superficial part of the deltoid is formed by the tibionavicular fibers anteriorly, the tibiocalcaneal fibers medially (the strongest component of the superficial deltoid), and the superficial posterior tibiotalar fibers posteriorly. Behind the navicular tuberosity, tibionavicular fibers blend with the medial margin of the plantar calcaneonavicular or spring ligament. A tibioligamentous
fascicle inserts onto the superior border of the calcaneona vicular ligament. The deep part of the deltoid, which is rectangular, consists of a small anterior component (the anterior tibiotalar ligament) and a strong posterior component (the posterior tibiotalar ligament) (Fig. 5.64). The posterior tibiotalar ligament represents the strongest part of the entire medial ligament complex. The deep portion of the deltoid ligament, covered by synovium, is intra-articular.
fascicle inserts onto the superior border of the calcaneona vicular ligament. The deep part of the deltoid, which is rectangular, consists of a small anterior component (the anterior tibiotalar ligament) and a strong posterior component (the posterior tibiotalar ligament) (Fig. 5.64). The posterior tibiotalar ligament represents the strongest part of the entire medial ligament complex. The deep portion of the deltoid ligament, covered by synovium, is intra-articular.
![]() FIGURE 5.58 ● transverse section through the midcalf shows the anterior and lateral compartments and their contents. |
![]() FIGURE 5.61 ● An anterior view of the ankle joint and its articular surfaces is revealed by removal of the capsule. |
Lateral Ligament
Three distinct bands make up the weaker lateral ligament of the ankle (Fig. 5.65): the ATFL, the CFL, and the PTFL. The posterior inferior tibiofibular ligament lies superior to the horizontally oriented PTFL. The inferior part of the posterior inferior tibiofibular ligament is also referred to as the transverse tibiofibular ligament (Fig. 5.66). In dorsiflexion, the posterior talofibular (Fig. 5.67) and posterior inferior tibiofibular ligaments diverge like the blades of a scissors, and in plantarflexion they lie edge to edge.
![]() FIGURE 5.64 ● A coronal section through the ankle (tibiotalar joint) and talocalcaneal joints shows their articular surfaces. |
The anterior talofibular ligament, which is a flat, relatively strong ligament (although the first to fail in lateral ligament injuries), may be divided into two bands. This ligament is taut in plantarflexion.13,14 The CFL, the largest of the lateral collateral ligaments, is a strong, cord-like structure. It is deep to the peroneal tendons and their sheaths and extends from the distal anterior border of the lateral malleolus inferiorly and posteriorly to insert on the upper part of the lateral surface of the calcaneus. The PTFL, the strongest and deepest portion of the lateral ligament, is intracapsular but extrasynovial. The posterior intermalleolar ligament or tibial slip originates on the PTFL, which inserts on the posterior tibia and posterior surface of the medial malleolus and blends with the transverse tibiofibular ligament.
Tarsal Joints
Subtalar Joint
The subtalar or talocalcaneal joint is the posterior articulation between the talus and the calcaneus. Functionally, the subtalar joint includes the talocalcaneal part of the talocalcaneonavicular joint (Fig. 5.68). The strong t alocalcaneal or interosseous ligament attaches to the sulcus tali and sulcus calcanei. The capsule is strengthened by the medial and lateral talocalcaneal ligaments.
Talocalcaneonavicular Joint
The talocalcaneonavicular joint is a multiaxial articulation at the triple-faceted anterior-inferior talar surface with the talar head, the posterior concavity of the navicular bone, the middle and anterior talar facets of the calcaneus, and the fibrocartilaginous superior surface of the plantar calcaneonavicular (i.e., spring) ligament. The talonavicular, plantar calcaneonavicular, and calcaneonavicular parts of the bifurcated ligament support the osseous components of this joint.
Calcaneocuboid Joint
The calcaneocuboid joint is a separate joint with its own capsule between the anterior calcaneus and the posterior surface of the cuboid. The calcaneocuboid and the talonavicular part of the talocalcaneonavicular joint form the midtarsal joint (Fig. 5.69). Support is provided by the fibrous capsule, the calcaneocuboid part of the bifurcate ligament, and the long plantar and plantar calcaneocuboid (i.e., short plantar) ligaments. The bifurcate ligament is a strong, Y-shaped ligament on the dorsal surface of the joint, with attachments to the anterior dorsal calcaneal surface proximally and to the dorsomedial aspect of the cuboid and dorsolateral aspect of the navicular bone distally. The long plantar ligament, also a strong ligament, is located along the plantar surface. It is attached to the undersurface of the calcaneus, the cuboid, and the bases of the third, fourth, and fifth metatarsal bones. A tunnel for the peroneus longus is created as it bridges the tendon groove on the plantar surface of the cuboid. The plantar calcaneocuboid, or short plantar ligament, is a wide, short band attached to the anterior tubercle on the plantar aspect of the calcaneus and the adjacent surface of the cuboid.
Other Tarsal Joints
The cuneonavicular joint is a synovial joint formed by the navicular bone and the three cuneiform bones (see Fig. 5.69). The cuboideonavicular joint is a fibrous joint. The intercuneiform and cuneocuboid joints are synovial joints continuous with the cuneonavicular joint cavity.
Regional Anatomy of the Ankle
Retinacula
The extensor (Fig. 5.70) and flexor (Fig. 5.71) retinacula are formed by thickened deep fascia and maintain the position of the long tendons crossing the ankle. The superior extensor retinaculum attaches to the distal anterior fibula and tibia and invests the tibialis anterior tendon medially. The Y-shaped inferior extensor retinaculum attaches to the anterolateral part of the calcaneus (the stem) and extends to the medial malleolus (the upper limb) and the medial plantar fascia (the lower limb). The tibialis anterior, the extensor hallucis longus, the extensor digitorum longus, and the peroneus tertius tendons divide the upper limb of the retinaculum into superficial and deep layers. The flexor retinaculum extends inferiorly and posteriorly from the medial malleolus to the medial calcaneal surface. The tendons of the deep calf muscles (the FDL, the FHL, and the tibialis posterior) and the neurovascular structures in the posterior compartment pass underneath the flexor retinaculum before entering the foot.
The SPR extends inferiorly and posteriorly from the lateral malleolus to the lateral calcaneal surface, binding the peroneus longus and brevis tendons. The inferior peroneal retinaculum is attached to the peroneal trochlea and calcaneus above and below the peroneal tendons.
Anterior Structures
The saphenous nerve, the great saphenous vein, and the medial and lateral branches of the superficial peroneal nerve pass anterior to the extensor retinaculum in a medial-to-lateral direction. The tibialis anterior tendon, the extensor hallucis longus tendon, the anterior tibial artery with venae comitantes, the deep peroneal nerve, the extensor digitorum longus tendon, and the peroneus tertius pass deep to or through the extensor retinaculum in a medial-to-lateral direction (Figs. 5.72 and 5.73).
Posterior Structures
The tibialis posterior tendon (PTT), the FDL, the posterior tibial artery with venae comitantes, the tibial nerve, and the FHL flow in a medial-to-lateral direction and are located posterior to the medial malleolus and deep to the flexor retinaculum. The sural nerve and the small saphenous vein pass posterior to the lateral malleolus, superficial to the SPR. The peroneus longus and brevis tendons course posterior to the lateral malleolus deep to the SPR. The pre-Achilles fat pad and the Achilles tendon are located posterior to the ankle. The plantaris tendon is identified medial to the Achilles tendon and joins the anteromedial aspect of the Achilles tendon at the level of the subtalar joint.
Foot
Muscles of the Sole of the Foot
Deep to the plantar aponeurosis (Fig. 5.74), the muscles of the sole of the foot are divided into four layers from superficial to deep:
The first layer consists of the abductor hallucis, the flexor digitorum brevis, and the abductor digiti minimi (Fig. 5.75).
The second layer consists of the quadratus plantae, the lumbricals, the flexor digitorum longus tendons, and the flexor hallucis longus tendons (Fig. 5.76).
The third layer includes the flexor hallucis brevis, the adductor hallucis, and the flexor digiti minimi brevis (Fig. 5.77).
Arches of the Foot
The arches of the foot, which provide support for bipedal motion and forward propulsion, are as follows:
The medial and lateral longitudinal arches are formed by the tarsal and metatarsal bones (Fig. 5.80). The higher medial arch, which forms the instep of the foot, consists of the calcaneus, the talus, the navicular, the three cuneiform bones, and the medial three metatarsals (see Fig. 5.80; Fig. 5.81). The plantar calcaneonavicular (i.e., spring) ligament helps support the head of the talus, which articulates with the navicular anteriorly and the sustentaculum tali posteriorly (Fig. 5.82). The lateral arch consists of the calcaneus, the cuboid, and the lateral two metatarsals.
The transverse arch of the foot consists of the five metatarsal bones and the adjacent cuboid and cuneiform bones.
![]() FIGURE 5.70 ● (A) The lateral aspect of the ankle and foot shows the peroneal tendons and the retinacula. (B) Lateral view of the ankle tendons and tendon sheaths. |
![]() FIGURE 5.72 ● The principal structures of the dorsum of the ankle and foot can be seen after removal of the extensor retinaculum. |
Body weight is transmitted through the anterior and posterior pillars of the arches. The posterior pillars of the medial and lateral arches are the tubercles on the inferior calcaneal surface. The anterior pillars of the medial and lateral arches are formed by their respective metatarsal heads.
Metatarsophalangeal and Interphalangeal Joints of the Foot
The metatarsophalangeal joints are ball-and-socket articulations between the metatarsal head and the base of the proximal phalanx and the fibrocartilaginous plantar plate (Figs. 5.83 and 5.84). The interphalangeal joints are hinge joints that permit flexion and extension (Fig. 5.85).
Anatomic Variants
Pearls and Pitfalls
Anatomic Variants
An accessory soleus may present as a mass in the distal calf or medial ankle.
The flexor digitorum accessorius is an anomalous muscle posterior to the FHL.
The peroneus quartus originates from the peroneus brevis muscle and inserts onto the peroneal tubercle of the calcaneus.
A number of normal anatomic variants of the ankle are seen on MR images and may be misleading.15 These have been characterized in studies of asymptomatic patients and include the following:16
In the posterior tibiotalar joint, a low-signal-intensity cortical irregularity may mimic the appearance of osteonecrosis.
The posterior inferior tibiofibular ligament may be mistaken for a loose body in the posterior capsule on midsagittal images.
Occasionally, the intact PTFL appears as an attenuated structure with signal inhomogeneity.
Less frequently, fluid in the peroneal tendon sheath may be confused with a longitudinal tendon tear.
In one patient, axial planar images revealed marked asymmetry and hypertrophy of the peroneus brevis muscle and tendon as a normal anatomic variant.
An accessory soleus muscle (Fig. 5.86) is an anatomic variant that may present as a mass in the distal calf or medial ankle.17,18
The tensor fasciae suralis represents an anomalous muscle and tendon that contributes to the Achilles tendon and originates from the semitendinosus muscle.19 It may be mistaken for a posterior thigh, popliteal, calf, or Achilles tendon mass or lesion. MR imaging demonstrates imaging characteristics of either muscle or tendon.20
The flexor digitorum accessorius is an anomalous muscle located posterior to the FHL and has been associated with tarsal tunnel syndrome, although it is usually asymptomatic (Fig. 5.87).
In addition, the orientation of a tendon relative to the main magnetic field (B0) affects its MR appearance. When the orientation approximates the “magic angle” of 55°, the collagen may demonstrate intermediate signal intensity with loss of the normal low spin-density characteristics (Fig. 5.89).21 The magic-angle effect is prominent on short-TE spin-echo or GRE images. T2-weighted images minimize the magic-angle signal intensity. On sagittal images, the peroneus longus and FHL tendons are commonly affected and often display intermediate signal intensity in their midportions. Changes in ankle position that alter the orientation relative to the main magnetic field can be used to confirm that the tendon is normal.
Most ankle ligaments, including the anterior talofibular, the calcaneofibular, and the superficial deltoid, show uniform low signal intensity on all pulse sequences. Mixed or striated signal intensity, however, can be seen in the posterior talofibular, posterior inferior tibiofibular, deep deltoid, and interosseous talocalcaneal ligaments.
Sesamoid bones within the peroneus longus (os peroneum) (Fig. 5.90) or the distal tibialis posterior normally demonstrate
hyperintensity on T1-weighted images, secondary to fatty marrow. This finding should not be mistaken for tendon degeneration or a tear. Accessory bones, including the os tibiale externum (an accessory navicular bone medial to the navicular) and the os trigonum (located posterior to the talus and occurring approximately 10% of the time), represent commonly seen secondary ossification centers. These are normal variants that may be misinterpreted as a fracture or loose body.21
hyperintensity on T1-weighted images, secondary to fatty marrow. This finding should not be mistaken for tendon degeneration or a tear. Accessory bones, including the os tibiale externum (an accessory navicular bone medial to the navicular) and the os trigonum (located posterior to the talus and occurring approximately 10% of the time), represent commonly seen secondary ossification centers. These are normal variants that may be misinterpreted as a fracture or loose body.21
![]() FIGURE 5.74 ● The plantar aponeurosis, deep fascia, and a cutaneous nerve are revealed by removing the skin of the sole of the foot. |
![]() FIGURE 5.80 ● The dorsal aspect of the bones of the foot shows the medial and lateral longitudinal arches. |
![]() FIGURE 5.84 ● The internal features of the first metatarsophalangeal joint are revealed when part of the capsule and the distal part of the metatarsal bone are removed. |
Small fluid collections in the ankle joint, including the tibiotalar and subtalar joints as well as the ankle tendon sheath, are common in asymptomatic individuals.22 Relatively larger amounts of fluid, however, are usually associated with the FHL tendon. Fluid is not commonly seen in the extensor tendons. The amount of fluid in normal or asymptomatic and symptomatic patients may be similar in volume and distribution. Cystic change with hyperintensity in the anterolateral third of the calcaneus can be a normal finding (Fig. 5.91). Bone cysts, including intraosseous lipoma, however, may also occur in this area.
Pathology of the Ankle and Foot
Osteochondral Lesions
Pearls and Pitfalls
Osteochondral Lesions
Osteochondral lesion of the talus (OLT) is the accepted terminology and should be used in place of transchondral fracture, osteochondral fracture, or osteochondritis dissecans.
Osteochondral lesions may involve the posteromedial or anterolateral talus.
An equivalent tibial lesion is also referred to as an osteochondral lesion (osteochondral lesion of the tibia).
It is important not to overestimate the size of the lesion by including adjacent marrow edema in the dimensions of the lesion proper.
Osteochondral lesion of the talus (OLT) is the accepted term for a variety of disorders, including transchondral fracture, osteochondral fracture, osteochondritis dissecans, and talar dome fracture. Osteochondral lesions of the medial and lateral talar dome involve the articular cartilage and subchondral bone and have a high association with antecedent trauma (e.g., torsional impaction). They are responsible for 0.09% of all fractures, 1% of talar fractures, 4% of cases of osteochondritis, and 6.5% of sprained ankles. They occur more often in males (67%) than females (33%), usually approximately 25 years of age, although there is a second peak of occurrence in the fifth to sixth decades. They are not commonly seen in children.
Etiology, Pathology, and Clinical Features
Trauma remains the best-accepted theory for the etiology of OLT, which is thought to represent the chronic phase of a compressed or avulsed talar dome fracture.13 Anatomically relevant features include the wider anterior talus, the narrower posterior talus, and the ankle mortise (the medial and lateral malleolus). Direct trauma or repetitive microtrauma may contribute to the development of this lesion in individuals predisposed to talar dome ischemia. Subchondral fractures are susceptible to AVN, and an osteonecrotic process leads to subchondral fracture and collapse. Increased joint pressure forces synovial fluid into the fracture site, preventing healing. Torsional impaction may also be a contributing factor. Lesions are bilateral in 10% to 30% of cases.
The lateral and medial surfaces of the talus are involved in approximately 40% and 60% of osteochondral lesions of the talus, respectively. Lateral lesions (Fig. 5.92), which tend to be wafer-shaped and thin, are almost always (94%) caused by trauma. The most common mechanism is a strong inversion force with dorsiflexion of the foot and internal rotation of the tibia. The lateral talar margin is impacted or compressed against the medial fibula, causing shear end compression. Lateral lesions usually occur in the mid- to anterior aspect of the talar dome.
Medial lesions, which are usually cup-shaped and deeper than lateral lesions (see Fig. 5.92), are not as strongly associated with a history of antecedent trauma. The mechanism of injury is a strong inversion force with plantarflexion of the foot and lateral rotation of the tibia on the talus (external rotation). The posteromedial edge of the talar dome is impacted against the posteromedial tibia with shear stress injury (Fig. 5.93). Lesions are bilateral in 10% to 30% of cases.
If bone alone is affected by the shear stress, the result is a subchondral fracture with intact articular cartilage (Fig. 5.94). If the stress exceeds the ultimate strength of both bone and cartilage, the result is a complete displaced osteochondral lesion.23
Classic pathologic findings include a soft, frayed cartilage, subchondral plate defects or cysts, loose bodies, joint effusion, and a stable or unstable fragment in the crater. Clinically, the patient presents with some or all of the following:
Pain: persistent ankle pain after an inversion injury or chronic ankle pain and sprains. If the collateral ligament fails to rupture because articular surfaces are in direct contact, pain may be minimal.
Stiffness, swelling, and reduced range of motion
Ecchymosis
Catching, clicking, locking, or giving way
Diagnosis and Staging
There are several staging systems for OLT. A surgical grading system, based on the articular cartilage, classifies lesions into six categories:
Grade A: The articular cartilage is smooth, intact, and soft.
Grade B: The articular cartilage has a rough surface.
Grade C: Fibrillations and fissures in the cartilage
Grade D: A flap and exposed bone
Grade E: A loose, undisplaced fragment
Grade F: The fragment is displaced.
Berndt and Harty24 have developed a four-part staging system for characterizing OLT based on plain radiographs:
Stage I: A subchondral compression fracture of the talus with no ligamentous sprain. Radiograph results are negative, and lesions may be painless.
Stage II: A partially detached osteochondral fragment with a hinge or flap of articular cartilage. A T2- or T2*-weighted image may be necessary to identify the osteochondral fragment.
Stage III: A complete nondisplaced fracture remains within the bony crater.
Stage IV: Detachment with a loose osteochondral fragment
Although lesions in stages II to IV are themselves painless, they may be associated with a painful sprain or rupture of the collateral ankle ligaments.
To improve on the correlation between arthroscopic and conventional radiographic findings, another classification system, based on CT findings, was developed by Ferkel and Sgaglione.25 CT is performed in the direct axial and coronal planes at 1.5 mm, in association with sagittal reformations. (CT scans are performed for a known diagnosis of OLT, whereas MR imaging is preferred in patients with ankle pain of unknown etiology.) The CT classification progresses from stage I to stage IV:
Stage I: A cystic lesion of the talar dome with an intact roof
Stage IIA: A cystic lesion with communication to the talar dome surface
Stage IIB: An open articular surface lesion with an overlying nondisplaced fragment
Stage III: A nondisplaced lesion with lucency
Stage IV: A displaced fragment
Both CT and MR imaging provide more information about the size and location of the lesion than does conventional radiography.
MR imaging can also be used to stage OLT, and it has the advantage of detecting radiographically occult lesions that in certain cases may not be evident on CT. In a direct comparison of CT with MR imaging, Anderson et al.26 found that, compared with MR imaging, CT did not detect stage I lesions in 4 of 24 patients. The following proposed four-stage classification of OLT lesions is based on MR findings:
Stage I: Subchondral trabecular compression. Radiograph results are negative, bone scans are posi-tive, and marrow edema is seen on MR imaging (Fig. 5.95).
Stage IIA: A subchondral cyst (Fig. 5.96)
Stage IIB: Incomplete separation of the fragment (Fig. 5.97)
Stage IV: A displaced fragment (Fig. 5.100)
Two classification systems have been developed based on arthroscopic findings in OLT. In the system proposed by Pritsch et al.,27 the appearance of the overlying cartilage is organized into three grades:
Grade 1: The cartilage is intact, firm, and shiny.
Grade 2: The articular cartilage is intact but soft.
Grade 3: The cartilage is frayed.
Another arthroscopic staging system was developed by Cheng et al.28 after reviewing 100 consecutive ankle arthroscopies.
MR Appearance
As mentioned, results of x-rays in the early stages of OLT may be negative, and the lesions may not be detected until a necrotic focus is observed.26 Necrosis is characterized by increased bone density in the necrotic focus and surrounding demineralization secondary to increased vascularization. In addition, it is not possible to assess the integrity of hyaline articular cartilage surfaces in osteocartilaginous defects with conventional radiographs or CT scans. With MR imaging, however, tibiotalar anatomy can be displayed in the coronal, axial, or sagittal planes to identify the talar defect and the presence of an avulsed body fragment. Subchondral marrow and articular cartilage surfaces are uniquely demonstrated on MR images. The normal hyaline articular cartilage surface of the talar dome demonstrates intermediate signal intensity on T1- and T2-weighted images, low to intermediate signal intensity on FS PD FSE images, and high signal intensity on T2*-weighted images.
In OLT, the following MR findings are key:
A detached cortical fragment that remains low in signal intensity
Adherent hyaline articular cartilage, reparative fibrocartilage, and associated fibrous tissue
A low- or intermediate-signal-intensity bony defect of the talus on T1-weighted images, depending on the degree of synovial fluid and fibrous tissue, respectively
Increased-signal-intensity synovial fluid contents on FS PD FSE images
Peripheral areas of low signal intensity within the subchondral bone on T1- and FS PD FSE images, which correlate with reactive bone sclerosis on plain radiographs
Abnormalities of the articular surface, including regions of cartilage thinning, bowing, nodularity, or disruption29
Accumulation of joint fluid at or undermining the cartilage surface, indicating small fissures or breaks
![]() FIGURE 5.87 ● The flexor digitorum accessorius longus muscle is located posterior to the flexor hallucis longus and courses through the tarsal tunnel with the posterior tibial neurovascular bundle. |
On STIR (including FSE STIR) and FS PD FSE sequences, there are large areas of adjacent subchondral talar marrow edema or hyperemia, which are hyperintense compared with the low signal intensity observed on T1-weighted images. These subchondral changes should not be misinterpreted and do not indicate that the overlying osteochondral lesion is more extensive or more aggressive than the focal and smaller superficially located lesion. In contrast to AVN of the talus, the marrow hyperemia associated with the osteochondral lesion is usually less extensive and directly radiates from the lesion. Early AVN of the talus may be seen in association with a more diffuse hyperemia or edema of the entire talar head and body.
On sagittal or coronal images, a focal, upward bowing of the hyaline cartilage overlying the bony defect may be demonstrated. The cartilage may be deformed or bowed without disruption (Fig. 5.101) (frequently showing softening at surgery), or both articular cartilage surface and bone may be involved in a complete lesion (when the shear stress surpasses the strength of both).23 A postsurgical fibrocartilaginous scar can appear as an intermediate-intensity, focal area of thickening, bridging a cartilaginous defect. T2*-weighted images are useful for identification of small areas of cartilage disruption. However, FS PD FSE and STIR images are more sensitive.23
MR imaging identifies both the presence of undermining fluid and fragment displacement. Fluid transgressing articular cartilage and subchondral bone edema can be identified routinely on FS PD FSE and STIR images with greater sensitivity than with corresponding FS PD FSE or T2*-weighted images. De Smet et al.30 have correlated the stability of the osteochondral fragment with signal intensity on T2-weighted images. They found an irregular high-signal-intensity zone at the fragment—talar interface in partially attached fragments, whereas a complete ring of fluid signal intensity surrounded the lesion in unattached fragments. Granulation tissue may also produce a high-signal-intensity band at the fragment—subchondral bone interface, which should not be misinterpreted as articular cartilage violation.
Intravenous contrast administration may help to evaluate the congruity of the articular cartilage surface and to depict subchondral hyperemia and synovial tissue hypertrophy. Intra-articular contrast (MR arthrography) with FS is used to directly evaluate the flow of contrast over the articular cartilage surface of the talar dome. This is useful in identifying unstable and free fragments.
Osteochondral lesions may also involve the tibia. Coronal images that allow separation of tibial and talar chondral surfaces facilitate localization of the lesion to the tibial side of the ankle joint (Fig. 5.102).
Value of MR Imaging
Since conventional radiographs are not sensitive to early lesions, immobilization and surgery may be delayed, resulting in arthritis in 50% of cases. MR imaging of the talar dome is more accurate than CT scanning and complements diagnostic arthroscopic examination of the tibiotalar joint in early diagnosis.
Although conventional radiographs are still the first choice for initial evaluation of patients with suspected osteochondral defects, MR imaging offers the ability to assess both the talar defect and the integrity of overlying cartilage surfaces. MR depiction of an intact articular surface, for example, may assist in the decision to use more conservative treatments, such as drilling, and obviate the need for surgical excision and curettage. Although the ultimate treatment decision in an osteochondral lesion is determined at surgery, preoperative MR imaging can help to determine whether the lesion is loose.
Treatment
Treatment protocols for osteochondral lesions of the talus vary depending on the stage of the lesion and whether it is acute or chronic. Without treatment there is usually progression to joint stiffness and ankle instability. Healing typically results in a stable fragment, although healing plus motion may result in the development of fibrous tissue at the fracture site plus sequestration. Degenerative arthritis is a not uncommon final outcome.
Conservative management is appropriate for stage I and II lesions and medial stage III lesions. For stage I lesions, reduced
activity and limited ankle motion may be sufficient. For acute stage II lesions, casting is usually required.
activity and limited ankle motion may be sufficient. For acute stage II lesions, casting is usually required.
Stage III and IV lesions require surgery for healing. Approaches include:
Free fragment excision
Curettage
Drilling
Abrasion arthroplasty
Microfracture (Fig. 5.103).
A drilled or abraded base permits fibrocartilaginous ingrowth in lesions with a nonreplaceable fragment. Complications include locking of the joint, which occurs with larger bone fragments; degenerative arthritis, which is more likely to occur with lateral, often symptomatic, lesions; and nonunion of the fracture. These often lead to traumatic osteoarthritis.
Arthroscopic treatment of talar dome fractures has relatively low morbidity and complication rates.31 Cystic areas are bone-grafted if cartilage is intact; otherwise, the cyst is corrected with drilling or abrasion of the base. Arthroscopic treatment for acute osteochondral lesions of the talus is different from that for chronic lesions. Acute lesions may require the use of CT or MR imaging to identify the lesion and characterize the size, morphology, and stage. In displaced lesions, arthroscopy is performed with possible open pinning or removal of the osteochondral lesion. Acute lesions are palpated with a small joint probe. The chondral fragment is then assessed
to determine whether there is enough bone to permit healing, if reattachment with absorbable pins, Kirschner wires, or screws is undertaken. Primary chondral lesions without attached bone are excised, with débridement and drilling or abrading of the base. Loose fragments are either fixed with absorbable pins, Kirschner wires, or screws or are excised with drilling of the base. Displaced lesions are excised, and the base is drilled or abraded if it cannot be reattached.
to determine whether there is enough bone to permit healing, if reattachment with absorbable pins, Kirschner wires, or screws is undertaken. Primary chondral lesions without attached bone are excised, with débridement and drilling or abrading of the base. Loose fragments are either fixed with absorbable pins, Kirschner wires, or screws or are excised with drilling of the base. Displaced lesions are excised, and the base is drilled or abraded if it cannot be reattached.
Injuries to the Tendons
A variety of studies have confirmed the usefulness of MR imaging in the evaluation of tendinous and ligamentous struc-tures about the ankle. Intact tendons and ligaments demonstrate low signal intensity on all pulse sequences. The thicker tendons of the ankle can be studied in multiple planes, although smaller ligamentous bands may be seen in only one orthogonal plane.
Achilles Tendon Injuries
The Achilles tendon is subject to both midsubstance tendinosis (manifested by fusiform thickening) and insertional tendinitis (seen as distal thickening at the os calcis insertion) as well as longitudinal, partial, or complete tears, which are optimally evaluated in the sagittal plane. Associated abnormalities include retrocalcaneal bursitis and inflammation of the soft tissues surrounding the tendon (paratendinitis).
Achilles Tendinitis
Pearls and Pitfalls
Achilles Tendinitis
Tendinitis or tendinopathy represents intrasubstance tendon degeneration.
Achilles tendinitis is subdivided into non-insertional tendinosis and insertional tendinitis.
The Achilles tendon has no synovial sheath but is associated with a paratenon or connective tissue envelope.
MR identifies nodular or convex tendon thickening and intratendinous mucoid degeneration.
Haglund’s deformity represents insertional tendinitis with a posterosuperior calcaneal bony prominence and retrocalcaneal tendo Achilles bursitis.
The Achilles tendon does not have a true tendon synovial sheath (Fig. 5.104). Instead, there is a connective tissue envelope surrounding the tendon, which is referred to as a paratenon (or peritenon) (Fig. 5.105). Achilles tendinopathy is classified into the following categories:
In tendinosis or tendinopathy, there is intrinsic or intrasubstance degeneration of the Achilles tendon.
Tendinitis represents the clinical symptoms that develop in association with the degenerative process of tendinosis.
In paratendinitis (also known as peritendinitis, since it refers to the peritendinous tissues), there is generalized inflammation of the tissues surrounding the Achilles tendon (pre-Achilles fat).32
In paratendinitis (peritendinitis) (Fig. 5.106) with tendinosis, there is inflammation of the surrounding tissues with associated tendon degeneration.
The paratenon (also referred to as the peritenon) represents the connective tissue envelope surrounding the Achilles tendon.
Paratenonitis is an inflammation of the Achilles tendon connective tissue envelope (usually limited to the posterior paratenon on MR images).
An irregular pre-Achilles fat pad may be seen with paratendinitis (peritendinitis) with or without abnormal Achilles tendon morphology.
Etiology, Pathology, and Clinical Features
Achilles tendinitis is seen most often in adults 30 to 40 years of age. Young athletes and sedentary and older individuals may
also be at risk. Although it may occur in both men and women, pronounced lesions are more often seen in males. It affects 11% of runners and 9% of dancers. Overuse of the calf muscles is a primary cause, and several mechanisms contribute to overuse injuries, including:
also be at risk. Although it may occur in both men and women, pronounced lesions are more often seen in males. It affects 11% of runners and 9% of dancers. Overuse of the calf muscles is a primary cause, and several mechanisms contribute to overuse injuries, including:
Eccentric loading of a fatigued muscle—tendon unit, seen in overtraining. Runners are susceptible in both acceleration (sprinting) and deceleration (eccentric contraction).
Hyperpronation, in which the leg and foot generate opposing forces of rotation: the subtalar joint pronates, the calcaneus everts, and the knee extends.
Forefoot varus, particularly in cavus foot
Equinus deformity, including triceps surae contracture
Insertional changes, such as a calcaneus bony prominence
Hypertrophic spurring or enthesophytes
Systemic arthropathies, such as HLA-B27 antigen-associated arthritides or rheumatoid arthritis
Additional causes include direct trauma to the Achilles tendon, which may result in hematoma with associated inflammation and fibrosis with restricted tendon sliding. In stenosing tenosynovitis, there is chronic inflammation of the peritenon. Partial tearing, intrasubstance cysts from chronic partial tears,
or nodules may also be identified. New collagen formation and associated fibrillation, nodularity, degeneration, and discoloration contribute to constriction of the peritenon.
or nodules may also be identified. New collagen formation and associated fibrillation, nodularity, degeneration, and discoloration contribute to constriction of the peritenon.
Achilles tendinitis occurs in two locations: non-insertional and insertional. Non-insertional tendinosis (Fig. 5.107) is seen in the hypovascular watershed zone 2 to 6 cm proximal to the calcaneal insertion. The tendon is affected to varying degrees in both the longitudinal extent and anteroposterior thickening within the watershed region of decreased vascular perfusion. Insertional tendinitis (Fig. 5.108) affects the distal calcaneal tendon insertion.
Pathologically, inadequate healing is the key event, leading to adhesions, fibrotic changes and inflammation of the peritenon, loss of the normal tendon luster, nodular thickening, and calcification. Microscopically, chronic paratendinitis is characterized by the development of hypertrophic connective tissue, increased capillary infiltration, regional blood vessel degeneration, fibrinogen deposition and fibrinoid necrosis, a round cell infiltrate, an increase in glycosaminoglycans (chondroitin sulfate) (see Fig. 5.107), and mucoid degeneration. In addition, there is leakage of plasma proteins secondary to disruption of local blood flow. There may be absence of a tendon inflammatory response separate from inflammatory disease of the peritendinous tissues and peritenon.
The retrocalcaneal bursae between the Achilles insertion and calcaneus (Fig. 5.109) and the tendo-Achilles bursa located posterior to the Achilles tendon (Fig. 5.110) may also be involved.
Clinically, patients present with pain with weight-bearing. In non-insertional tendinosis (see Fig. 5.107) there is acute pain with altered biomechanics. Symptoms are most often proximal to the retrocalcaneal bursa, and there is tenderness with deep palpation and palpable tendon nodularity. Non-insertional tendinosis is more likely to occur in higher-level athletes and is found in 6.5% to 18% of runners. Insertional tendinitis tends to occur in older, less athletic, or sedentary individuals, and the tenderness is at the os calcis tendon insertion in Haglund’s deformity.
MR Appearance
MR findings in Achilles tendinitis in-clude focal or fusiform thickening of the Achilles tendon and diffuse or linear low to intermediate signal intensity on T2-weighted, FS PD FSE, or STIR images.33 Key findings include:
Increased cross-sectional diameter on axial images
Increased anteroposterior dimensions
Prominent anterior convexity with focal or diffuse thickening in the sagittal plane
Thickening and intermediate signal of peritendinous tissue dorsal, medial, and lateral to the Achilles tendon on T1- or PD-weighted images
Intermediate-signal-intensity effacement of peritendinous tissue anterior to the Achilles tendon on T1- or PD-weighted images
Hypointense to intermediate signal within an enlarged tendon in hypoxic fibromatosis (Fig. 5.111) on FS PD FSE images
Inflammatory fluid anterior to the tendon and proximal extension of fluid in the retrocalcaneal bursa
Myxoid degeneration (Fig. 5.112) with increased signal on FS PD FSE or STIR images
Calcific (Fig. 5.113) or ossific degeneration with tendon thickening
Associated partial tears
Enthesopathic insertional tendinitis (Fig. 5.114)
Haglund’s deformity (Figs. 5.115, 5.116, and 5.117) (insertional tendinitis with reactive calcaneal marrow edema [see Fig. 5.116], and the constellation of thickened tendon, retrocalcaneal/tendo Achilles bursitis, and a calcaneal bony prominence)
Effacement and edema of the pre-Achilles fat body with normal tendon morphology and/or signal
![]() FIGURE 5.102 ● Coronal FS PD FSE images of an osteochondral lesion of the tibia (A). The subchondral plate fragment is defind on a 2-month follow-up study (B). |
T2* sequences demonstrate increased hyperintensity (relative to other pulse sequences) in areas of tendon degeneration. FS PD FSE images are not as sensitive to intrasubstance signal intensity as are T2*-weighted or STIR techniques. After contrast administration there is soft-tissue inflammatory fluid enhancement in paratendinitis.
In chronic Achilles tendinitis there is focal or diffuse widening of the tendon diameter or contours, but intrasubstance increased signal intensity may not be evident in uncomplicated (not associated with partial tears) chronic lesions. Adhesions between the peritenon and Achilles tendon are associated with chronic peritendinitis. A healed Achilles tendon tear displays
thickening without associated increased signal intensity. It may be difficult to distinguish areas of chronic tendinitis from intrasubstance tendon tears without visualizing either discontinuity in tendon fibers or discrete hyperintense signal intensity on T2-weighted or STIR images with partial tears.
thickening without associated increased signal intensity. It may be difficult to distinguish areas of chronic tendinitis from intrasubstance tendon tears without visualizing either discontinuity in tendon fibers or discrete hyperintense signal intensity on T2-weighted or STIR images with partial tears.
In gout, deposition of tophaceous material (Fig. 5.118) may lead to spontaneous rupture of the Achilles tendon (see also the discussion below on Achilles tendon rupture). Tophi appear similar to nodular myxoid-like degeneration and demonstrate intermediate and increased signal inhomogeneity on T2-weighted, T2*-weighted, or STIR images. Xanthomas (Fig. 5.119) of the Achilles tendon have been shown to produce infiltrative lesions with diffuse tendon thickening and enlarged soft-tissue components (masses).34–34 Bilaterality of these lesions is common. Xanthomas enhance with intravenous gadolinium administration. The soft-tissue component displays hyperintense inhomogeneity on FS PD FSE images.
![]() FIGURE 5.105 ● There is no true synovial sheath around the Achilles tendon. It is covered by the paratenon alone. The normal paratenon is not hyperintense on FS PD FSE images. Axial FS PD FSE image. |
Treatment
Acute tendinitis may progress to chronic tendinopathy, which is characterized by perfusion insufficiency with vascular proliferation, abnormal tendon fiber morphology, and hypercellularity. Tendinopathy is symptomatic in 90% of cases, and partial tears are associated with regions of tendinosis. Tendinosis may be a precursor lesion to a final stage of partial or complete tendon tear.
Conservative treatment, used to alleviate the symptoms of tendinopathy, includes therapeutic rest, stretching exercises, cross-training or alternative exercise, anti-inflammatory medications, immobilization, orthoses to correct hyperpronation, and brisement to lift an adherent paratenon. If conservative treatment is not successful, surgical options include tendon release with or without stripping and excision of the thickened peritenon and granulation tissue, excision of the calcaneal prominence in insertional tendinopathy, repair of any associated partial tears, and débridement of mucoid degeneration.
![]() FIGURE 5.110 ● (A) Anatomy of the retrocalcaneal and subcutaneous bursae. (B) Hyperintense retrocalcaneal bursa and more linear tendo-Achilles bursa on a sagittal FS PD FSE image. |
Partial and Complete Tears of the Achilles Tendon
Pearls and Pitfalls
Partial and Complete Achilles Tendon Tears
Achilles tendon rupture usually occurs 2 to 6 cm proximal to its os calcis insertion.
Sagittal images are used to identify the proximal and distal tendon ends.
Axial FS PD FSE images are used to confirm complete rupture (an intact plantaris may simulate an intact tendon in the sagittal plane).
Partial Tears of the Achilles Tendon
Partial tendon tears can be defined on MR images in the sagittal and axial planes. Linear or focal regions of increased signal and thickening of fibers without a tendinous gap are characteristic.37 Partial tears may involve either the anterior (Fig. 5.120) or posterior (Fig. 5.121) tendon surface. A decreased tendon diameter without loss of tendon continuity is seen in more extensive partial tears (Fig. 5.122). Incomplete tears may also present with partial continuity of a portion of the tendon fibers on at least one sagittal image. Longitudinal splits in chronic Achilles tendinitis that are low to intermediate in signal intensity on long-TR/TE images may be seen in association with a superimposed acute partial tear. The acute partial tear is hyperintense on long-TR/TE images.
Achilles Tendon Rupture
The Achilles tendon, formed by the confluence of the gastrocnemius and soleus muscle complexes, is the largest tendon in the body and is the third most frequently ruptured tendon. Injuries to this tendon are most commonly seen secondary to athletic activity in middle-aged (after 30 years of age and most frequently between 30 and 50) men.38,39 Sports involving concentric loading (such as basketball, tennis, or racquetball) are frequently implicated. The male-to-female ratio is 5:1 to 6:1 for complete rupture secondary to indirect trauma. In younger individuals, the musculotendinous junction is more commonly injured. Disorders such as rheumatoid arthritis, systemic lupus erythematosus, diabetes mellitus, and gout may predispose to disruption of the Achilles tendon because of already weakened connective tissue and collagen fibers. The Achilles tendon is most susceptible to rupture 2 to 6 cm superior to the os calcis. Acute rupture is associated with forced dorsiflexion of the foot against a contracting force generated by the triceps surae group. Rupture of the contracted musculotendinous unit may occur secondary to direct trauma.
Etiology, Diagnosis, Pathology, and Clinical Features
In evaluating Achilles tendon rupture and healing, it is important to remember that the tendon has no true synovial sheath, the mesotendon provides the blood supply through the anterior mesentery, and there is a triangular fat pad anterior to the Achilles tendon called Kager’s fat triangle (see Fig. 5.106).
The most common causes of Achilles tendon tears are:
Indirect trauma
Repetitive microtrauma
Overpronation of the foot or heel stress leading to microtears
Forced dorsiflexion of the foot against a contracting force (triceps surae group) or eccentric loading in a sudden stretch
Direct trauma, causing rupture at the myotendinous junction
Soleus muscle atrophy
Rheumatoid arthritis, systemic lupus, diabetes mellitus, and gout
Chronic tendinitis and partial tears are important predisposing causes in acute rupture.
Fluoroquinolone antibiotics (and statin drugs, retinoids, and calcium channel blockers) have been linked to Achilles tendon tears.
![]() FIGURE 5.113 ● Thickened calcific tendon degeneration in association with distal tendon rupture. Sagittal T1-weighted image. |
On pathologic examination a tear is found 2 to 3 cm proximal to the calcaneal insertion. Tears may be related to a reduction in the anterior mesenteric blood supply with increasing age. The posterior fibers rupture first (partial tearing may exist in anterior fibers, which are under less tension), and the plantaris usually remains intact because of its more anterior calcaneal insertion. Histologic examination reveals degeneration with decreased collagen cross-linking, resulting in increased stiffness and loss of viscoelasticity.
The typical patient is a middle-aged man participating in athletic activity. Patients present with pain and soft tissue swelling, usually related to hemorrhage. With clinical examination alone, rupture of the Achilles tendon is missed in up
to 25% of cases, perhaps because the other tendons of the posterior calf maintain plantarflexion and allow a tendinous gap to be missed on clinical examination. Clinical signs include the hyperdorsiflexion sign, O’Brien’s needle test to detect proximal tendon motion as a sign of tendon continuity in acute ruptures, a palpable tendon defect, and Thompsen’s sign. The Thompsen test is performed in the prone position and is positive if squeezing the calf does not produce the normal plantarflexion response.
to 25% of cases, perhaps because the other tendons of the posterior calf maintain plantarflexion and allow a tendinous gap to be missed on clinical examination. Clinical signs include the hyperdorsiflexion sign, O’Brien’s needle test to detect proximal tendon motion as a sign of tendon continuity in acute ruptures, a palpable tendon defect, and Thompsen’s sign. The Thompsen test is performed in the prone position and is positive if squeezing the calf does not produce the normal plantarflexion response.
![]() FIGURE 5.122 ● Attenuated anterior-to-posterior tendon thickness in an extensive partial Achilles tendon tear without a tendinous gap. (A) Sagittal T1-weighted image. (B) Axial FS PD FSE image. |
Lateral radiography and xerography have not been effective in identifying abnormalities in the Achilles tendon. Although tendon thickening in inflammation and discontinuities in tears have been observed with some success using real-time ultrasonography, this technique is limited in soft-tissue contrast discrimination and FOV, and by the inability to accurately evaluate both adjacent soft-tissue and bony structures.
Staging
A four-stage classification system has been developed to grade Achilles tendon ruptures:
Type 1: Partial ruptures affecting 50% or less of the tendon (Fig. 5.123)
Type 2: Complete ruptures with a tendinous gap of 3 cm or less (Fig. 5.124)
Type 3: Complete ruptures with a tendinous gap of 3 to 6 cm (Fig. 5.125)
Type 4: Complete tendon ruptures with a defect greater than 6 cm (Fig. 5.126). Type 4 is associated with neglected ruptures.
![]() FIGURE 5.123 ● Partial Achilles tendon tear with the “corkscrew” morphology of partially retracted fibers. Coronal FS PD FSE image. |
![]() FIGURE 5.124 ● Focal complete tear of the Achilles tendon with less than 3 cm of retraction. (A) Lateral color graphic. (B) Sagittal T1-weighted image. (C) Axial FS PD FSE image. |
MR Imaging Technique
The Achilles tendon can be demonstrated on sagittal, axial, and thin-section coronal MR images.40 A routine examination of the tendon uses a 14- to 16-cm FOV and sections 3 to 4 mm thick. A T1-weighted sequence is performed in the sagittal plane through the Achilles tendon. T2*-weighted, FS PD FSE, or STIR images show associated hemorrhage or edema in the intratendinous or peritendinous soft-tissue structures. FS PD FSE or STIR axial sequences reveal fluid, hemorrhage, or inflammatory tendon changes and are useful in the evaluation of the integrity of other tendons and ligaments supporting the ankle. Thin-section (3 mm) coronal images display the width of the Achilles tendon and the condition of disrupted tendon fibers. In one study, preoperative MR imaging findings, including tendon morphology, orientation of torn fibers, and measurement of tendon diastases, all correlated with surgical findings during tendon repair.41
MR Appearance
The normal Achilles tendon demonstrates uniform low signal intensity. Axial images show the tendon in cross-section with a mildly flattened anterior surface and a convex posterior surface. In ruptures of the Achilles tendon, the relationship of the proximal and distal portions of the torn tendon can be seen on MR studies either before or after application of a plaster cast. Key MR findings include:
A fluid-filled gap with or without interposed fat at the tear site in complete tendinous disruptions with discontinuity
Fraying or corkscrewing (see Fig. 5.123) of the tendon edges associated with proximal tendon retraction
In the absence of overlapping tendon edges, no tendon fibers can be seen at the tear site on axial images.
Tendon disruption with discontinuity and a wavy retracted tendon
Associated hemorrhage or edema in intratendinous or peritendinous soft tissues on axial or sagittal images
Disruption of muscle fibers in a musculotendinous junction tear, although on gross examination the tendon appears intact
Effacement of Kager’s triangle18
Intratendinous degeneration
In addition, there may be overlapping or nondisplaced proximal and distal tendon components (Fig. 5.127). Enlarged proximal and distal ends may be associated with an attenuated union bridging the tear site. Associated soleus muscle strain (Fig. 5.128) is also sometimes seen.
After surgery MR can also be used to follow healing. Intratendinous fluid may be seen for up to 6 months after treatment and tendon widening may be visible for up to 12 months. An increased size of the tendon associated with decreased tendinous signal intensity is secondary to scar tissue. Distal repairs are associated with distal tendon thickening and residual signal intensity (Fig. 5.129).
![]() FIGURE 5.127 ● Partial overlap of torn Achilles tendon ends. (A) Lateral color illustration. (B) Sagittal FS PD FSE image. |
The main differential features between partial and complete tears include the following:
Partial tears demonstrate hyperintense signal with incomplete anterior-to-posterior or posterior-to-anterior extension on FS PD FSE images.
Complete tears demonstrate a hyperintense fluid-filled tendinous gap.
Tendon rupture usually occurs 2 to 6 cm superior to the os calcis.
The size of the rupture varies, based on the degree of tendon retraction.
Ruptures demonstrate diffuse convexity of the anterior margin and enlarged tendon ends at the tear site.
Treatment
MR imaging may be most useful in Achilles tendon rupture in the selection of patients best suited for conservative therapy, thereby improving the present statistics for rerupture.42,43 Retear of a primary repaired tendon shows residual suture artifact.
Conservative or nonoperative measures may be recommended for acute ruptures, less than 48 hours old, and for steroid-induced ruptures.44 Cast immobilization with an above-the-knee cast with equinus for 4 weeks, or a below-the-knee cast with decreased equines, is the main approach. Surgical repair is usually required. An end-to-end anastomosis is recommended for type 1 and 2 ruptures; an autogenous tendon graft flap is used for type 3 ruptures; and a free tendon graft or synthetic graft is needed for type 4 ruptures. Suture techniques include Bunnell, Kessler, Krackow (locking loop) with four sutures across the repair site, percutaneous repair, and use of synthetic graft materials. Alternative function bracing and plantaris reinforcement may also be part of surgical treatment.
In making a decision about surgery versus serial casting, MR is used to determine the degree of apposition of the disrupted proximal and distal tendon fragments. Retracted tendon sections are less likely to heal with conservative management, and MR evaluation allows early identification of these patients, who are candidates for surgical intervention. Fibrous bridging also appears to be more tenuous with increased tendon separation. A bulbous contour may be demonstrated in the proliferating ends of a torn Achilles tendon, and it increases with greater tendon diastases. Fibrous healing, with approximation of torn fibers, can be evaluated with MR imaging performed at monthly intervals. The healing of conservatively or surgically managed tendons may be associated with intratendinous fluid
spaces, studied at 3 and 6 months by Dillon et al..45 Over time, decreased signal intensity was found in the areas of successful healing. By 12 months, these areas demonstrated low signal intensity within a widened tendon. Caution must be exercised in interpreting MR images obtained in the first 2 months postoperatively; Achilles tendon pathology should not be overread as retear, poor healing, or failed surgical repair.46
spaces, studied at 3 and 6 months by Dillon et al..45 Over time, decreased signal intensity was found in the areas of successful healing. By 12 months, these areas demonstrated low signal intensity within a widened tendon. Caution must be exercised in interpreting MR images obtained in the first 2 months postoperatively; Achilles tendon pathology should not be overread as retear, poor healing, or failed surgical repair.46
![]() FIGURE 5.128 ● Grade 1 soleus muscle strain secondary to an Achilles rupture. Axial FS PD FSE image. |
![]() FIGURE 5.129 ● Residual post-surgical Achilles tendon thickening with an intratendinous scar. (A) Sagittal T1-weighted image. (B) Sagittal FS T1-weighted contrast enhanced image. |
The Achilles tendon and overlying skin are relatively avascular and may become infected after surgery with implanted material or autogenous tendon. The incidence of complications with surgical repair is reported to be 20%. The incidence of rerupture of the Achilles tendon is significantly higher in patients who undergo nonsurgical treatment than in those who have had surgical repair. Surgery is the preferred treatment for any patient who has a rerupture of the Achilles tendon treated conservatively, and possibly should be the primary treatment choice for any patient who is active and athletic.47 Chronic thickening of the Achilles tendon or residual inflammatory changes are demonstrated on axial and sagittal MR images. After repair with strands of a polymer of lactic acid (PLA), T1-weighted images show a thickened fusiform tendon with moderate-signal-intensity streaks.48 Changes in signal intensity are attributed to the PLA implant and surrounding collagenogenic response.
Tibialis Posterior Injuries
Pearls and Pitfalls
Tibialis Posterior Injuries
Tendinopathy and progressive tendon tear results in a collapsed pes valgus deformity.
Tibialis posterior tendon tears occur at or just distal to the medial malleolus.
Tears are classified as type 1 (enlarged tendon), type 2 (attenuated tendon), or type 3 (complete rupture).
Associated medial malleolar spur may contribute to tendon degeneration.
Subtendons are evaluated on axial images.
Rupture of the tibialis posterior tendon (posterior tibial tendon [PTT]), which may occur spontaneously, is commonly associated with prior synovitis, steroid injection, or trauma.49 Chronic PTT rupture usually occurs in middle-aged women in their fifth or sixth decades. Sixty percent of cases are associated with hypertension, diabetes, and obesity.
Etiology, Pathology, and Clinical Features
In assessing tibialis posterior injuries, it important to remember that the tibialis posterior is located superficial to the superomedial calcaneal navicular component of the spring ligament and that its relationship with the spring ligament is synergistic. The PTT inserts proximally on the navicular tuberosity, and there are also extensive plantar insertions. Functionally, the PTT supports the medial arch and stabilizes the foot. It is also responsible for supination of the foot and, with the medial malleolus, for pulley actions.
The most commonly seen indications of tibialis posterior injuries are a change in tendon size, morphology, and/or signal intensity. Most lesions are located at the midportion of the tendon at the level of and immediately distal to the medial malleolus, corresponding to the relative zone of tendon hypovascularity. The normal PTT is twice the size of the FDL. Tears presents as a spectrum of tendon hypertrophy, attenuation, or disruption. Morphologic characteristics include bulbous cross-sectional enlargement, attenuation in a curvilinear orientation, and a complete tendinous gap.
There are a number of causes of posterior tibialis failure, including:
Chronic degeneration
Trauma
Systemic disease
Tendon hypovascularity
Biomechanical disorders and abnormal insertions
Iatrogenic conditions
Chronic degeneration is the most frequent etiology of tibialis posterior dysfunction. Acute trauma is less commonly involved, and although minor trauma, such as lacerations and puncture wounds, is sometimes implicated, rupture is usually associated with indirect trauma such as sprains and/or fractures. Rarely, traumatic dislocation of the PTT can be attributed to forced dorsiflexion with inversion.
Patients frequently have associated systemic disorders, such as chronic inflammatory disease, rheumatoid arthritis, seronegative spondyloarthropathies (including psoriasis, ankylosing spondylitis, Reiter’s disease, and enteropathic arthritis), and infections (particularly gonococcal and tubercular). Tibialis posterior failure has also been found at sites of enthesopathy.
Tendon hypovascularity also contributes to failure. There is a zone of hypovascularity in the midportion of the tendon, at and distal to the medial malleolus, that is at particular risk for rupture. Diminished arterial perfusion (age-related) leads to tendon ischemia, and acute angulation of the tendon posterior to the medial malleolus compounds the problem. In addition, pronation of the hindfoot causes tendon compression.
Biomechanical causes include overuse, pes valgus, equinus deformity, and increased stress on the tendon with decreased medial longitudinal arch support. Abnormal insertions, such as an accessory navicular or a prominent medial navicular tubercle, are associated with tendon degeneration secondary to a change in leverage.
Iatrogenic tendon dysfunction is caused by medial ankle/tarsal tunnel surgery and sometimes by the use of corticosteroids.
On pathologic examination there is usually a longitudinal split with tendon laxity, fibrosis, and elongation. An irregular surface can be identified, and there are adhesions with the tendon sheath and flexor retinaculum. In addition, there is bone proliferation and atrophy of the sheath, and the scarred and frayed proximal and distal ends of the tendon rupture can be identified. Intrasubstance degeneration is noted on histologic examination.
The typical patient is a middle-aged woman who presents with unilateral, often left-sided flatfoot deformity accompanied by pain, swelling, and tenderness and no history of trauma.50 There is flattening of the medial longitudinal arch with weakness of inversion, a severe pes planus deformity, heel valgus, talar plantarflexion, and forefoot abduction. There is intrinsic degeneration of the tendon, and the typical site of rupture is either at or within 6 cm proximal to its navicular insertion. It is the collapse of the medial longitudinal arch that creates the characteristic flatfoot deformity with associated heel valgus, talar plantarflexion, and forefoot abduction.51 Failure of calcaneal inversion with a valgus heel is observed on clinical examination when the patient stands on tiptoe. The clinical diagnosis is not straightforward, and in some cases several years may elapse between initial presentation and diagnosis.
Edema or soft-tissue thickening may be demonstrated inferior to the medial malleolus, although conventional radiographs do not demonstrate tendon pathology. Loss of a convex arch may be observed on weight-bearing views through the talonavicular or naviculocuneiform joint. Radiographs may also reveal a decreased calcaneal plantar angle, an increased lateral talometatarsal angle, an increased anterior talocalcaneal angle, and an increased lateral talocalcaneal angle.51 Forefoot abduction is associated with lateral subluxation of the navicular at the talonavicular joint, which is shown on axial images through the midfoot. The presence of an accessory navicular bone is associated with both pes planus and PTT dysfunction.
Tenosynovitis may also involve the tibialis posterior and peroneal tendons. Tibialis posterior tenosynovitis is associated with rheumatoid arthritis and planovalgus foot and usually occurs in older patients.
Classification
PTT tears have been divided into three classification types based on MR criteria. Types I and II are partial tears and type III is a complete tear. In comparison with CT scans, and using surgical exploration as the gold standard, MR imaging is superior in detecting the spectrum of early partial tendon ruptures, longitudinal tearing, and the presence of synovial fluid.52 Subtle areas of associated periostitis, however, are more readily delineated with CT.
Specific findings in the various tear types include the following:
Type 1: There is tendon hypertrophy with heterogeneous signal intensity in intrasubstance vertical splits (Fig. 5.130). Associated findings include increased signal intensity (intrasubstance striations) and girth at the distal tendon insertion to the navicular (a normal variant), osseous spurring with or without fatty marrow signal in the posteromedial aspect medial malleolus (see Fig. 5.130), and tendon dysfunction or dislocation with disruption of the flexor retinaculum (Fig. 5.131).
Type 2: There are thin or attenuated sections of the tendon at the level of the medial malleolus53 with variable intratendinous signal change (Fig. 5.132). Subtendons may occur associated with mixed areas of atrophic and hypertrophic tendon segment (Fig. 5.133).
Type 3: There is complete tendinous discontinuity with a low- to intermediate-signal-intensity fluid-filled gap (Fig. 5.134). There may be subtendons associated with irregular tendon morphology of the retracted proximal or distal segments on either side of the tendinous gap. Digital avulsion (Fig. 5.135) in the foot at the insertion into the cuneiforms, cuboid, and medial metatarsal bases is uncommon.
Chronic dysfunction: In spring ligament laxity or rupture, the superomedial calcaneal navicular component is usually injured. The sinus tarsi syndrome may occur after progression from PTT dysfunction to spring ligament pathology. A more detailed discussion of spring ligament pathology can be found later in the chapter.
MR Appearance
In complete tears, MR imaging demonstrates disruption of the PTT with or without abnormal morphology
of the ends of the tendon.37 Partial or chronic tears or a retracted tendon may present with enlargement. FS PD FSE axial images that extend inferior to the medial malleolus should demonstrate the normal PTT anterolateral to the FDL tendon.
of the ends of the tendon.37 Partial or chronic tears or a retracted tendon may present with enlargement. FS PD FSE axial images that extend inferior to the medial malleolus should demonstrate the normal PTT anterolateral to the FDL tendon.
The tendon hypertrophy seen in type I partial tears is best appreciated on axial images as an increased cross-sectional diameter. An increase in central intrasubstance signal intensity may also be seen on T1-weighted and often on FS PD FSE or STIR images. In a common pattern of presentation associated with prominent or long-segment longitudinal splits of the tibialis posterior in type I tears, three medial flexor tendons instead of two flexor tendons are seen at or proximal to the level of the medial malleolus. Proximal to the split, the PTT is enlarged and seen as a single tendon.
Longitudinal splitting of the PTT into two subtendons is seen in type II tears of the tibialis posterior. Type II partial tears, in which the PTT is attenuated or reduced in size, are usually identified at the level of the medial malleolus. A nonconcentric reduction in tendon width may give the tendon an elongated appearance, without any associated intrasubstance hyperintensity. In these type II tears, the two subtendons are usually located proximal to the medial malleolus, and a single attenuated PTT is identified at the level of the medial malleolus. A medial malleolus periostitis or spur may contribute to the spectrum of tendon degeneration preceding rupture. The native PTT is seen directly posterior to the medial malleolus, and the torn or split fragment is seen posterior to the tendon. The normal FHL tendon is the most posterior and laterally positioned of the three tendons. Normally, only two tendons (the PTT and FDL) should be visualized medially.
Complete (or type III) tears of the PTT demonstrate a discontinuity on axial, coronal, and sagittal images. Tendon retraction and a fluid-filled gap are present on FS PD FSE, T2*, or STIR images. Schweitzer et al.46 reported the following additional, secondary, signs of rupture:
Prominence or hypertrophy of the medial tubercle of the navicular (sensitivity 89%, specificity 75%)
Abnormalities of talonavicular alignment (sensitivity 82%, specificity 100%)
Presence of an accessory navicular (sensitivity 20%, specificity 100%)
Normal talonavicular alignment can be determined by a line drawn through the long axis of the talus, which typically bisects the navicular bone. Schweitzer et al.54 additionally found that there is an overlap in the range of normal and abnormal distal tibialis posterior tendon size. There is also overlap in the range of intratendinous signal intensity in normal and torn PTT. This overlap is primarily observed on T1-weighted images and is not observed on FS PD FSE. In clinical practice, tears of the PTT are usually evident proximal to the navicular and diagnosis can be made without reliance on characterizing the bulbous configuration of the distal attachment.
Intrinsic degenerations usually demonstrate varying degrees of increased internal signal intensity with fusiform enlargement. Fluid in the tendon sheath, which demonstrates increased signal intensity on conventional T2, FS PD FSE, and T2*-weighted images, may be observed in patients with tenosynovitis. Laxity or rupture of the plantar calcaneonavicular or spring ligament (see below) is associated with chronic dysfunction of the PTT55 and may lead to a pes planovalgus deformity.
Treatment
The natural course of PTT injury includes a change in the shape of the foot, decreased exercise tolerance, plantar fasciitis, severe pes planus, tenderness of the sinus tarsi, and lateral ankle pain in advanced deformity.
Conservative treatment, which frequently fails, may be attempted if the tendon is intact and without significant degeneration. Approaches involve support of the medial longitudinal arch with orthoses such as a medial heel wedge, nonsteroidal anti-inflammatory drugs (NSAIDs), and physical therapy.
Most patients require surgery, which may include osseous stabilization (i.e., osteotomies with double or triple arthrodesis) with hindfoot arthrodesis, primary repair, or replacement of the ruptured tendon. Excision of segmental defects with a side-to-side anastomosis to the FDL or transfer of the FDL with suturing of its distal stump to the FHL may also be attempted. Treatment of chronic dislocation, which occurs anterior to the ruptured flexor retinaculum and FDL tendon, involves reconstruction of the flexor retinaculum and deepening of the groove around the medial malleolus, as described by Healy et al.56
Spring Ligament Complex and Pathology
Pearls and Pitfalls
Spring Ligament Complex and Pathology
The spring ligament complex consists of three components: the lateral, intermediate, and superomedial oblique calcaneonavicular ligaments.
Degeneration of the spring ligament complex is associated with posterior tibial (tibialis posterior) tendinopathy.
The spring ligament complex comprises three distinct components: the lateral, intermediate, and superomedial oblique calcaneonavicular ligaments (Figs. 5.136 and 5.137). These
structures are critical static stabilizers of the medial longitudinal arch of the foot, providing support for the head of the talus at the talocalcaneonavicular joint (or acetabulum pedis). The other major stabilizer of the medial longitudinal arch, the PTT, is a dynamic stabilizer. Pathology of the spring ligament complex rarely occurs in isolation and is almost always associated with PTT dysfunction. Attention to the components of the spring ligament on routine MR imaging of the foot is important, since there is often a cascade of failures that can lead to or be seen with acquired pes planus deformity.
structures are critical static stabilizers of the medial longitudinal arch of the foot, providing support for the head of the talus at the talocalcaneonavicular joint (or acetabulum pedis). The other major stabilizer of the medial longitudinal arch, the PTT, is a dynamic stabilizer. Pathology of the spring ligament complex rarely occurs in isolation and is almost always associated with PTT dysfunction. Attention to the components of the spring ligament on routine MR imaging of the foot is important, since there is often a cascade of failures that can lead to or be seen with acquired pes planus deformity.
In the literature, the nomenclature has not been consistent regarding the lateral and intermediate calcaneonavicular ligaments. The lateral calcaneonavicular ligament is also termed the inferoplantar longitudinal calcaneonavicular ligament 57 and the inferior calcaneonavicular ligament.58,59 The intermediate calcaneonavicular ligament is also termed the plantar medial oblique calcaneonavicular ligament 58 and the “third ligament. ”57 For purposes of clarity the ligaments will be referred to with respect to their relative navicular attachment sites.
![]() FIGURE 5.136 ● Spring ligament complex anatomy. Lateral-plantar oblique (A), plantar (B), and superior (C) views. |
The inferior (lateral and intermediate) calcaneonavicular ligaments originate adjacent to each other from the notch between the anterior and middle articular facets of the calcaneus. The lateral calcaneonavicular ligament is a short ligament that travels longitudinally to attach to the lateral and proximal aspect of the navicular bone (the “beak” of the navicular). This ligament is best visualized on sagittal and axial images on routine ankle MR examination. The intermediate calcaneonavicular ligament is a longer ligament that travels obliquely across the floor of the talocalcaneonavicular joint to attach on the lateral and plantar aspect of the tuberosity of the navicular bone. This ligament is best seen on axial and coronal images. Abnormalities in these components of the spring ligament complex are not commonly seen on MR imaging, and there are no current reports in the literature regarding surgical repair of these structures.
The superomedial calcaneonavicular ligament originates from the medial aspect of the sustentaculum tali. It then extends distally in an oblique orientation as it wraps around the tuberosity of the navicular to attach along the superomedial aspect of the navicular bone. The tibiospring ligament, part of the superficial fibers of the deltoid ligament, merges with the superficial and medial fibers of the superomedial calcaneonavicular ligament near its origin from the sustentaculum. The superomedial calcaneonavicular ligament is often difficult to visualize, and evaluation in all three imaging planes is required. It is often best seen on the coronal and axial images just deep to the PTT. Abnormalities in the superomedial calcaneonavicular ligament are commonly seen in cases where there is PTT pathology. Surgical repair of this ligament is now often being attempted along with repair of the PTT to improve the static support to the longitudinal arch of the foot.59,60
Complete tears involving components of the spring ligament complex are typically clearly apparent on MR imaging as a fluid-filled gap in the ligament(s) and, depending on acuity, associated high intensity signal (edema) in the surrounding soft tissues. As mentioned, full-thickness tears of the spring ligament complex very rarely occur in isolation. Typically, there is associated pathology (tendinosis, strain, or tear) of the PTT.
Sprains and partial tears of the components of the spring ligament complex are a more difficult diagnostic dilemma on MR because there is significant overlap between normal and abnormal tendon morphologic characteristics. Findings include thickening, high signal, or heterogeneous signal within the spring ligament (Fig. 5.138A). Associated abnormalities in the PTT or reactive marrow edema in the talar head are useful in determining if the findings involving the spring ligament components indicate true pathology.
Degeneration of the spring ligament complex is more common than traumatic tears or stains. These degenerative changes are also typically seen in association with pes planus deformity and posterior tibial tendinopathy. In these instances the component ligaments demonstrate abnormal heterogeneous signal, attenuation, or thickening (Fig. 5.138B, C). Again, there is a great deal of overlap with surgically normal ligaments, and attention to associated abnormalities may help guide the diagnosis.
Flexor Hallucis Longus Abnormalities
Pearls and Pitfalls
Flexor Hallucis Longus Abnormalities
Tenosynovitis is associated with hyperintense fluid in the FHL synovial sheath disproportionate to the quantity of tibiotalar joint fluid.
Chronic muscle changes and acute tenosynovitis are associated with hyperplantar flexion in ballet dancers.
The distal course of the FHL should be examined on coronal images to exclude a distal rupture at its third or lost fibro-osseous tunnel.
The FHL tendon is associated with a fibro-osseous tunnel and runs deep to the flexor retinaculum (between the medial and lateral tubercles of the posterior talar process) (Fig. 5.139). It passes underneath the sustentaculum tali to its plantar insertion at the base of the distal phalanx and great toe. The sheaths of the FHL and FDL cross at the master knot of Henry (see Fig. 5.139). The os trigonum is an ununited lateral tubercle in the posterior aspect of the talus found in 10% of individuals. FHL injuries at the level of the medial malleolus are seen in young athletes and dancers in conjunction with active plantarflexion mechanisms of injury. They are more common in female than male dancers. Hallux saltans refers to the development of a nodule or partial tear with triggering of the big toe.
Etiology, Pathology, and Clinical Features
The fibroosseous tunnel of the FHL runs between the medial and lateral posterior talar tubercles. Abnormalities are restricted to either the muscle or the tendon sheath.61 Muscle involvement may be focal or may involve the entire cross-sectional diameter. Tendon sheath involvement is variable. Muscle—tendon unit injuries are characterized by diffuse edema with or without focal muscle hemorrhage, and there may be accompanying tenosynovitis with a circular to elongated fluid collection.
Etiologic factors in FHL abnormalities include twisting injuries to the ankle and calcaneal fracture with involvement of the sustentaculum tali. Other causes include repetitive plantar-flexion and dorsiflexion that lead to tenosynovitis, a low-lying FHL muscle that is at risk for impingement, partial tearing or thickening with nodule development and hallux triggering (hallux saltans), and a fixed or locked FHL within the sheath (associated with severe tenosynovitis) (Fig. 5.140). The condition is exacerbated by hyper-plantarflexion.
In dancers, posterior impingement or talar compression syndrome results from full weight-bearing in maximum ankle plantarflexion in demi-pointe or full en pointe position.62 This is accentuated in the presence of an os trigonum, laxity of the lateral ligaments, and/or soft-tissue entrapment between the posterior lip of the talus and the os calcis. A fixed tethering of the FHL tendon proximal to the flexor retinaculum produces a check-rein deformity with a flexion contracture of the interphalangeal joint of the hallux and an extension contracture of the first metatarsophalangeal joint.63 The FHL is sometimes called the Achilles tendon of the foot or “dancer’s Achilles heel” (see Fig. 5.140; Fig. 5.141).
Paratendinitis (tenosynovitis) remains the most common abnormality (Figs. 5.136 and 5.137). With tendinosis and partial tears there is tendon thickening (Fig. 5.144), and with stenosing tenosynovitis there is fluid proximal to the talar fibro-osseous tunnel (Fig. 5.145). Complete tendon rupture in the talar fibro-osseous tunnel is uncommon (Figs. 5.136 and 5.137), and midfoot rupture at the distal insertion is more frequently seen. Paratenonitis occurs in three anatomic sites:
Fibro-osseous tunnel (first fibro-osseous tunnel) between the medial and lateral tubercles of the posterior talar process
Deep to the flexor retinaculum (see Fig. 5.143)
The level of the sesamoids within the distal hallux tunnel (third fibro-osseous tunnel) (see Fig. 5.146)
The clinical presentation is dominated by pain, swelling, and tenderness of the posterior ankle.
MR Appearance
Tearing of the FHL has been demonstrated on axial MR images at the level of the musculotendinous junction.37,64 The FHL is a plantarflexor of the first toe and participates in plantarflexion and inversion of the foot. Rupture of this tendon may be difficult to identify on clinical examination without the assistance of MR localization. A distal rupture requires coronal images performed through the distal foot in addition to routine axial images through the tibiotalar joint.
MR imaging usually demonstrates communication of fluid between the FHL tendon sheath and the tibiotalar joint, commonly between the tibial plafond and the calcaneus. On FS PD FSE images the circular to elongated fluid collection generally demonstrates uniform hyperintensity with or without septations. The volume of fluid in the FHL tendon sheath is disproportionate relative to the tibiotalar joint (Fig. 5.148). Muscle strains are characterized by hyperintense edema or hemorrhage radiating from the trauma epicenter on FS PD FSE images. Foci of hemosiderin may also be found within the muscle.
In addition to tendinitis, ballet dancers may have muscle strains at or near the musculotendinous junction. FS PD FSE and STIR sequences are the most sensitive techniques in these injuries. In os trigonum syndrome (discussed in more detail below), there is hypertrophy of the os trigonum, resulting in a partial tethering of the FHL tendon.63
Treatment
Repetitive stress may result in chronic muscle strain with or without hemorrhage, stenosing tenosynovitis, and partial or complete tendon tears. Conservative approaches to treatment include the use of anti-inflammatory agents, rest, modification of dance or related causative activities, and immobilization. When surgery is required, procedures include release of the constricting FHL tendon sheath and, if required, excision of an associated symptomatic os trigonum.
Tibialis Anterior Tendon Injuries
Pearls and Pitfalls
Tibialis Anterior Tendon Injuries
Rupture occurs between the extensor retinaculum and the insertion onto the medial first cuneiform and the adjacent base of the first metatarsal.
It is important to differentiate between a partial and complete rupture using axial and coronal oblique images.
The tibialis anterior tendon inserts onto the medial cuneiform (Figs. 5.136 and 5.137) and functions in ankle dorsiflexion and inversion of the subtalar and metatarsal joints. It receives its blood supply exclusively from the anterior tibial artery, which puts it at risk for ischemia (Fig. 5.151). Rupture of the tibialis anterior tendon can occur between the extensor retinaculum and the insertion onto the medial first cuneiform and adjacent base of the first metatarsal. Weakness of dorsiflexion, localized tenderness, and drop foot gait are observed on clinical evaluation. The musculotendinous junction at the level of the middle to distal third of the tibia is a rare site of tendinosis.
Etiology, Pathology, and Clinical Features
Anterior tibialis tendon ruptures account for only 1% of muscle and tendon injuries. Spontaneous rupture is rare but may occur in association with diabetes, gout, or rheumatoid arthritis. It is usually seen in individuals over 45 years of age and more commonly in those over 60 years of age. Tibialis tendon ruptures may also occur in athletes, usually males, particularly
runners, soccer players, and hikers. Steroid injections also predispose to tendon rupture. The usual mechanisms of injury are eccentric contraction during midfoot and forefoot forced plantarflexion63 and repetitive dorsiflexion and plantarflexion, because plantarflexion places eccentric stress on the tibialis anterior. Rupture may also be related to abrasion from the inferior edge of the extensor retinaculum or from mechanical irritation, such as occurs from ski boots and hockey skates. Traumatic lacerations, related to the superficial and anterior location of this tendon, may also occur. Although the rupture usually occurs proximal to the insertion, no zone of hypovascularity has been documented.
runners, soccer players, and hikers. Steroid injections also predispose to tendon rupture. The usual mechanisms of injury are eccentric contraction during midfoot and forefoot forced plantarflexion63 and repetitive dorsiflexion and plantarflexion, because plantarflexion places eccentric stress on the tibialis anterior. Rupture may also be related to abrasion from the inferior edge of the extensor retinaculum or from mechanical irritation, such as occurs from ski boots and hockey skates. Traumatic lacerations, related to the superficial and anterior location of this tendon, may also occur. Although the rupture usually occurs proximal to the insertion, no zone of hypovascularity has been documented.
Morphologic and pathologic findings include:
A high-grade partial to complete tear (normal width 1 cm) with tendon retraction
A mass effect produced by protrusion of the tendon relative to the inferior extensor retinaculum
Secondary inflammation of adjacent tendon sheaths
Hemorrhage
An empty tendon sheath
Dorsal exostosis
Medial cuneiform morphology with ridge contour associated with distal tendon degeneration of a partial tear (Fig. 5.152)
Histologic evidence of peritenonitis
Inflammatory changes but no intrinsic tendon degeneration
Clinically, patients present with weakness with attempted dorsiflexion, local tenderness and foot drop, and a palpable mass and/or defect. Initial symptoms may be minimal.
MR Appearance
Tibialis anterior tendon injuries are best seen on oblique axial images oriented perpendicular to the tendon distal to the level of the medial malleolus.65,66
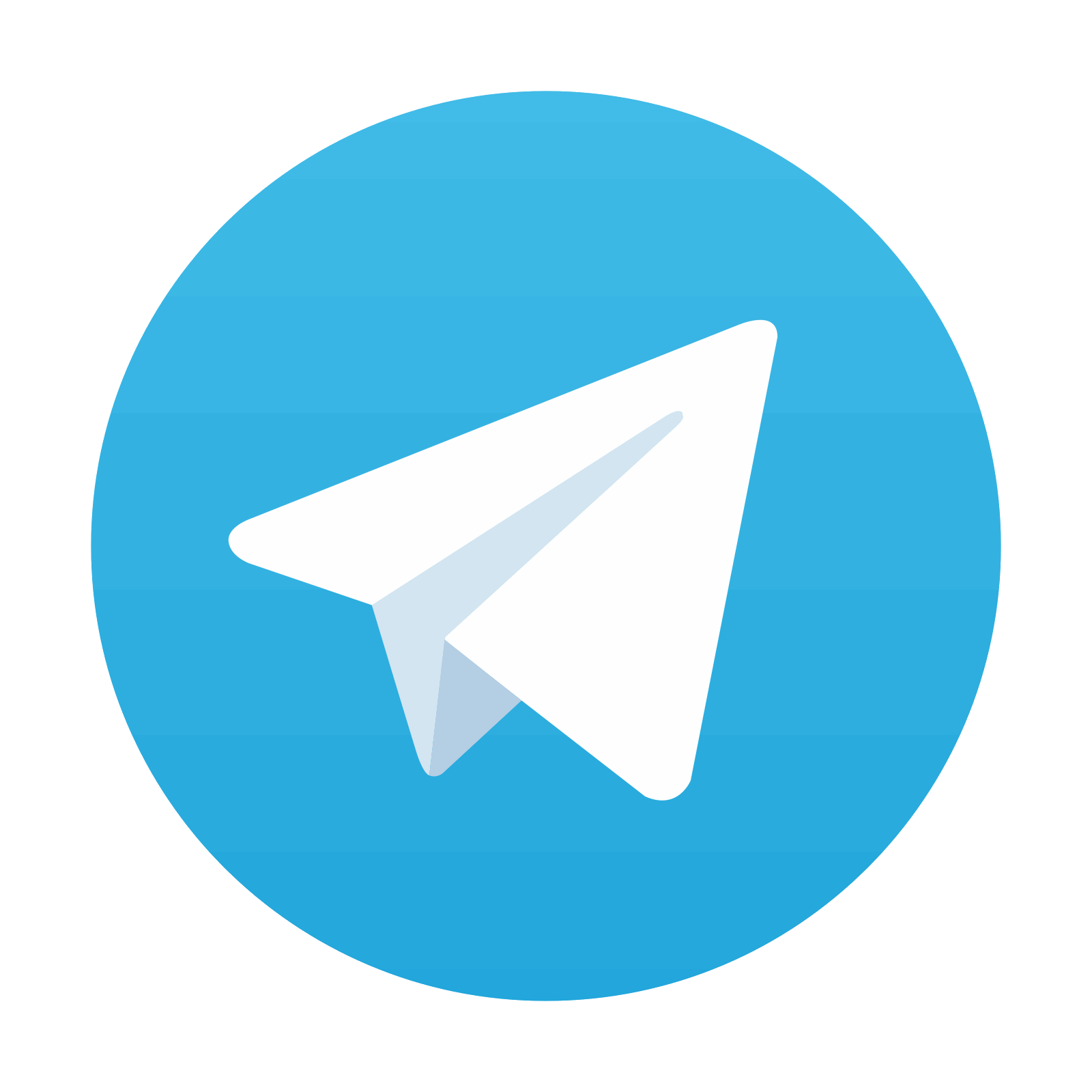
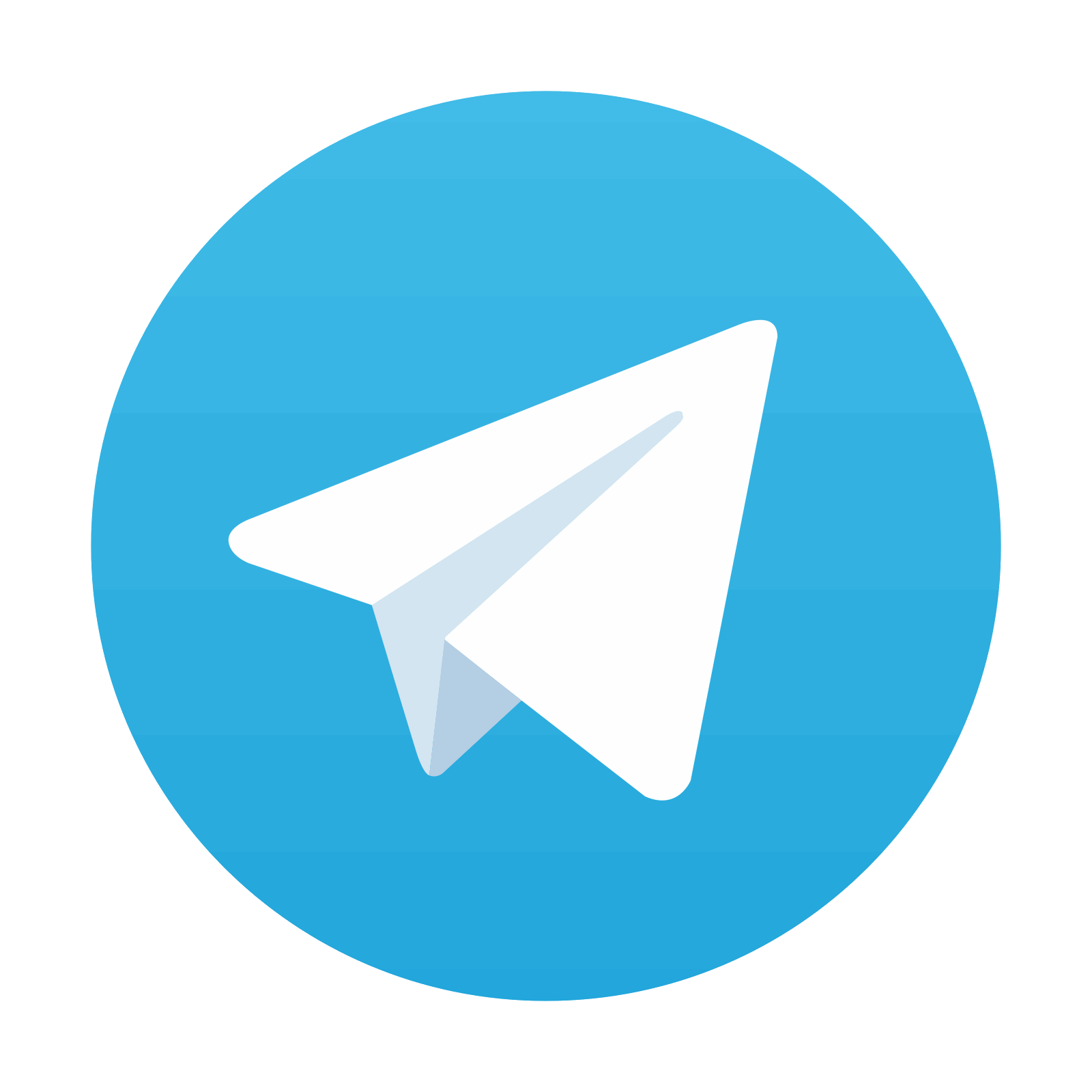
Stay updated, free articles. Join our Telegram channel

Full access? Get Clinical Tree
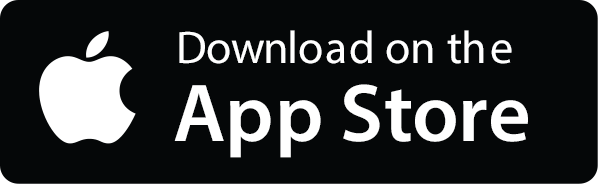
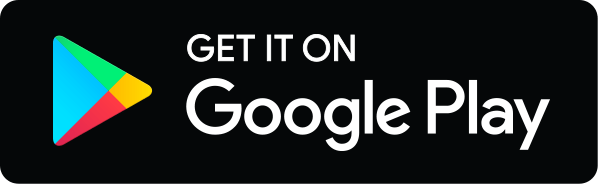
